
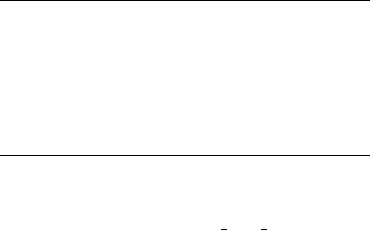
16. Heterogeneous catalytic hydrogenation |
863 |
TABLE 12. Catalytic activity and selectivity of amorphous and crystalline alloys in the hydrogenation of 1-hexene (0.4 g alloy, 5 ml 1-hexene, 5 ml 1,4-dioxane, 323 K)
Catalysts |
Activitya |
Selectivityb |
Hydrogen pressure |
|||
|
A |
|
C |
|
(105 Pa) |
|
Ni30Zr70 |
0.33 |
|
|
c |
57 |
7 |
|
|
c |
||||
Fe20Ni60B20 |
0.31 |
|
|
85 |
12 |
|
|
|
c |
||||
Fe20Ni60P20 |
0.34 |
|
|
93 |
12.5 |
|
|
|
|
||||
Fe18Ni54B16Pd12 |
0.77 |
0.56 |
58 |
12 |
||
Cu30Zr70 |
0.42 |
0.34 |
55 |
15 |
||
Pd80Si20 |
1.7 |
1.2 |
54 |
14 |
||
Pd35Zr65 |
1.0 |
0.48 |
49 |
7 |
||
Ni foil |
0.38 |
|
|
c |
65 |
14 |
|
|
c |
||||
Pd foil |
1.1 |
|
|
57 |
14 |
|
|
|
|
a Reciprocal log time (min) required for 10% conversion. A denotes amorphous, C denotes crystalline.
b Percentage of n-hexane at 10% conversion. c Too low for accurate comparisons.
considerably less active than Pd-based alloys (Pd Si, Pd Zr) and slightly less active than Ni foil (Table 12). Ni alloys, however, exhibited better selectivities. Two alloys, Fe20Ni60P20 and Fe20Ni60B20, showed very high selectivity for hydrogenation. Addition of Pd to the latter catalyst increased the activity but resulted in a considerable drop in selectivity.
A highly dispersed Pd on ZrO2 catalyst was prepared by activating an amorphous Pd35Zr65 alloy by oxidation at 543 K. Almost complete conversion in the hydrogenation of cyclohexene (523 K, hydrogen/cyclohexene D 9) was achieved with the catalyst prepared by a 10-h oxidation207. The conversion with the as-received sample was only about 5%.
5. Surface modification
Adsorption and/or transformation of appropriately selected molecules can alter the surface characteristics of metal catalysts thereby affecting both activities and selectivities.
The poisoning of the hydrogenation of propylene and cyclohexene was caused by decomposing Et3SiH on silica-supported Ni, Rh, Pd, Pt and Cu catalysts208. Different silanes (Et3SiH, Pr2SiH2 and PenSiH3) were shown to exhibit increasing poisoning activity with increasing number of Si H bonds in the molecules in the hydrogenation and isomerization of 1-pentene209. The sensitivity of the metals to poisoning increased in the sequence Rh < Cu < Pt. Decomposition of Et3SiH resulted in a gradual loss of activity in the hydrogenation of cyclohexene over supported Pd and Pt catalysts210. Upon oxidation, however, the poisoned catalysts regained their activities after hydrogen treatment. Moreover, in some cases, activities greater than the original level were detected. These changes in activity were attributed to the formation of a new, different surface.
Sulfur poisoning affects both hydrogenation and isomerization of alkenes21. The decrease in activity of hydrogenation of 1-butene over Pd pretreated with thiophene is, however, much more pronounced than the decrease in isomerization211. Isomerization, for example, still takes place on a catalyst which is inactive in hydrogenation. Since isomerization does not consume hydrogen, this was interpreted to demonstrate that sulfur affects essentially hydrogen activation.
The effect of quinoline and CCl4 on the activity of silica-supported transition metal catalysts in the hydrogenation of 1-hexene and cyclohexene was studied212.
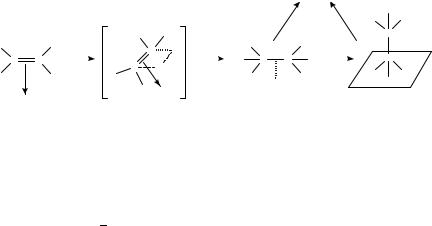
864 |
´ |
Mihaly´ Bartok´ and Arpad´ Molnar´ |
6. Hydrogenation of alkenes over metal oxides
Much less information is available on the mechanism of the hydrogenation of alkenes on oxides. Among these oxides ZnO and ZrO2 have been the most intensively studied.
