
Rotorcraft Flying Handbook
.pdf
Ch 11.qxd 7/15/2003 9:14 AM Page 11-4
the ground track approximately 200 feet away from the touchdown point. If a strong crosswind exists, it will be necessary to move your downwind leg closer or farther out. When abeam the intended touchdown point, reduce collective, and then split the needles. Apply proper antitorque pedal and cyclic to maintain proper attitude. Cross check attitude, trim, rotor r.p.m., and airspeed.
After the descent and airspeed is established, roll into a 180° turn. For training, you should initially roll into a bank of a least 30°, but no more than 40°. Check your airspeed and rotor r.p.m. Throughout the turn, it is important to maintain the proper airspeed and keep the aircraft in trim. Changes in the aircraft’s attitude and the angle of bank cause a corresponding change in rotor r.p.m. Adjust the collective, as necessary, in the turn to maintain rotor r.p.m. in the green arc.
At the 90° point, check the progress of your turn by glancing toward your landing area. Plan the second 90 degrees of turn to roll out on the centerline. If you are too close, decrease the bank angle; if too far out, increase the bank angle. Keep the helicopter in trim with antitorque pedals.
The turn should be completed and the helicopter aligned with the intended touchdown area prior to passing through 100 feet AGL. If the collective pitch was increased to control the r.p.m., it may have to be lowered on roll out to prevent a decay in r.p.m. Make an immediate power recovery if the aircraft is not aligned with the touchdown point, and if the rotor r.p.m. and/or airspeed is not within proper limits.
From this point, complete the procedure as if it were a straight-in autorotation.
POWER FAILURE IN A HOVER
Power failures in a hover, also called hovering autorotations, are practiced so that you automatically make the correct response when confronted with engine stoppage or certain other emergencies while hovering. The techniques discussed in this section refer to helicopters with a counter-clockwise rotor system and an antitorque rotor.
TECHNIQUE
To practice hovering autorotations, establish a normal hovering altitude for the particular helicopter being used, considering load and atmospheric conditions. Keep the helicopter headed into the wind and hold maximum allowable r.p.m.
To simulate a power failure, firmly roll the throttle into the spring loaded override position, if applicable. This disengages the driving force of the engine from the rotor, thus eliminating torque effect. As the throttle is closed, apply proper antitorque pedal to maintain heading. Usually, a slight amount of right cyclic control is necessary to keep the helicopter from drifting to the left, to compensate for the loss of tail rotor thrust. However, use cyclic control, as required, to ensure a vertical descent and a level attitude. Leave the collective pitch where it is on entry.
Helicopters with low inertia rotor systems will begin to settle immediately. Keep a level attitude and ensure a vertical descent with cyclic control while maintaining heading with the pedals. At approximately 1 foot above the surface, apply upward collective pitch control, as necessary, to slow the descent and cushion the landing. Usually the full amount of collective pitch is required. As upward collective pitch control is applied, the throttle has to be held in the closed position to prevent the rotor from re-engaging.
Helicopters with high inertia rotor systems will maintain altitude momentarily after the throttle is closed. Then, as the rotor r.p.m. decreases, the helicopter starts to settle. When the helicopter has settled to approximately 1 foot above the surface, apply upward collective pitch control while holding the throttle in the closed position to slow the descent and cushion the landing. The timing of collective pitch control application, and the rate at which it is applied, depends upon the particular helicopter being used, its gross weight, and the existing atmospheric conditions. Cyclic control is used to maintain a level attitude and to ensure a vertical descent. Maintain heading with antitorque pedals.
When the weight of the helicopter is entirely on the skids, cease the application of upward collective. When the helicopter has come to a complete stop, lower the collective pitch to the full down position.
The timing of the collective pitch is a most important consideration. If it is applied too soon, the remaining r.p.m. may not be sufficient to make a soft landing. On the other hand, if collective pitch control is applied too late, surface contact may be made before sufficient blade pitch is available to cushion the landing.
COMMON ERRORS
1.Failing to use sufficient proper antitorque pedal when power is reduced.

