
Garrett R.H., Grisham C.M. - Biochemistry (1999)(2nd ed.)(en)
.pdf
19.6 ● Anaerobic Pathways for Pyruvate |
631 |
19.5 ● The Metabolic Fates of NADH and Pyruvate—
The Products of Glycolysis
In addition to ATP, the products of glycolysis are NADH and pyruvate. Their processing depends upon other cellular pathways. NADH must be recycled to NAD , lest NAD become limiting in glycolysis. NADH can be recycled by both aerobic and anaerobic paths, either of which results in further metabolism of pyruvate. What a given cell does with the pyruvate produced in glycolysis depends in part on the availability of oxygen. Under aerobic conditions, pyruvate can be sent into the citric acid cycle (also known as the tricarboxylic acid cycle; see Chapter 20), where it is oxidized to CO2 with the production of additional NADH (and FADH2). Under aerobic conditions, the NADH produced in glycolysis and the citric acid cycle is reoxidized to NAD in the mitochondrial electron transport chain (Chapter 21).
19.6 ● Anaerobic Pathways for Pyruvate
Under anaerobic conditions, the pyruvate produced in glycolysis is processed differently. In yeast, it is reduced to ethanol; in other microorganisms and in animals, it is reduced to lactate. These processes are examples of fermentation —the production of ATP energy by reaction pathways in which organic molecules function as donors and acceptors of electrons. In either case, reduction of pyruvate provides a means of reoxidizing the NADH produced in the glyc- eraldehyde-3-phosphate dehydrogenase reaction of glycolysis (Figure 19.30). In yeast, alcoholic fermentation is a two-step process. Pyruvate is decarboxylated to acetaldehyde by pyruvate decarboxylase in an essentially irreversible reaction. Thiamine pyrophosphate is a required cofactor for this enzyme. The second step, the reduction of acetaldehyde to ethanol by NADH, is catalyzed
(a) Alcoholic fermentation |
|
O |
|
|
|
|
|
|
|
|
|
(b) Lactic acid fermentation |
|
O |
|
|
|
|
|
|
|
|
|
|||||||||||||||||||
|
|
|
|
|
|
|
|
|
|
|
|
|
|
|
|
|
|
|
|
|
|
|
|
|
|
|
|
|
|
|
|
|
|
|
|
|
|
|
||||
|
|
CHO |
|
HPO24– |
|
|
|
C OPO23– |
|
|
|
|
|
|
|
|
|
CHO |
HPO24– |
|
|
|
|
|
|
|
C OPO23– |
|||||||||||||||
|
|
|
|
|
|
|
|
|
|
|