Hydrogenation of alkenes on ZnO was studied by means of IR spectroscopy17. The interaction of -adsorbed ethylene and ZnH species was concluded to yield adsorbed ethyl, which reacts with ZnOH to form the product ethane. Higher alkenes adsorb as allylic species. Active sites for hydrogenation and those for exchange and isomerization are independent of ZnO. As a result, the main product in the deuteration of alkenes is the d2 isotopomer.
Different features of the hydrogenation of ethylene on ZrO2 were revealed in recent studies213. Over this oxide preadsorbed hydrogen species (ZrH and OH) are not reactive in hydrogenation but replaced by -bonded ethylene214. Hydrogen without dissociative adsorption, in turn, is believed to participate in hydrogenation. The H2 molecule is activated on (or in the vicinity of) the site where ethylene is already adsorbed. Both H atoms are instantaneously involved in the product without the participation of the ethyl species to form side-on adsorbed (20) and end-on adsorbed ethane (21) (Scheme 3).
|
|
|
|
|
|
|
|
|
DH2 CCH2 D |
|
|
|
|
|
|
|
|
|
|
|
|
|
|
|
H |
|
|
|
|
|
|
|
|
|
|
|
D |
H |
H |
H |
|
H |
|
H |
|
D |
|
C |
|||
|
|
|
|
|
H |
|
|
|
||||
|
|
D2 |
|
C D |
|
|
|
|
C |
|||
C |
C |
|
C |
|
|
|
H C C |
H |
|
|
||
|
|
|
|
|
|
|||||||
|
|
|
|
D |
|
|
|
H |
D |
|||
H |
H |
|
H |
|
|
|
D |
Zr |
H |
|||
|
|
|
|
H |
Zr |
|
|
|
H |
|||
|
|
|
|
(20) |
|
|
|
(21) |
||||
|
Zr |
|
|
|
|
|
|
|||||
|
|
|
|
|
|
|
|
|
|
SCHEME 3
The reaction of propylene on ZrO2 exhibits the same characteristics as on other oxides. Propane-d2, for example, is selectively formed in the deuteration process, with no hydrogen exchange in propylene215. New features appear, however, when zirconia is dispersed on other oxides (alumina, silica, titania)215,216. A considerable rate increase is observed and exchange in propylene proceeds simultaneously with addition via the associative mechanism through the common intermediate n-propyl and s-propyl species.
The activity of Mo Al2O3 in alkene hydrogenation has been recently correlated with the oxidation state of Mo217. A sharp increase in activity of propylene hydrogenation (347 K, flow microreactor) was observed and an increased abundance of Mo3C species was detected when the catalyst was reduced at temperatures higher than 573 K. Reduction at even higher temperatures (at and above 973 K), in turn, resulted in the formation of significant amounts of Mo metal and hydrogenolysis became the predominant reaction.
Perovskite oxides, though they characteristically promote hydrogenolysis, were demonstrated to exhibit activity in the hydrogenation of simple alkenes218. Exclusive hydrogen addition to ethylene occurs over LaCoO3 below 420 K219. Increasing temperature, however, brings about sharply increasing activity of hydrogenolysis of the product saturated hydrocarbons.
Photocatalytic decomposition of water over various metal oxides to H2 and O2 is of current interest, representing an approach to the utilization of solar energy. This catalytic system catalyzes C C bond fission and, to a lesser extent, the hydrogenation of multiple bonds. Titania220,221 and its binary oxides222 have been studied most in the hydrogenation of simple alkenes (ethylene, propylene and butenes). This photohydrogenation activity is,
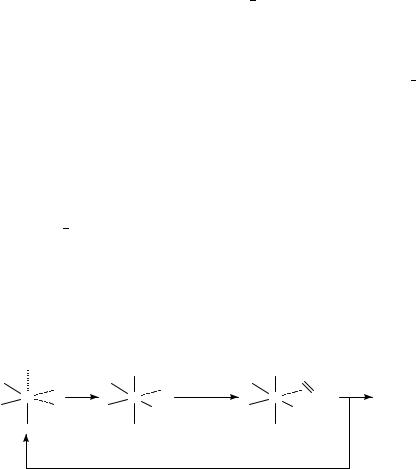
16. Heterogeneous catalytic hydrogenation |
865 |
however, enhanced significantly by the addition of small amounts of metals (Pt, Pd, Ni and Cu)223,224. The highest activity and selectivity were achieved by loading Pt and Cu in an optimum ratio into titania224. Increasing partial pressure of water led to further increase in the yield of ethane in the photohydrogenation of ethylene223.
