
Dale_Molecular Genetics of Bacteria 4th ed
.pdf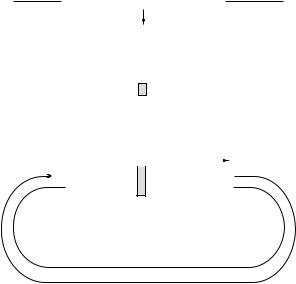
GENETIC MODIFICATION |
237 |
Site-directed mutagenesis |
|
It is possible, using synthetic DNA, to construct |
a totally new gene of |
any sequence. In practice, it is more common to introduce defined alterations in a naturally-occurring gene, a procedure known as site-directed mutagenesis. There are many ways of doing this; the basic concept of one method is shown in Figure 8.14.
A short synthetic oligonucleotide is made that contains the altered sequence and this is hybridized to a plasmid containing the complementary strand of the old gene. Although the sequences are not identical, there is sufficient similarity for binding to occur. The synthetic oligonucleotide (the mismatch primer) can then prime synthesis of the complete DNA strand which now contains the altered sequence. After transformation, the mismatched sequences will both be replicated, so that some cells will contain the new gene and some the original. These can be distinguished using the mismatch primer as a probe. The example in Figure 8.14 represents part of the active site of a b-lactamase in which the serine residue plays an essential role in the hydrolysis of the b-lactam bond of penicillins. Changing this residue to a cysteine results in a novel enzyme with some unusual properties.
Required mutation changes a serine to a cysteine:
pro met met SER thr phe lys
C C A A T G A T G A G C A C T T T T A A G
TGC
CYS
Synthetic oligonucleotide containing the altered base:
CCAATGATGTGCACTTTTAAG
Hybridize oligonucleotide to circular (complementary) single-strand DNA: acts as a primer for synthesis of new DNA strand containing the mutated gene
5 |
|
Primer |
|
|
|
|
|||
|
|
|
|
|
CCAATGATG T GCACTTTTAAG |
GGTTACTAC T CGTGAAAATTC
Mismatch
Figure 8.14 Site-directed mutagenesis. The diagram illustrates the basic principle of the procedure; there are a number of different methods for achieving site-directed mutagenesis
238 |
MOLECULAR GENETICS OF BACTERIA |
Protein engineering
One application of these techniques is in the production of proteins with novel properties. For example, some commercially used enzymes are limited in their application because of temperature instability. If the enzyme were more stable, it would be possible to carry out that process at a higher temperature, thus increasing reaction rates and the overall efficiency of the process. Alternatively minor changes in the amino acid sequence around the substrate binding site could alter the substrates recognized by the enzyme. Changes of this sort are not easy to obtain by conventional genetic means.
This technique, and many refinements thereof, forms the basis of an exciting area of research known as protein engineering. It should be said however, that replacing even a single amino acid in a protein chain can have many untoward effects on the structure of that protein. A completely rational use of protein engineering demands not only a detailed knowledge of the crystal structure of that protein but also an intimate understanding of what determines that conformation and the relationship between the structure and function of the enzyme. This understanding is still essentially fragmentary.
Directed in vitro evolution of protein function
Ways of introducing specific mutations into genes so as to produce enzymes with new functions or improved characteristics, have been discussed in the sections above. However, the specific methods of site-directed mutagenesis suffer not only from limited understanding of the structure and function of the protein, but also become very laborious if a number of changes to the gene are required. An alternative technique, DNA shuffling, allows the accelerated and directed evolution of proteins in vitro through recombination which can be carried out in a test tube using PCR. In this procedure, related genes from different species, or genes with related function, are fragmented. The fragments are then mixed and PCR is used to reassemble them randomly. The new genes are then cloned into E. coli to identify which of them produce usable or potentially interesting products, for example by screening clones for the ability to degrade a novel substrate. It is possible to extend the concept by using synthetic DNA fragments with random sequence to replace selected parts of the gene.
In part therefore this is a return to the older concept of selecting randomly generated strains that have the required characteristics, rather than the more specific methods that are usually associated with gene cloning technology, but using the technology to speed up and extend the generation of new variants.