|
|
|
|
|
|
|
|
|
|
|
|
|
|
|
|
|
|
|
|
|
|
|
|
|
|
|
|
|
|||
|
|
|
|
|
|
|
|
|
|
|
|
|
|
|
|
|
|
|
|
|
|
|
|
|
|
|
|
|
|
|
|
|
|
|
|
|
|
|
|
|
|
|
H |
|
C |
|
OH |
|
|
|
H |
|
C |
|
OH |
|
|
|
|
|
H |
|
|
|
C |
|
|
OH |
|
|
H |
|
|
|
|
|
C |
|
|
OH |
|||||
|
|
|
G3PDH |
|
|
|
|
|
|
|
|
|
|
|
|
|
G3PDH |
|
|
|
|
|
|
|
|
|||||||||||||||||
|
|
|
|
|
|
|
|
|
|
|
|
|
|
|
|
|
|
|
|
|
|
|
|
|
|
|
|
|
|
|
|
|
|
|
|
|
|
|
|
|
||
|
|
CH2OPO23– |
|
|
|
|
CH2OPO23– |
|
|
|
|
|
|
|
|
CH2OPO23– |
|
|
|
|
|
|
|
|
CH2OPO23– |
|||||||||||||||||
D-Glyceraldehyde- |
|
|
|
|
1,3-BPG |
|
|
|
|
|
D-Glyceraldehyde- |
|
|
|
|
|
|
|
|
1,3-BPG |
||||||||||||||||||||||
3-phosphate |
|
|
|
|
|
|
|
|
|
|
|
|
|
|
3-phosphate |
|
|
|
|
|
|
|
|
|
|
|
|
|
||||||||||||||
|
|
|
|
|
|
|
NAD+ |
NADH + H+ |
|
|
|
|
|
|
|
|
|
|
|
|
|
|
|
NAD+ |
NADH + H+ |
|
|
|
|
|
||||||||||||
|
|
|
|
|
|
|
|
|
|
|
|
|
|
|
O |
|
|
|
|
|
|
|
|
|
|
|
|
|
|
|
|
|
|
|
|
|
|
|
||||
|
|
|
|
|
|
|
|
|
|
|
|
|
|
|
|
|
|
|
COO– |
|
|
|
|
|
|
|
|
|
|
|
|
|
|
|
|
|
|
|
|
|
|
|
|
|
|
|
|
|
|
|
|
|
|
|
|
|
|
CH3C |
|
|
|
|
|
|
|
|
|
|
|
|
|
|
|
|
|
|
|
|
|
|
|
|
|||
|
|
|
|
|
|
|
|
|
|
|
|
|
|
|
|
|
|
|
|
|
|
|
|
|
|
|
|
|
|
|
|
|
|
|
|
|
|
|
||||
|
|
|
|
|
|
|
|
|
|
|
|
|
|
|
Pyruvate |
|
|
|
|
|
OH |
|
|
|
O |
|
|
|
|
|
||||||||||||
|
|
|
|
|
|
|
|
|
|
|
|
|
|
|
|
|
|
|
|
|
|
|
|
|
|
|
|
|
|
|
|
|
||||||||||
|
|
|
|
|
|
|
|
|
|
|
|
|
|
|
|
|
|
|
|
|
|
|
|
|
|
|
|
|
COO– |
|
|
|
|
|
|
|
|
|
|
|
COO– |
|
|
|
CH |
CH |
OH |
|
|
CH |
CHO |
CO |
|
|
|
|
CH |
3 |
|
|
C |
|
|
|
CH |
C |
|
|
|
||||||||||||||||
|
|
|
|
|
|
|
|
|
|
|
|
|
|
|
|
|
||||||||||||||||||||||||||
3 |
2 |
|
Alcohol |
|
3 |
|
|
|
|
2 |
|
|
|
|
|
|
|
|
|
|
Lactate |
3 |
|
|
|
|
|
|
|
|
|
|||||||||||
|
|
|
Ethanol |
|
Acetaldehyde |
|
|
|
|
|
|
|
|
|
|
|
|
|
|
|
Pyruvate |
|||||||||||||||||||||
|
|
|
dehydrogenase |
|
|
|
|
|
|
|
|
|
|
H |
|
|
dehydrogenase |
|||||||||||||||||||||||||
|
|
|
|
|
|
|
|
|
|
|
|
|
|
|
|
|
|
|
|
|
|
|
|
|
|
|
|
|
|
|
|
|
|
|
|
Lactate
● (a) Pyruvate reduction to ethanol in yeast provides a means for regenerating NAD consumed in the glyceraldehyde-3-P dehydrogenase reaction. (b) In oxy- gen-depleted muscle, NAD is regenerated in the lactate dehydrogenase reaction.