7. Alkene hydrogenation as probe reaction
Practical heterogeneous metal catalysts (metals dispersed on inert supports) possess different types of surface atoms exhibiting different adsorption and reaction characteristics. These atoms (sites) are thought to catalyze various processes (exchange reaction, isomerizations, both double bond migration and cis trans isomerization, hydrogen shift and hydrogen addition). Different sites may also catalyze the same reaction with different activation energies, i.e. different reaction rates are expected. Attempts have been made to find correlation between the various surface sites (atoms) and the reactions they catalyze by studying the transformation of appropriately selected probe molecules under carefully controlled reaction conditions. This approach can provide direct information on the selectivity of various surface sites and has the significance to establish product site correlations.
Metal surfaces contain three principal types of sites. First Ledoux225 correlated the reactions taking place with possible surface sites. Then Siegel226 suggested to relate these sites to organometallic coordination sites with different degrees of coordinative unsaturation. 3M sites with three coordinative unsaturations are analogous to corner (kink) atoms of metal surfaces. 2M sites (two coordinative unsaturations) and 1M sites (one coordinative unsaturation), in turn, correspond to edge (step) and face (terrace) atoms, respectively.
Augustine developed a technique to determine the relative number of these various active sites present on supported metal catalysts. The method has been applied to characterize Pt174,227 229, Rh230 and Pd151,230 catalysts. During an STO olefin hydrogenation process each site reacts only once per turnover sequence. The product composition, therefore, could be related directly to the densities of the various types of sites present. In an STO sequence measurement, the catalyst is first exposed to hydrogen to achieve complete coverage followed by the injection of 1-butene. Product composition (alkane, initial and isomerized alkenes) is determined and then a portion of the alkene which remained on the catalyst is removed by a second hydrogen pulse as alkane. The amount of alkane formed originally is related to the number of 3M sites present229 catalyzing hydrogen addition according to equation 19. These sites later were directly correlated with corner atoms231.
|
|
H |
|
H |
CH2 |
|
|
|
|
|
=CHR |
|
|
M |
H2 |
M |
CH2 |
CHR |
CH3 CH2 R |
|
|
|
M |
||||
|
|
H |
|
|
H |
(19) |
3 M |
|
|
|
|
|
The amount of alkane formed from the second hydrogen pulse determines the number of 3MH sites228,229,231. Since these sites catalyze hydrogen addition in a two-step process (Scheme 4) involving a metalalkyl intermediate (22), they are also called two-step hydrogenation sites or metalalkyl sites. From the degree of isomerization the number of 2M sites (edge atoms) present on the catalyst surface can be calculated, although isomerization can
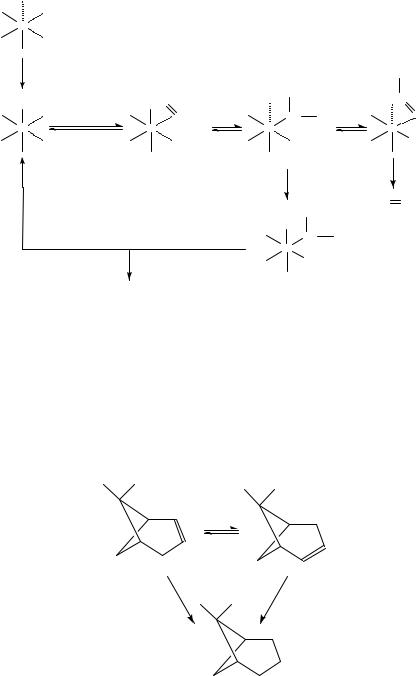
866 |
|
´ |
|
|
Mihaly´ Bartok´ and Arpad´ Molnar´ |
|
|
||
M |
|
|
|
|
3 M |
|
|
|
|
H |
|
|
|
CH3 |
|
H CH2 |
CH3 |
|
CH |
H |
|
|
||
|
|
|
||
CH2 =CHCH2 R |
CHCH2 R |
CH |
CH2 R |
CHR |
M |
M |
M |
|
M |
3 MH |
|
|
|
H |
|
(22) |
|
|
|
|
|
|
|
|
|
|
H2 |
|
|
|
|
|
CH3 CH2 |
CHR |
|
|
CH3 |
|
|
|
|
H |
|
|
|
|
CH |
CH2 R |
|
|
|
M |
|
|
|
|
H |
|
|
CH3 CH2 CH2 R
SCHEME 4
also occur on 3MH sites (Scheme 4). Surface sites of supported Pd catalysts characterized by the same technique were shown to exhibit different reactivities than those of Pt151.
The STO method was used to characterize Pt-CPG catalysts with different metal loadings and reduction temperatures to determine the most useful preparation conditions for maximum hydrogenation activity232 and explore the effects of various heat treatments for the reactivation of deactivated samples233.