GENETIC MODIFICATION |
239 |
8.6.3 Other bacterial hosts
Although E. coli is the most versatile host for gene cloning, it has its limitations. One of these is the difficulty of obtaining product secretion. Secretion of proteins is advantageous for commercial production since it facilitates purification of the product. In addition, expression of proteins at a very high level intracellularly can cause the solubility limit to be exceeded, so that the expressed proteins form insoluble aggregates within the cell. These can be damaging to the cell and are also difficult to re-solubilize. Since the extracellular volume of a culture is much larger, this problem is unlikely to arise with a secreted protein. The use of other bacteria, such as Bacillus subtilis, can facilitate secretion of the product.
Furthermore, genetic modification of bacteria is not limited to the production of foreign proteins. Specific alterations of the genetic composition of a bacterium can provide us with invaluable information about biochemical and physiological processes and other important attributes – notably pathogenicity (see Chapter 9). This has involved the development of vectors and other techniques for modifying a wide range of bacteria.
Shuttle vectors
A major problem in applying gene cloning and associated techniques to a wider range of bacteria is that the techniques available for these organisms are limited – for example, transformation (or electroporation) frequencies may be low and the methods for screening gene libraries may be difficult. It is therefore advantageous to carry out the initial cloning or manipulation of a gene using E. coli as the host organism. Then, when the correct piece of DNA has been obtained, it can be recovered from that recombinant and inserted into the target bacterium. This procedure is made much easier by the use of a vector that can replicate in either organism. Such a vector can be constructed by inserting, into an E. coli plasmid, an origin of replication that will function in the second host. This plasmid is then capable of replicating in either bacterium. Since such a vector can be used to carry pieces of DNA between two organisms, it is known as a shuttle vector (see Figure 8.15). Broad host range plasmids (see Chapter 5) can be used as an alternative to dual-origin shuttle vectors.
Protoplast transformation
The transformation system for E. coli as described earlier in this chapter, is not easily adapted for transformation of other bacterial hosts. Other transformation
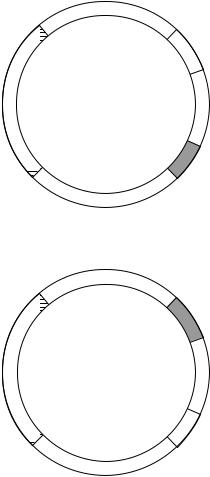
240 |
MOLECULAR GENETICS OF BACTERIA |
In E.coli, the plasmid is replicated using oriE
r |
oriX |
e |
|
k |
|
r |
|
a |
|
m |
|
e |
|
l |
|
b |
|
a |
|
t |
|
c |
|
e |
|
l |
|
e |
|
S |
oriE |
|
In the alternative host, the plasmid is replicated using the second origin, oriX
r |
oriX |
e |
|
k |
|
r |
|
a |
|
m |
|
e |
|
l |
|
b |
|
a |
|
t |
|
c |
|
e |
|
l |
|
e |
|
S |
oriE |
|
Figure 8.15 Shuttle plasmids
systems are needed. One of these is known as protoplast transformation. Enzymatic removal of the cell wall in the presence of an osmotic stabilizer such as sucrose, generates protoplasts in which large areas of the cytoplasmic membrane are exposed. Addition of the DNA solution together with polyethylene glycol (PEG) causes the cells to take up the DNA. The PEG is then removed and the cells allowed to regenerate on an osmotically-stabilized medium. If the correct conditions are used, a very high proportion of the resultant colonies will be transformed. This procedure can be used for transformation of many different bacteria, including Streptomyces and Streptococcus species.
GENETIC MODIFICATION |
241 |
Electroporation
Although protoplast transformation can be achieved with many bacterial species, the efficiency of the process is variable when applied to different species and in some cases may be too low to be of much practical use. It can also be difficult to optimize the conditions for formation and regeneration of protoplasts. Electroporation provides a more versatile alternative. In this procedure, the bacterial cells are mixed with plasmid DNA and are subjected to a brief pulse of high voltage electricity. This probably causes transient tiny holes in the cell membrane through which the DNA enters the cell. The transformants can then be selected on the basis of the antibiotic resistance conferred by the plasmid.