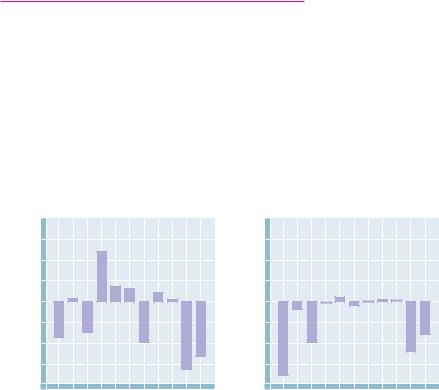
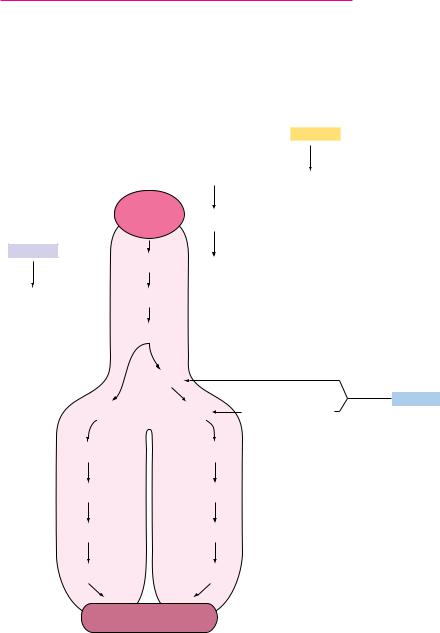
634 Chapter 19 ● Glycolysis
duced by breakdown of sucrose, may participate in glycolysis by at least two different routes. In the liver, fructose is phosphorylated at C-1 by the enzyme fructokinase:
D-Fructose ATP4 88n D-fructose-1-phosphate2 ADP3 H (19.10)
Subsequent action by fructose-1-phosphate aldolase cleaves fructose-1-P in a manner like the fructose bisphosphate aldolase reaction to produce dihydroxyacetone phosphate and D-glyceraldehyde:
D-Fructose-1-P2 88n D-glyceraldehyde dihydroxyacetone phosphate2
(19.11)
Dihydroxyacetone phosphate is of course an intermediate in glycolysis. D-Gly- ceraldehyde can be phosphorylated by triose kinase in the presence of ATP to form D-glyceraldehyde-3-phosphate, another glycolytic intermediate.
In the kidney and in muscle tissues, fructose is readily phosphorylated by hexokinase, which, as pointed out above, can utilize several different hexose substrates. The free energy of hydrolysis of ATP drives the reaction forward:
D-Fructose ATP4 88n D-fructose-6-phosphate2 ADP3 H (19.12)
Fructose-6-phosphate generated in this way enters the glycolytic pathway directly in step 3, the second priming reaction. This is the principal means for channeling fructose into glycolysis in adipose tissue, which contains high levels of fructose.
The Entry of Mannose into Glycolysis
Another simple sugar that enters glycolysis at the same point as fructose is mannose, which occurs in many glycoproteins, glycolipids, and polysaccharides (Chapter 7). Mannose is also phosphorylated from ATP by hexokinase, and the mannose-6-phosphate thus produced is converted to fructose-6-phosphate by phosphomannoisomerase.
D-Mannose ATP4 88n D-mannose-6-phosphate2 ADP3 H |
(19.13) |
D-Mannose-6-phosphate2 88n D-fructose-6-phosphate2 |
(19.14) |
The Special Case of Galactose
A somewhat more complicated route into glycolysis is followed by galactose, another simple hexose sugar. The process, called the Leloir pathway after Luis Leloir, its discoverer, begins with phosphorylation from ATP at the C-1 position by galactokinase:
D-Galactose ATP4 88n D-galactose-1-phosphate2 ADP3 H (19.15)
Galactose-1-phosphate is then converted into UDP-galactose (a sugar nucleotide) by galactose-1-phosphate uridylyltransferase (Figure 19.33), with concurrent production of glucose-1-phosphate and consumption of a molecule of UDPglucose. The uridylyltransferase reaction proceeds via a “ping-pong” mechanism (Figure 19.34) with a covalent enzyme-UMP intermediate. The glucose- 1-phosphate produced by the transferase reaction is a substrate for the phosphoglucomutase reaction (Figure 19.33), which produces glucose-6-phos- phate, a glycolytic substrate. The other transferase product, UDP-galactose, is converted to UDP-glucose by UDP-glucose-4-epimerase. The combined action of the uridylyltransferase and epimerase thus produces glucose-1-P from galac- tose-1-P, with regeneration of UDP-glucose.