Ch 11.qxd 7/15/2003 9:14 AM Page 11-5
Figure 11-2. By carefully studying the height/velocity diagram, you will be able to avoid the combinations of altitude and airspeed that may not allow you sufficient time or altitude to enter a stabilized autorotative descent. You might want to refer to this diagram during the remainder of the discussion on the height/velocity diagram.
2.Failing to stop all sideward or backward movement prior to touchdown.
3.Failing to apply up-collective pitch properly, resulting in a hard touchdown.
4.Failing to touch down in a level attitude.
5.Not rolling the throttle completely to idle.
HEIGHT/VELOCITY DIAGRAM
A height/velocity (H/V) diagram, published by the manufacturer for each model of helicopter, depicts the critical combinations of airspeed and altitude should an engine failure occur. Operating at the altitudes and airspeeds shown within the crosshatched or shaded areas of the H/V diagram may not allow enough time for the critical transition from powered flight to autorotation. [Figure 11-2]
An engine failure in a climb after takeoff occurring in section A of the diagram is most critical. During a climb, a helicopter is operating at higher power settings and blade angle of attack. An engine failure at this point causes a rapid rotor r.p.m. decay because the upward movement of the helicopter must be stopped, then a descent established in order to drive the rotor. Time is also needed to sta-
bilize, then increase the r.p.m. to the normal operating range. The rate of descent must reach a value that is normal for the airspeed at the moment. Since altitude is insufficient for this sequence, you end up with decaying r.p.m., an increasing sink rate, no deceleration lift, little translational lift, and little response to the application of collective pitch to cushion the landing.
It should be noted that, once a steady state autorotation has been established, the H/V diagram no longer applies. An engine failure while descending through section A of the diagram, is less critical, provided a safe landing area is available.
You should avoid the low altitude, high airspeed portion of the diagram (section B), because your recognition of an engine failure will most likely coincide with, or shortly occur after, ground contact. Even if you detect an engine failure, there may not be sufficient time to rotate the helicopter from a nose low, high airspeed attitude to one suitable for slowing, then landing. Additionally, t h e altitude loss that occurs during recognition of engine failure and rotation to a landing attitude, may not leave enough altitude to prevent the tail skid from hitting the ground during the landing maneuver.
Basically, if the helicopter represented by this H/V diagram is above 445 feet AGL, you have enough time and altitude to enter a steady state autorotation, regardless of your airspeed. If the helicopter is hovering at 5 feet AGL (or less) in normal conditions and the engine fails, a safe hovering autorotation can be made. Between approximately 5 feet and 445 feet AGL, however, the transition to autorotation depends on the altitude and airspeed
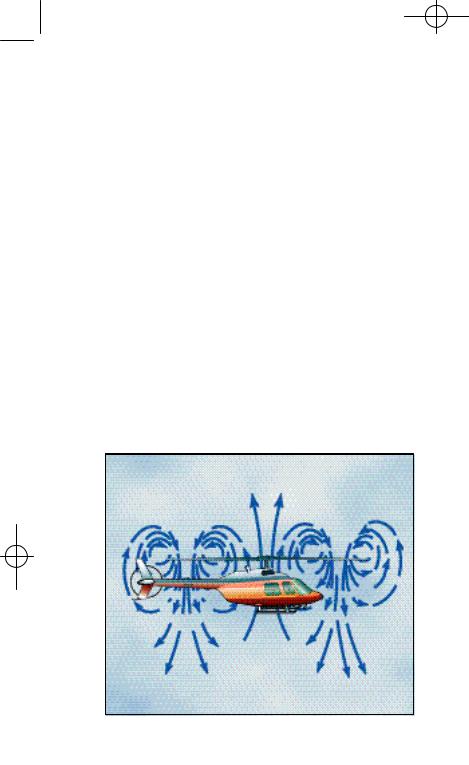
Ch 11.qxd 7/15/2003 9:14 AM Page 11-6
of the helicopter. Therefore, you should always be familiar with the height/velocity diagram for the particular model of helicopter you are flying.