Although a certain amount of work is required to optimize the electroporation conditions, in principle electroporation can be used to introduce DNA into any type of cell.
Agrobacterium tumefaciens: nature’s genetic engineer
As is often the case with new scientific developments, the exuberant scientist is often chastened to find that nature got there first. This is the case with a simple soil bacterium which essentially has been producing transgenic plants for millennia and certainly long before the advent of molecular biology. The soil-borne bacterium Agrobacterium tumefaciens is the causative agent of crown gall disease in plants. In this disease, the gall is actually a plant tumour. One of the most exciting discoveries associated with this bacterium was that formation of the tumour occurs because a specific fragment of bacterial DNA is transferred to the plant cells and incorporated into the plant chromosome. A key component in this mechanism is the tumour-inducing (Ti) plasmid (see Chapter 5). This DNA transfer process has been exploited to create transgenic plants and has become a cornerstone of plant molecular genetics.
8.6.4 Novel vaccines
Until recently, all the vaccines in use were of one of three types: (a) live attenuated vaccines (e.g. oral polio vaccine), which consist of a strain of the pathogen that has been mutated so as to lose its pathogenicity; (b) killed vaccines (e.g. influenza); and
(c) toxoids (diphtheria, tetanus), which consist of a purified toxin that has been inactivated. More recent developments have added additional possibilities, such as subunit vaccines including the acellular pertussis (whooping cough) vaccine which consists of several components purified from whole cells of the pathogen Bordetella pertussis and the Haemophilus influenzae type B (Hib) vaccine which contains the polysaccharide capsule from H. influenzae conjugated to a protein carrier.
242 |
MOLECULAR GENETICS OF BACTERIA |
Genetic technology contributes in several ways to the development of novel vaccines. Firstly, for a subunit vaccine, it is possible to clone a gene and express it at a high level in a more convenient host. Not only does this make it easier to produce large amounts of purified protein, but it also avoids the hazard of growing large amounts of a pathogenic microorganism. The best example is the preparation of a vaccine against the hepatitis B virus. Cloning and expressing the gene that codes for the hepatitis B virus surface antigen enabled the production of a safe and effective vaccine that is now in routine use. (In this case, production is obtained using the yeast Saccharomyces cerevisiae, as the antigen is not produced in the correct form in E. coli).
For some diseases, a live vaccine is required. One way of achieving this is to insert the appropriate gene into the genome of an existing vaccine strain. This produces a recombinant vaccine which expresses the foreign gene and can therefore be administered as a live vaccine. The principal carrier that has been exploited for this purpose is the smallpox vaccine virus, vaccinia. It is in fact possible to include genes from several different infectious agents in the one virus, thus raising the possibility of using a single vaccination to confer protection against a number of diseases simultaneously. In the same vein, bacteria such as attenuated strains of Salmonella typhi and the tuberculosis vaccine BCG are being used for the development of live recombinant vaccines.
Another way of using gene technology for the development of live vaccines is by rational attenuation of the pathogen. If the genes required for pathogenicity but not needed for growth in the laboratory can be identified, then it is possible to use gene knock-out technology (as described in Chapter 9) to specifically inactivate one or more of these genes, thus producing a strain which will be unable to cause disease. This has the advantage over conventional mutation in that it can be ensured that the relevant gene is completely deleted and thus there is no possibility of reversion to virulence. The determination of the complete genome sequence of pathogenic bacteria (see Chapter 9) is playing an important part in identifying potential targets for rational attenuation by gene replacement.
Gene cloning provides yet another, even more novel, route for vaccination. If the gene coding for the key antigen is inserted into a plasmid vector under the control of a promoter which functions in an animal cell, then this recombinant plasmid is by itself able to elicit an immune response. These DNA vaccines are still at an experimental stage and much remains to be determined regarding their safety and efficacy.