A rare hereditary condition known as galactosemia involves defects in galac- tose-1-P uridylyltransferase that render the enzyme inactive. Toxic levels of
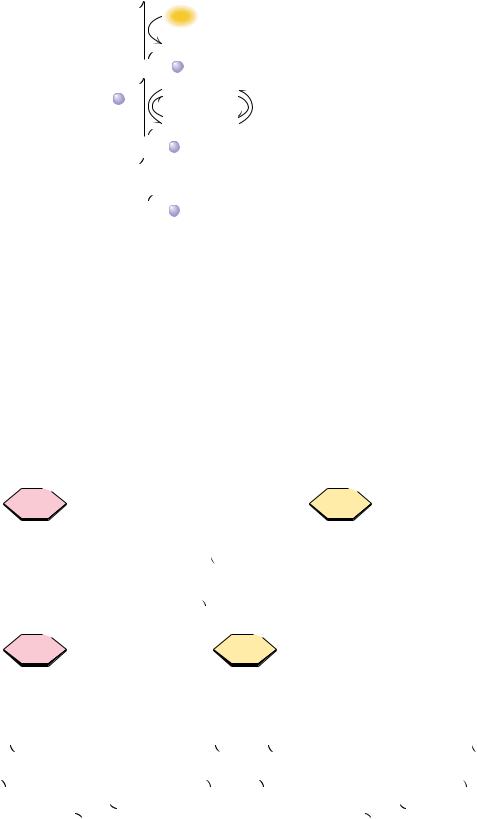
19.8 ● Utilization of Other Substrates in Glycolysis |
635 |
Galactose |
|
|||
|
|
|
ATP |
|
Galactokinase |
|
ADP |
|
|
|
|
|
|
|
|
|
|
|
|
Galactose-1- P |
|
|||
Galactose-1- P |
|
UDP-Glucose |
UDP-Galactose- |
|
|
||||
|
|
|||
uridylyltransferase |
|
|
4-epimerase |
|
|
|
|
UDP-Galactose |
|
|
|
|
|
|
Glucose-1- P |
|
|||
|
|
|
Phosphoglucomutase |
|
|
|
|
|
|
|
|
|
|
|
Glucose-6- P
FIGURE 19.33 ● Galactose metabolism via the Leloir pathway.
galactose accumulate in afflicted individuals, causing cataracts and permanent neurological disorders. These problems can be prevented by removing galactose and lactose from the diet. In adults, the toxicity of galactose appears to be less severe, due in part to the metabolism of galactose-1-P by UDP-glucose pyrophosphorylase, which apparently can accept galactose-1-P in place of glucose-1-P (Figure 19.35). The levels of this enzyme may increase in galactosemic individuals, in order to accommodate the metabolism of galactose.
|
|
|
CH2OH |
|
|
|
|
|
|
|
|
|
|
|
|
|
|
|
|
|
|
|
|
|
|
|
|
|