Another approach in surface characterization of metal catalysts by alkene transformations is the use of (C)-apopinene (23) as the probe molecule194,196. Due to its peculiar geometry this molecule can expose only one face of its double bond to a catalyst surface.
|
ki |
|
|
ki |
|
(23) |
|
|
H2 |
H2 |
ka |
ka |
|
SCHEME 5
16. Heterogeneous catalytic hydrogenation |
867 |
Therefore, double bond migration leads to its enantiomer, which has reactivities identical to the starting enantiomer for a symmetrical surface (Scheme 5).
This technique was used to characterize supported Pt234 and Pd235 catalysts by measuring the extent of isomerization (by determining the change in optical rotation during hydrogenation) and the degree of saturation. These data allow one to calculate the ratio of isomerization to addition, ki/ka, which was found to go through a maximum at about 60% dispersion (percentage exposed metal atoms). This maximum occurs approximately at the maximum in the number of edge sites on fcc octahedral crystallites. These sites are identified as 2M sites catalyzing isomerization, whereas 3M sites can catalyze both addition and isomerization.
Alkene hydrogenation was also suggested to test for mass transfer effects during liquidphase hydrogenations236,237. The method is based on the linear poisoning of hydrogen addition to alkenes (cyclohexene and apopinene) by CS2. When the active sites of Pd or Pt catalysts are titrated with CS2 the decrease in rate is linear unless mass transfer limitations occur.
B. Hydrogenation of Dienes and Polyenes
Selective hydrogenation of a diene means the saturation of one of the double bonds to form a monoene. Such regioselective saturation, called semihydrogenation, is essentially governed by the same effects that determine the relative reactivities of monoalkenes during competitive hydrogenation in binary mixtures: a terminal double bond exhibits higher reactivity than other, more substituted double bonds and, therefore, it is saturated preferentially (see Section IV.A.1). A different kind of selectivity also arises when a diene is hydrogenated, since the newly formed monoene and the unreacted diene compete for the same active site.
1. Conjugated dienes
The reactivity of conjugated dienes, in general, exceeds that of monoenes and even nonconjugated dienes. This is attributed to the fact that in these compounds the entire system is involved in adsorption through di- -coordination which is more favored than the dimode of adsorption of a single double bond238. Due to its practical importance (see Section IV.C) and theoretical significance the semihydrogenation of 1,3-butadiene has been studied extensively and data are also available for isoprene and 1,3-pentadienes.
a. Open-chain dienes. Monohydrogenation of 1,3-butadiene can take place by either 1,2 or 1,4 addition to produce 1-butene or 2-butenes, respectively. It was established that on palladium 1-butene and trans-2-butene are the main products with only a small amount of cis-2-butene239. Other Group VIII metals240,241, and Cu240 and Au242, catalyze mainly 1-butene formation and the isomeric 2-butenes are formed in roughly equal amounts. These metals are classified as Type A catalysts (dominant 1-butene formation, near-unity trans: cis ratio), whereas Pd (as well as Fe) is a Type B metal (1-butene and trans-2-butene are the main products). Rare-earth metal clusters (Sm and Yb) studied recently yield 2- butenes in high amounts161. Catalyst pretreatment and the form of catalyst (supported or bulk metal), however, can markedly affect selectivity240.
The two types of addition were proposed to occur through different surface absorbed forms240,243 (Scheme 6). The 24 -adsorbed intermediate is suggested to result in 1,2 addition (Type A metals). The 25 and 26 -allyl species, in contrast, ensure 1,4 addition. The selectivity of the formation of stereoisomeric 2-butenes, in turn, depends on the interconversion of the possible half hydrogenated syn and anti surface -allyl complexes
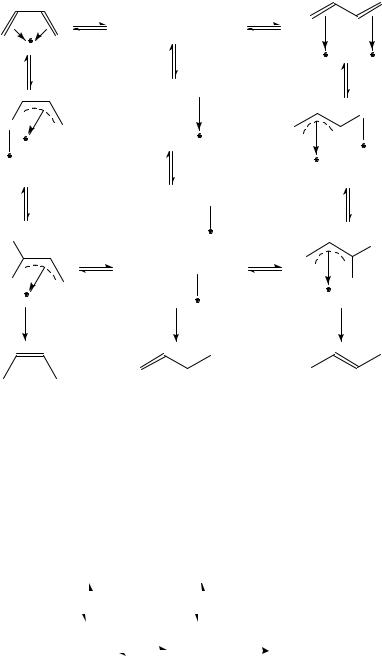
868 |
´ |
Mihaly´ Bartok´ and Arpad´ Molnar´ |
H2 C
(25)
H
H
syn
(27)
Me
H
CH2 CHCH
CH2
CH2 CHCH
CH2
(24)
H
CH2 CHCH2 CH2
+
CH2 CHCHCH3
H
SCHEME 6
CH2
(26)
H
Me
anti
(28)
H
H
(27 and 28)243. Since the s-trans conformer of 1,3-butadiene is much more stable than the s-cis conformer, the high trans/cis ratio over Pd indicates that the interconversion of the adsorbed -allyl complexes is not significant.