8.7 Other uses of gene technology
In this chapter the focus has been deliberately concentrated on the applications of gene technology to bacteria. Some of the most publicized uses of this technology –
GENETIC MODIFICATION |
243 |
notably molecular diagnosis of inherited diseases, human DNA fingerprinting, gene therapy for the treatment of human diseases and the production of transgenic plants and animals – are beyond the scope of this book. These topics are covered in the further reading suggestions in Appendix A.

9
Genetic Methods for
Investigating Bacteria
Genetics has been central to the development of our understanding of bacterial metabolism and physiology and some examples will be reviewed in this chapter. In Chapter 10 the discussion will be extended to include the lessons that can be learned from genome sequencing, amongst other things. Although genomics receives most of the publicity, the techniques in this chapter are important for understanding the function of the genes concerned in relation to the overall behaviour of the cell.
9.1 Metabolic pathways
The initial impetus for the use of genetic analysis to investigate metabolic pathways came from the work of Beadle and Tatum in the 1940s. Although their work was with the fungus Neurospora, many of the principles were applied subsequently to bacteria. The concepts will be illustrated in relation to an hypothetical pathway leading to the formation of an essential product P (see Figure 9.1). Auxotrophic mutants that are unable to grow unless P is provided should be of three types corresponding to the three steps involved and can be assigned to three groups by complementation analysis.
|
1 |
2 |
3 |
S |
A |
B |
P |
Figure 9.1 An unbranched metabolic pathway
Molecular Genetics of Bacteria, 4th Edition by Jeremy Dale and Simon F. |
Park |
# 2004 John Wiley & Sons, Ltd ISBN 0 470 85084 1 (cased) ISBN 0 |
470 85085 X (pbk) |
246 |
MOLECULAR GENETICS OF BACTERIA |
9.1.1 Complementation
In Chapter 4, it was shown that a mixed infection with two mutant bacteriophages could result in complementation between the two mutants if the two phage strains carry mutations in different genes, so that between them they have a full set of functional genes. If complementation analysis is applied to mutants of a pathway such as that in Figure 9.1, the mutants could be assigned to three groups corresponding to the step in which they are deficient, assuming each enzyme contains a single polypeptide. For example, mutants defective in gene 1 would not be able to complement one another, but they would be able to complement mutants defective in either of the other two genes. Complementation analysis of this sort is easier to undertake in Neurospora than in bacterial systems (apart from phage).
In bacteria complementation is encountered more commonly in connection with plasmids. Introducing a plasmid can create a partial diploid with one version of a gene on the chromosome and another version on the plasmid. If the chromosomal gene is defective, it can be complemented by the plasmid. Partial diploids and F0 plasmids were discussed in Chapter 6 and in Chapter 8 plasmid complementation was described as a method of screening a gene library.
9.1.2 Cross-feeding
Complementation analysis does not identify the steps involved nor the order in which they occur. Additional information is obtained by a procedure known as cross-feeding, as illustrated in Figure 9.2. None of these mutants is able to grow on a medium that lacks the essential nutrient P. However if the plate is inoculated with each mutant in the pattern shown, then intermediates accumulated by a mutant may stimulate the growth of the neighbouring mutant. For example mutant I is unable to produce the intermediate A, but this is produced by mutant II. So the growth of mutant A is stimulated where it is near to mutant II. Similarly, growth of mutant II is stimulated by mutant III. A cross-feeding experiment can therefore categorize mutants in a way that corresponds to the steps in the biochemical pathway.
Neither of these procedures identifies the steps involved or the nature of the intermediates. However, an hypothetical pathway can be tested by examining the ability of the predicted intermediates to stimulate the growth of each mutant. Such an analysis was used to confirm the role of ornithine and citrulline as intermediates in the pathway of arginine biosynthesis. One class of mutants responded only to arginine, a second class responded to either arginine or citrulline, while the third group responded to arginine, ornithine or citrulline. This established that the synthetic sequence went from ornithine to citrulline to arginine.