|
|
|
|
|
CH2OH |
|
|
|
|
|
|
|
|
|
|
|||||||||||||
|
|
|
|
|
O |
|
|
O |
|
|
|
O– |
|
|
|
|
|
|
|
|
|
|
|
|
|
|
|
HO |
|
|
|
O |
|
|
O |
|
|||||||||||||||||||||
|
|
|
OH |
|
|
|
|
|
|
|
|
|
|
|
|
|
|
|
|
|
|
|
|
|
|
+ |
|
|
|
|
|
|
|
|
|
|
OH |
|
|
|
|
|
|
|
O– |
|
|||||||||||
|
|
|
|
|
|
|
|
|
|
|
|
|
|
|
|
|
|
|
|
|
|
|
|
|
|
|
|
|
|
|
|
|
|
|
|
|
|
|
|
|
|
|
|
|
|
|
|
P |
|
|
|
||||||
HO |
|
|
|
O |
|
|
P |
|
O |
|
|
P |
|
O |
|
Uridine |
|
|
|
|
|
|
|
|
|
H |
|
|
|
|
|
|
O |
|
|
|
|
|
|||||||||||||||||||
|
|
|
|
|
|
|
|
|
|
|
|
|
|
|
|
|
|||||||||||||||||||||||||||||||||||||||||
|
|
|
|
|
|
|
|
|
|
|
|
|
|
|
|
|
|
|
|
|
|
|
|
|
|
|
|
|
|
|
|
|
|
|
|
|
|
|
|
|
|
|
|
|
|
|
|
|
|
|
|
|
|
|
|
|
|
|
|
|
H |
OH |
|
|
|
|
|
|
|
|
|
|
|
|
|
|
|
|
|
|
|
|
|
|
|
|
|
|
|
|
|
|
H |
|
OH |
|
|
|
|
|
|
|
|
|
|
||||||||||
|
|
|
|
|
O– |
|
|
|
O |
|
|
|
|
|
|
|
|
|
|
|
|
|
|
|
|
|
|
|
|
|
O– |
|
|||||||||||||||||||||||||
|
|
|
|
|
|
|
|
|
|
|
|
|
|
|
|
|
|
|
|
|
|
|
|
|
|
|
|
|
|
|
|
|
|
|
|
|
|
|
|
|
|
|
|
|
|||||||||||||
|
|
|
CH2OH |
UDP-glucose |
|
|
|
|
|
CH2OH |
|
|
|
|
|
|
|
α -D-Galactose-1-P |
|
||||||||||||||||||||||||||||||||||||||
|
|
|
|
|
|
|
|
|
|
|
|
|
|
|
|
||||||||||||||||||||||||||||||||||||||||||
|
|
|
|
|
|
|
|