A general scheme of hydrogenation of 1,3-butadiene, the ‘rake’ mechanism, is given in Scheme 7. Two kinds of selectivity for the intermediate butenes can be defined. Selective formation of butenes can be expected if k2 > k4 (mechanistic selectivity). On the other hand, if k1/k 1 > k3/kŁ 3, adsorption of the diene prevents the readsorption of butenes and hence the consecutive hydrogen addition cannot take place (thermodynamic
C4 H6 (g ) |
C4 H8 (g ) |
|
|
||||||
k1 |
|
k−1 |
k3 |
|
|
|
|||
|
|
k−3 |
|
|
|||||
C4 H6 (a) |
H2 , k2 |
C4 H8 (a) |
H2 |
, k4 |
C4 H10 (g ) |
||||
|
|
|
|||||||
|
|
k−2
SCHEME 7
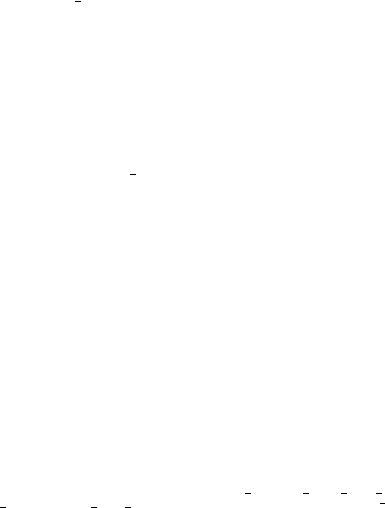
16. Heterogeneous catalytic hydrogenation |
869 |
selectivity). All experimental observations indicate that Pd exhibits the highest selectivity for semihydrogenation. Over Pd the rates of saturation of the first double bond and the consecutive hydrogenation of the product butenes have the same order of magnitude. Selectivity, therefore, is attributed to the stronger adsorption of the diene compared to that of the monoene formed97,238,244,245.
Hydrogenation of 1,3-butadiene exhibits antipathetic structure sensitivity, i.e. turnover
numbers decrease with increasing dispersion246. This was observed on Pd247,248, Pt249 and Rh250, and suggested not to be an intrinsic property of these metals251. Overcomplexation (autoinhibition), i.e. too strong interaction of the diene with the catalytic site at high dispersion, was invoked to explain this behavior. Electron-donating additives like piperidine are able to increase the activity by modifying the adsorption properties through electron donation251 253. Mechanistic selectivity was assumed to be dependent on the ability of metals to form carbene species251. Since carbenes are directly transformed to butane, carbene-forming metals (Pt and Rh) are characterized by low selectivity for semihydrogenation.
Under practical hydrogenation conditions the selectivity of semihydrogenation decreases with increasing conversion even on Pd. Catalyst modification, however, was found to
result in significant improvements. Bimetallic Pd catalysts containing Cu254, Ag255,256, Sn257, Pb257,258, Sb257, Cr259, Co260 and Ni261 as the second metal, proved to be especially selective. The general observation is that addition of the second metal leads to a decreased activity but the selectivity increases. The improved selectivity is suggested to be due to the modification of the electronic structure of Pd257,259,261,262. This, in turn, results in a change in the relative adsorption strength of the diene and intermediate alkene. When added to Pd Al2O3, a similar effect was attributed to ceria262. The beneficial role of Cu is, however, accounted for in a different way. In this case, the concentration of available surface hydrogen decreases due to the poor ability of Cu to adsorb or absorb hydrogen254. The further hydrogenation of monoenes, therefore, is severely retarded.
Bicomponent amorphous alloys also exhibit high selectivity15,16. A rapidly quenched Cu70Zr30 alloy after activation (annealed in hydrogen at 473 K for 16 h) showed an activity one order of magnitude higher than that of copper foil and 100% selectivity during the first stage of reaction263,264. The product distribution resembled that of Type A catalysts. This catalyst exhibited excellent characteristics to remove 1,3-butadiene from industrial olefin feedstocks265. At 348 K, for example, a mixture of butenes containing 3% 1,3- butadiene could be converted to a diene-free product with only 1.63% butane. It also hydrogenates 1,3-butadiene in ethylene with a selectivity of 95% with no hydrogenation of ethylene. The Raney-type catalyst prepared by HF treatment of a pulverized Cu62Zr38 glassy alloy showed the same activity enhancement in the hydrogenation of isoprene as in ethylene hydrogenation198. In contrast with the above observation, a Ni63.7Zr36.3 alloy is far less active in the amorphous state than in the crystalline form, but it exhibits much
higher selectivity to butenes (97% vs 79% at 80% conversion)266.