THE EFFECT OF WEIGHT VERSUS DENSITY ALTITUDE
The height/velocity diagram depicts altitude and airspeed situations from which a successful autorotation can be made. The time required, and therefore, altitude necessary to attain a steady state autorotative descent, is dependent on the weight of the helicopter and the density altitude. For this reason, the H/V diagram for some helicopter models is valid only when the helicopter is operated in accordance with the gross weight vs. density altitude chart. Where appropriate, this chart is found in the rotorcraft flight manual for the particular helicopter. [Figure 11-3]
Figure 11-4. Vortex ring state.
Figure 11-3. Assuming a density altitude of 5,500 feet, the height/velocity diagram in figure 11-2 would be valid up to a gross weight of approximately 1,700 pounds. This is found by entering the graph at a density altitude of 5,500 feet (point A), then moving horizontally to the solid line (point B). Moving vertically to the bottom of the graph (point C), you find that with the existing density altitude, the maximum gross weight under which the height/velocity diagram is applicable is 1,700 pounds.
The gross weight vs. density altitude chart is not intended as a restriction to gross weight, but as an advisory to the autorotative capability of the heli- c o p t e r during takeoff and climb. You must realize, however, that at gross weights above those recom-
mended by the gross weight vs. density altitude chart, the H/V diagram is not restrictive enough.
VORTEX RING STATE (SETTLING WITH POWER)
Vortex ring state describes an aerodynamic condition where a helicopter may be in a vertical descent with up to maximum power applied, and little or no cyclic authority. The term “settling with power” comes from the fact that helicopter keeps settling even though full engine power is applied.
In a normal out-of-ground-effect hover, the helicopter is able to remain stationary by propelling a large mass of air down through the main rotor. Some of the air is recirculated near the tips of the blades, curling up from the bottom of the rotor system and rejoining the air entering the rotor from the top. This phenomenon is common to all airfoils and is known as tip vortices. Tip vortices consume engine power but produce no useful lift. As long as the tip vortices are small, their only effect is a small loss in rotor efficiency. However, when the helicopter begins to descend vertically, it settles into its own downwash, which greatly enlarges the tip vortices. In this vortex ring state, most of the power developed by the engine is wasted in accelerating the air in a doughnut pattern around the rotor.
In addition, the helicopter may descend at a rate that exceeds the normal downward induced-flow rate of the inner blade sections. As a result, the airflow of the inner blade sections is upward relative to the disc. This produces a secondary vortex ring in addition to the normal tip-vortices. The secondary vortex ring is generated about the point on the blade where the airflow changes from up to down. The result is an unsteady turbulent flow over a large area of the disc. Rotor efficiency is lost even though power is still being supplied from the engine. [Figure 11-4]
A fully developed vortex ring state is characterized by an unstable condition where the helicopter experiences uncommanded pitch and roll oscillations, has little or no cyclic authority, and achieves a descent rate, which, if allowed to develop, may approach 6,000 feet per minute. It is accompanied by increased levels of vibration.
A vortex ring state may be entered during any maneuver that places the main rotor in a condition of high upflow and low forward airspeed. This condition is sometimes seen during quick-stop type maneuvers or during recoveries from autorotations. The

Ch 11.qxd 7/15/2003 9:14 AM Page 11-7
following combination of conditions are likely to cause settling in a vortex ring state:
1.A vertical or nearly vertical descent of at least 300 feet per minute. (Actual critical rate depends on the gross weight, r.p.m., density altitude, and other pertinent factors.)
2.The rotor system must be using some of the available engine power (from 20 to 100 percent).
3.The horizontal velocity must be slower than effective translational lift.
Some of the situations that are conducive to a settling with power condition are: attempting to hover out of ground effect at altitudes above the hovering ceiling of the helicopter; attempting to hover out of ground effect without maintaining precise altitude control; or downwind and steep power approaches in which airspeed is permitted to drop to nearly zero.
When recovering from a settling with power condition, the tendency on the part of the pilot is to first try to stop the descent by increasing collective pitch. However, this only results in increasing the stalled area of the rotor, thus increasing the rate of descent. Since inboard portions of the blades are stalled, cyclic control is limited. Recovery is accomplished by increasing forward speed, and/or partially lowering collective pitch. In a fully developed vortex ring state, the only recovery may be to enter autorotation to break the vortex ring state. When cyclic authority is regained, you can then increase forward airspeed.