|
|
|
|
|
|
|
|
|
|
|
|
|
|
|
|
|
|
|
|
|
|
|
|
|
|
|
|
|
|
|
|
|
|
|
|
|
|
|
|||||||||||
|
|
|
|
|
|
|
|
|
|
|
|
|
|
|
|
|
|
|
|
|
|
|
|
|
|
|
|
|
|
|
|
|
|
|
|
|
|
|
|
|
|
|
|
|
|
|
|||||||||||
|
|
|
|
|
O |
|
|
O |
|
|
|
|
|
|
|
HO |
|
|
O |
|
|
|
O |
|
|
|
O– |
|
|
|
|
|
|
|
|
|
|
|
|||||||||||||||||||
HO |
|
|
OH |
|
|
|
|
|
|
|
|
O– |
+ |
|
|
|
|
|
OH |
|
|
|
|
|
|
|
|
|
|
|
|
|
|
|
|
|
|
|
|
|
|
|
|
|
|
|
|
|
|
|
|||||||
|
|
|
|
|
O |
|
|
P |
|
|
|
|
|
|
|
|
|
|
|
|
O |
|
P |
|
|
O |
|
P |
|
|
O |
|
|
Uridine |
|
|
|
||||||||||||||||||||
|
|
|
|
|
|
|
|
|
|
|
|
|
|
|
|
|
|
|
|
|
|
|
|
|
|
|
|
|
|||||||||||||||||||||||||||||
|
|
|
|
|
|
|
|
|
|
|
|
|
|
|
|
|
|
|
|
|
|
|
|
|
|
|
|
|
|
|
|
|
|
|
|
|
|
|
|
|
|
|
|
|
|
|
|
|
|
|
|
|
|
|
|||
|
|
|
H |
OH |
|
|
|
|
|
|
|
|
|
|
|
|
|
|
|
|
|
H |
OH |
|
|
|
|
|
|
|
|
|
|
|
|
|
|
|
|
|
|
|
|
|
|
|
|
|
|
|
|||||||
|
|
|
|
|
O– |
|
|
|
|
|
|
|
|
|
|
|
|
|
|
|
O– |
|
|
|
O |
|
|
|
|
|
|
|
|
|
|
|
|||||||||||||||||||||
|
|
|
|
|
|
|
|
|
|
|
|
|
|
|
|
|
|
|
|
|
|
|
|
|
|
|
|
|
|
|
|
|
|
|
|
|
|
|
|
|
|
|
|
|
|||||||||||||
|
|
|
α -D-Glucose-1-P |
|
|
|
|
|
|
|
|
|
|
|
|
|
|
|
|
|
|
|
UDP-galactose |
|
|
|
|
|
|
|
|
|
|
||||||||||||||||||||||||
UDPGlc |
|
|
|
|
|
|
|
|
|
|
|
|
|
|