Rapidly quenched Ni81P19 and Ni62B38 alloys200,201,267 and a Pd2Ni50Nb48 ribbon268 are also selective catalysts in the hydrogenation of conjugated dienes (1,3-butadiene and isoprene). They show the usual activity enhancement after appropriate pretreatments (dilute HNO3, then oxidation and reduction, see Section IV.A.4.c) attributed to electrondeficient Ni species.
Detailed studies have been carried out with metal boron (Ni B, Pd B, Pt B) and metal phosphorous (Ni P, Pd P) films prepared by radiofrequency sputtering269 272. Pt hardly interacts with boron and shows the low selectivity of the pure metal269. Interaction between Ni or Pd and the metalloids results in a change in the electron density of
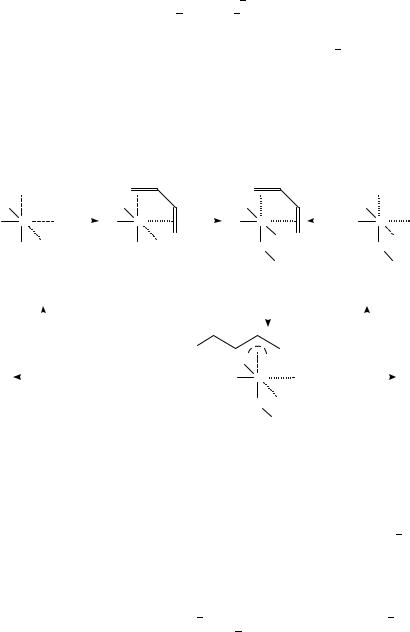
870 |
´ |
Mihaly´ Bartok´ and Arpad´ Molnar´ |
Ni and Pd, which, in turn, brings about a change in the selectivity of the hydrogenation of dienes. The best selectivities are around 98 99%. Further enhancement could be achieved by the heat treatment of Pd B and Pd P with high metalloid content271,272. This was attributed to the formation of new, highly selective phases, for example Pd3B and Pd5P2.
Conjugated dienes are hydrogenated on copper chromite273 276 and copper aluminate273. Once activated by hydrogen they are able to hydrogenate dienes even in the absence of gaseous hydrogen, i.e. they behave like a reversible hydrogen reservoir. The catalytic site involves cuprous ions in an octahedral environment and occluded hydrogen species (hydride ions) located in anionic vacancies. According to the mechanism
assumed by studying isomeric C5 dienes273,276 (Scheme |
8) |
the allyl carbanion 32 is |
||||
the major surface species. The catalytic cycle is 30 |
|
31 |
|
32 |
|
29 over the prereduced |
|
|
|
catalyst when occluded hydrogen is present. When gaseous hydrogen is used, in turn,
the 29 |
|
33 |
|
31 |
|
|
32 |
|
30 route is operative. |
|
|
|
|
|
|
|
|
|
|
|
|
|||||
|
|
|
|
|
|
|
|
|
|
|
|
|
|
|
|
|
||||||||||
O |
|
|
|
|
|
|
|
diene |
O |
H2 |
O |
|
|
O |
||||||||||||
|
|
+ |
|
|
|
|
|
+ |
|
+ |
|
diene |
+ |
|
||||||||||||
O |
Cu |
|
|
|
|
|
O |
Cu |
|
|
|
O |
Cu |
|
|
O |
|
Cu |
||||||||
|
|
|
|
|
|
|
|
|
|
|
||||||||||||||||
|
|
O |
|
|
|
|
|
|
O |
|
|
|
|
|
H |
|
|
|
H |
|||||||
|
|
|
|
|
|
|
|
|
|
|
O |
|
|
|
O |
|||||||||||
|
|
(29) |
|
|
|
|
|
|
(33) |
|
|
|
|
|
|
H |
|
|
|
H |
||||||
|
|
|
|
|
|
|
|
|
|
|
|
(31) |
|
|
|
(30) |
|
|||||||||
|
|
|
|
|
|
|
|
|
|
|
|
|
|
|
|
|
|
|
|
|
|
|
|
|
||
|
|
|
|
|
|
|
|
|
|
|
|
|
|
H |
|
|
|
− |
|
|
|
|
|
|
|
|
|
|
|
|
|
|
|
|
|
|
|
|
|
|
|
|
|
|
|
|
|
|
|
|
|
||
|
|
|
|
|
|
|
|
|
|
|
|
|
|
|
|
|
|
|
|
|
|
|
||||
|
|
|
|
|
|
|
|
|
|
|
|
|
|
|
|
|
|
|
|
|
|
|
|
|
|
|
|
|
|
|
|
|
|
|
|
|
|
|
|
|
|
|
|
O |
H2 |
|
|
|
|||||
|
|
|
|
|
|
|
|
|
|
|
|
|
|
|
|
|
|
+ |
|
|
|
|
|
|
||
alkene |
|
|
|
|
|
|
|
|
|
|
|
|
|
|
|
|
O |
Cu |
|
|
|
|
alkene |
|||
|
|
|
|
|
|
|
|
|
|
|
|
|
|
|
|
|
|
|
O
H
(32)
SCHEME 8
Metal oxides (CaO, MgO, CeO2, ZrO2 and La2O3) are active and selective in the hydrogenation of conjugated dienes at ambient temperature277. The rate is about 1 or 2 orders of magnitude higher when the reaction is carried out by hydrogen transfer relative to the hydrogenation with gaseous hydrogen. In addition, the hydrogen transfer process exclusively yields monoalkenes. trans-2-Butene is formed in very high yield (90 95%) over CeO2. Exclusive 1,4 addition was proved by the transformation of 1,3-butadiene-d6.