For settling with power demonstrations and training in recognition of vortex ring state conditions, all maneuvers should be performed at an elevation of at least 1,500 feet AGL.
To enter the maneuver, reduce power below hover power. Hold altitude with aft cyclic until t h e airspeed approaches 20 knots. Then allow the sink rate to increase to 300 feet per minute or more as the attitude is adjusted to obtain an airspeed of less than 10 knots. When the aircraft begins to shudder, the application of additional up collective increases the vibration and sink rate.
Recovery should be initiated at the first sign of vortex ring state by applying forward cyclic to increase airspeed and simultaneously reducing
collective. The recovery is complete when the aircraft passes through effective translational lift and a normal climb is established.
RETREATING BLADE STALL
In forward flight, the relative airflow through the main rotor disc is different on the advancing and retreating side. The relative airflow over the advancing side is higher due to the forward speed of the helicopter, while the relative airflow on the retreating side is lower. This dissymmetry of lift increases as forward speed increases.
To generate the same amount of lift across the rotor disc, the advancing blade flaps up while the retreating blade flaps down. This causes the angle of attack to decrease on the advancing blade, which reduces lift, and increase on the retreating blade, which increases lift. As the forward speed increases, at some point the low blade speed on the retreating blade, together with its high angle of attack, causes a loss of lift (stall).
Retreating blade stall is a major factor in limiting a helicopter’s top forward speed (VNE) and can be felt developing by a low frequency vibration, pitching up of the nose, and a roll in the direction of the retreating blade. High weight, low rotor r.p.m., high density altitude, turbulence and/or steep, abrupt turns are all conducive to retreating blade stall at high forward airspeeds. As altitude is increased, higher blade angles are required to maintain lift at a given airspeed. Thus, retreating blade stall is encountered at a lower forward airspeed at altitude. Most manufacturers publish charts and graphs showing a VNE decrease with altitude.

Ch 11.qxd 7/15/2003 9:14 AM Page 11-8
When recovering from a retreating blade stall condition, moving the cyclic aft only worsens the stall as aft cyclic produces a flare effect, thus increasing angles of attack. Pushing forward on the cyclic also deepens the stall as the angle of attack on the retreating blade is increased. Correct recovery from retreating blade stall requires the collective to be lowered first, which reduces blade angles and thus angle of attack. Aft cyclic can then be used to slow the helicopter.
GROUND RESONANCE
Ground resonance is an aerodynamic phenomenon associated with fully-articulated rotor systems. It develops when the rotor blades move out of phase with each other and cause the rotor disc to become unbalanced. This condi - tion can cause a helicopter to self-destruct in a matter of seconds. However, for this condition to occur, the helicopter must be in contact with the ground.
If you allow your helicopter to touch down firmly on one corner (wheel type landing gear is most conducive for this) the shock is transmitted to the main rotor system. This may cause the blades to move out of their normal relationship with each other. This movement occurs along the drag hinge. [Figure 11-5]
Figure 11-5. Hard contact with the ground can send a shock wave to the main rotor head, resulting in the blades of a three-bladed rotor system moving from their normal 120° relationship to each other. This could result in something like 122°, 122°, and 116° between blades. When one of the other landing gear strikes the surface, the unbalanced condition could be further aggravated.
If the r.p.m. is low, the corrective action to stop ground resonance is to close the throttle immediately and fully lower the collective to place the blades in low pitch. If the r.p.m. is in the normal operating range, you should fly the helicopter off the ground, and allow the blades to automatically realign themselves. You can then make a normal touchdown. If you lift off and allow the helicopter to firmly re-contact the surface before the blades are realigned, a second shock could move the blades again and aggravate the already unbalanced condition. This could lead to a violent, uncontrollable oscillation.
This situation does not occur in rigid or semirigid rotor systems, because there is no drag hinge. In addition, skid type landing gear are not as prone to ground resonance as wheel type gear.