|
Glc–1–P |
Gal–1–P |
|
|
|
|
|
|
|
|
|
|
|
|
|
|
UDPGal |
|||||||||||||||||||||||||
k1 |
|
k–1 |
|
|
|
|
|
|
|
|
|
|
|
|
|
|
|
k3 |
|
|
|
k–3 |
k4 |
|
|
k–4 |
|
|
|
|
|
|
|
|
|
|
|
|
|
|
k6 |
|
k–6 |
||||||||||||||
|
|
|
|
|
|
|
|
|
|
|
|
|
|
|
|
|
|
|
|
|
|
|
|
|
|
|
|
|
|
|
|
|
|
|
|
||||||||||||||||||||||
|
|
|
|
|
|
|
|
|
|
|
|
|
|
|
|
|
|
|
|
|
|
|
|
|
|
|
|
|
|
|
|
|
|
|
|
|
|
|
|
|
|
|
|
|
|
|
|
|
|
|
|||||||
|
|
|
|
|
|
|
|
|
|
|
|
|
|
|
|
|
|
|
|
|
|
|
|
|
|
|
|
|
|
|
|
|
|
|
|
|
|
|
|
|
|
|
|
|
|
|
|
|
|
|
|||||||
|
|
|
|
|
|
|
|
|
|
|
k2 |
|
|
|
|
|
|
|
|
|
|
|
|
|
|
|
|
|
|
|
|
|
|
|
|
|
|
|
|
|
|
|
|
|
|
|
k5 |
|
|||||||||
E |
E • UDPGlc |
|
|
|
|
|
|
|
E–UMP • Glc–1–P |
E–UMP |
E–UMP • Gal–1–P |
|
|
|
|
|
|
|
|
E–UDPGal |
E |
||||||||||||||||||||||||||||||||||||
|
|
|
|
|
|
|
|
|
|
|
|
|
|
||||||||||||||||||||||||||||||||||||||||||||
|
|
|
|
|
|
|
|
|
|
|
k–2 |
|
|
|
|
|
|
|
|
|
|
|
|
|
|
|
|
|
|
|
|
|
|
|
|
|
|
|
|
|
|
|
|
|
|
|
k–5 |
|
FIGURE 19.34 ● The galactose-1-phosphate uridylyltransferase reaction involves a “pingpong” kinetic mechanism.
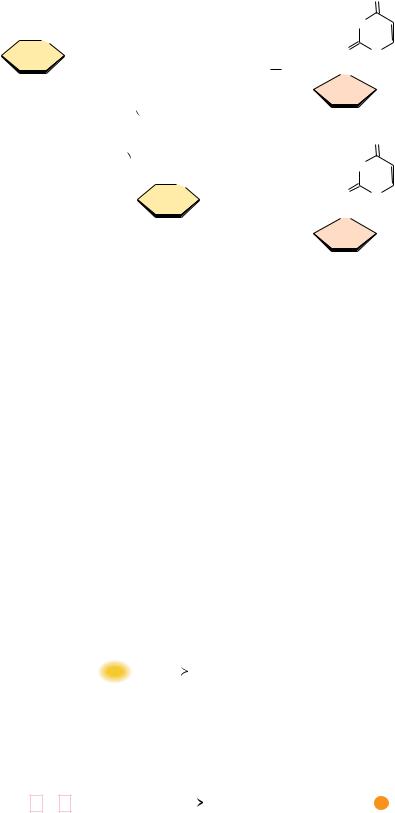
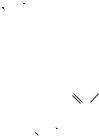
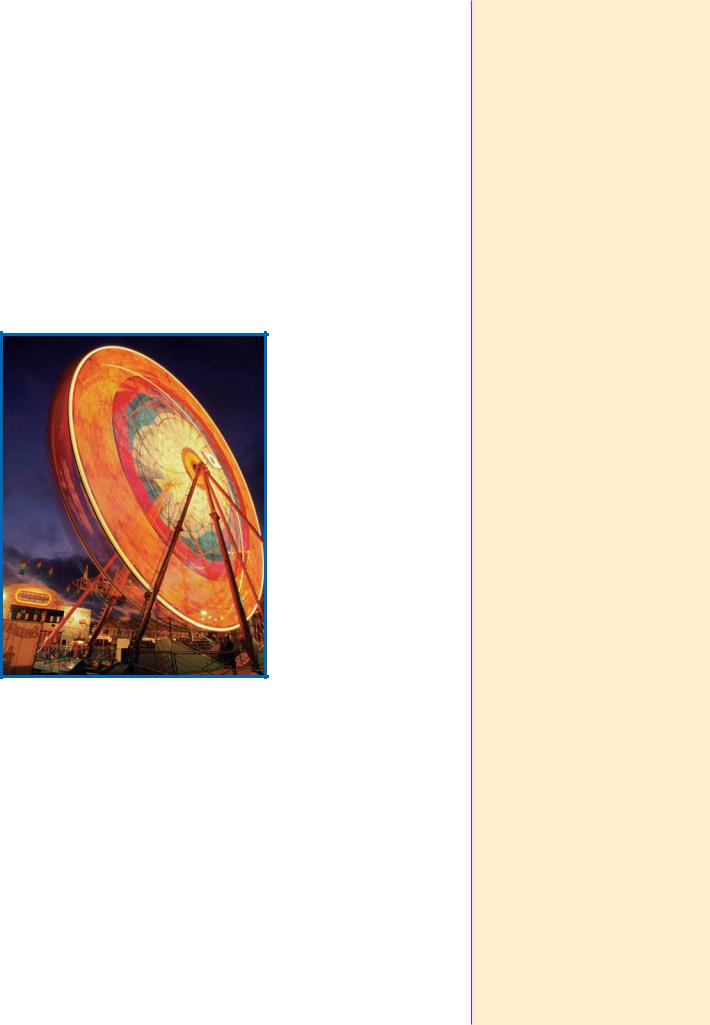
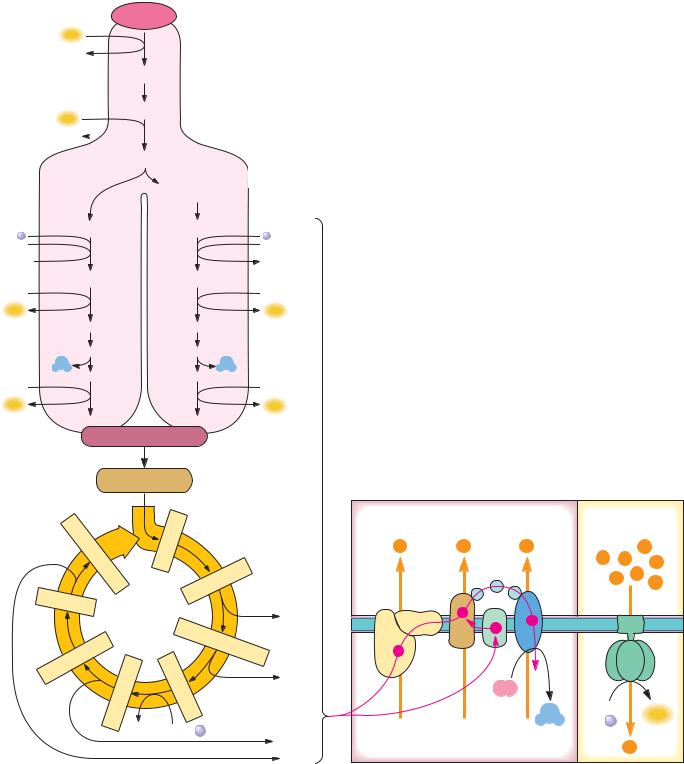