b. Cyclic dienes. Hydrogenation of simple, nonsubstituted cyclic conjugated dienes (cyclopentadiene and 1,3-cyclooctadiene) is less complicated, since only a single cycloalkene is formed. Cyclopentene is produced in excellent yield by the selective hydrogenation of cyclopentadiene over Cu Al2O3 aerogel in the gas phase (453 493 K, 100% selectivity at 92% conversion)278. The Eley Rideal mechanism88,89 was shown to be operative with the RDS between adsorbed cyclopentadiene and gas-phase hydrogen.
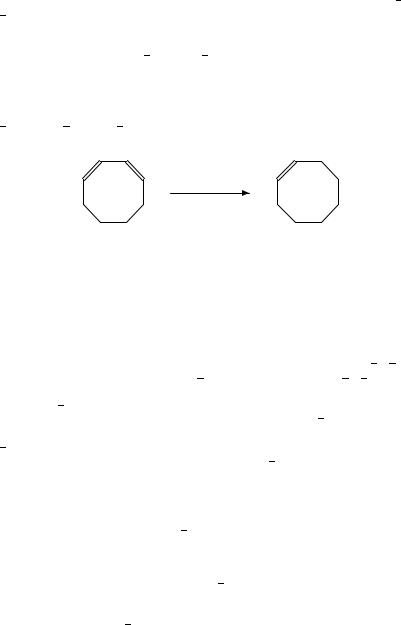
16. Heterogeneous catalytic hydrogenation |
871 |
Similar high selectivity values were reported when the reaction was carried out in the presence of colloidal Pd279,280.
The characteristics of the selective hydrogenation of cyclopentadiene over Pd P and Pd B alloys were very similar to those observed for 1,3-butadiene271,272. The selectivity increased with increasing P and B content and by the heat treatment of the alloys with higher metalloid content. Over catalysts containing more than 20% P the selectivity was 100%. Amorphous Ni B and Ni P catalysts prepared by chemical reduction proved to be more active than Raney nickel281,282. Activity was increased by the addition of Pd whereas hydrogenation in the presence of pyridine resulted in increased selectivity281.
Pd is the preferred metal for the partial hydrogenation of 1,3-cyclooctadiene. Pd CaCO3, Pd C and Pd -Al2O3 exhibit high selectivity283 (equation 20)256. Addition of Ag to Pd decreased the activity, without affecting selectivity256.
isooctane, |
|
293 K, 1 atm H2 |
(20) |
Pd−C: 99% selectivity at 99.8% conversion
Pd−γ-Al2 O3 : 99.2% selectivity at 100% conversion
Pumice-supported Pd showed similar selectivity (100% up to complete conversion) which is due to the very low hydrogenation rate of the intermediate cyclooctene284. The activity was invariant up to 35% dispersion, then decreased slowly, which is explained by the increasing electron density of Pd brought about by NaC and KC ions present in the support. The decrease in both activity and selectivity is anticipated when Pd is alloyed with Pt, a nonselective metal285. The substantial decrease in activity of Pd Pt pumice catalysts (TOF values are 90 s 1 for Pd pumice and 12 s 1 for Pd Pt pumice) was attributed to the reduced amount of Pd4 units necessary for the surface reaction and the very strong Pt diene bond.
High selectivity was also observed on a silica-supported Fe Cu catalyst prepared by coprecipitation (333 K, 10 atm H2, ethanol)286 and over polymer-protected colloidal Pd Pt cluster catalysts (303 K, 1 atm H2, ethanol)287,288. In contrast with the above observation, the activity of the bimetallic alloy was 1.4 3 times higher than that of the monometallic Pd cluster reaching the maximum activity at a composition of Pd/Pt D 4:1.
Rapid uptake of one equivalent of hydrogen was observed when 1,3-cyclooctadiene was hydrogenated over colloidal Pd supported on PVP289. The yield of cyclooctene was 99.9% (303 K, 1 atm H2). Alcohols, particularly methanol, were found to be good solvents. The activity of PVP-protected colloidal Cu Pd alloys290 reached the activity of pure Pd at a molar composition of 1:1. Both the presence of the protective polymer and the Cu/Pd ratio affect selectivity. It is assumed that PVP works as a position-blocking ligand whereas Cu acts by releasing electrons. These actions weaken the coordination of monoene to Pd but do not alter significantly the strength of diene catalyst complexation.
Siegel carried out detailed studies with di-tert-butyl-substituted cyclohexadienes with the aim of clarifying their role as intermediates in the hydrogenation of di-tert- butylbenzenes on a 5% Rh alumina catalyst291. The three isomeric dienes (34, 35, 36)
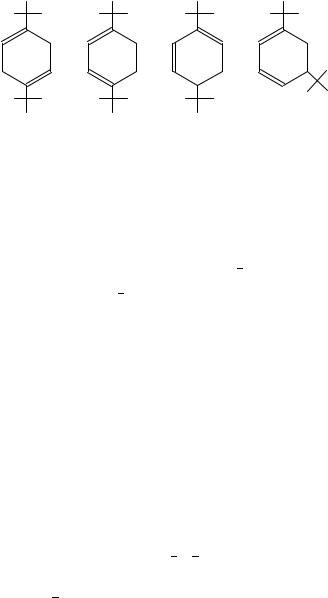
872 |
´ |
Mihaly´ Bartok´ and Arpad´ Molnar´ |
isolated in the hydrogenation of 1,4-di-tert-butylbenzene were shown to exhibit increasing reactivity (1:3:30) and increasing ability to exclude intermediate alkenes from the catalyst. Of the five isomeric dienes formed from 1,3-di-tert-butylbenzene the 1,5-di-tert-butyl-1,3- cyclohexadiene (37) interacts most strongly with the catalytic site292.
(34) |
(35) |
(36) |
(37) |
c. Sulfur poisoning. Much attention has |
been paid to |
the effect of sulfur poisoning |
on the hydrogenation of conjugated dienes, since pyrolytic gasoline streams, undergoing catalytic hydrorefining to selectively hydrogenate diolefins, always contain various sulfur compounds.
Sulfur is known to increase the selectivity of the semihydrogenation of dienes21. Improved 1,4 addition observed on Ni presulfided with H2S in the hydrogenation of 1,3-butadiene was attributed to a change in the electron density of nickel293. A similar improvement was observed on presulfided Pd211. The yield of cyclopentene was markedly increased when cyclopentadiene was hydrogenated on a Pd C catalyst partially poisoned by sulfur (97% vs 75%)294. Improved selectivity, in turn, was not always manifested on various crystallographic Pt faces295 297.
The way sulfur treatment is applied gives rise to pronounced differences in selectivities21. A decrease in the overall alkene yield was observed when 1,3-butadiene was hydrogenated on presulfided Pd. In contrast, much improvement in selective alkene formation from isoprene was achieved when sulfur was present in the feed. In the latter case adsorption competition was suggested to account for the favorable effect of sulfur.
2. Nonconjugated dienes
The problem of selective hydrogenation of one double bond of a nonconjugated diene is essentially the same as that of competitive hydrogenation of alkene mixtures (see Section IV.A.1). The less substituted double bond of the diene, consequently, can preferentially be hydrogenated under appropriate reaction conditions. The use of nonisomerizing catalysts, in general, is recommended to achieve such regioselective reaction.
Industrial processes were developed for the selective partial hydrogenation of 4- vinylcyclohexene with Ni catalysts exhibiting minimized isomerization activity in the presence of additives298,299. For example, supported nickel arsenides prepared by reducing nickel arsenate with NaBH4 display high selectivity in the formation of 4-ethylcyclohexene (96% selectivity at 96% conversion on Ni As Al2O3, 398 K, 25 atm H2, acetone additive).
Metal-assisted reductions with NaBH4 can be used to hydrogenate various functional groups41,42. The Co2C NaBH4 system selectively reduces limonene at the less substituted double bond300 though W-4 Raney Ni proved to be more effective301 (equation 21).