DYNAMIC ROLLOVER
A helicopter is susceptible to a lateral rolling tendency, called dynamic rollover, when lifting off the surface. For dynamic rollover to occur, some factor has to first cause the helicopter to roll or pivot around a skid, or landing gear wheel, until its critical rollover angle is reached. Then, beyond this point, main rotor thrust continues the roll and recovery is impossible. If the critical rollover angle is exceeded, the helicopter rolls on its side regardless of the cyclic corrections made.
Dynamic rollover begins when the helicopter starts to pivot around its skid or wheel. This can occur for a variety of reasons, including the failure
Figure 11-6. Forces acting on a helicopter with right skid on the ground.
Figure 11-7. Upslope rolling motion.

Ch 11.qxd 7/15/2003 9:14 AM Page 11-9
to remove a tiedown or skid securing device, or if the skid or wheel contacts a fixed object while hovering sideward, or if the gear is stuck in ice, soft asphalt, or mud. Dynamic rollover may also occur if you do not use the proper landing or takeoff technique or while performing slope operations. Whatever the cause, if the gear or skid becomes a pivot point, dynamic rollover is possible if you do not use the proper corrective technique.
Once started, dynamic rollover cannot be stopped by application of opposite cyclic control alone. For example, the right skid contacts an object and becomes the pivot point while the helicopter starts rolling to the right. Even with full left cyclic applied, the main rotor thrust vector and its moment follows the aircraft as it continues rolling to the right. Quickly applying down collective is the most effective way to stop dynamic rollover from developing. Dynamic rollover can occur in both skid and wheel equipped helicopters, and all types of rotor systems.
CRITICAL CONDITIONS
Certain conditions reduce the critical rollover angle, thus increasing the possibility for dynamic rollover and reducing the chance for recovery. The rate of rolling motion is also a consideration, because as the roll rate increases, the critical
Figure 11-8. Downslope rolling motion.
rollover angle at which recovery is still possible, is reduced. Other critical conditions include operating at high gross weights with thrust (lift) approximately equal to the weight.
Refer to figure 11-6. The following conditions are most critical for helicopters with counter-clock - wise rotor rotation:
1.right side skid/wheel down, since translating tendency adds to the rollover force.
2.right lateral center of gravity.
3.crosswinds from the left.
4.left yaw inputs.
For helicopters with clockwise rotor rotation, the opposite would be true.
CYCLIC TRIM
When maneuvering with one skid or wheel on the ground, care must be taken to keep the helicopter cyclic control properly trimmed. For example, if a slow takeoff is attempted and the cyclic is not positioned and trimmed to account for translating tendency, the critical recovery angle may be exceeded in less than two seconds. Control can be maintained if you maintain proper cyclic position and trim, and not allow the helicopter’s roll and pitch rates to become too great. You should fly your helicopter into the air smoothly while keeping movements of pitch, roll, and yaw small, and not allow any untrimmed cyclic pressures.
NORMAL TAKEOFFS AND LANDINGS
Dynamic rollover is possible even during normal takeoffs and landings on relative level ground, if one wheel or skid is on the ground and thrust (lift) is approximately equal to the weight of the helicopter. If the takeoff or landing is not performed properly, a roll rate could develop around the wheel or skid that is on the ground. When taking off or landing, perform the maneuver smoothly and trim the cyclic so that no pitch or roll movement rates build up, especially the roll rate. If the bank angle starts to increase to an angle of approximately 5 to 8°, and full corrective cyclic does not reduce the angle, the collective should be reduced to diminish the unstable rolling condition.
SLOPE TAKEOFFS AND LANDINGS
During slope operations, excessive application of cyclic control into the slope, together with excessive collective pitch control, can result in the downslope skid rising sufficiently to exceed lateral cyclic control limits, and an upslope rolling motion can occur. [Figure 11-7]
When performing slope takeoff and landing maneuvers, follow the published procedures and keep the roll rates small. Slowly raise the downs-

Ch 11.qxd 7/15/2003 9:14 AM Page 11-10
lope skid or wheel to bring the helicopter level, and then lift off. During landing, first touch down on the upslope skid or wheel, then slowly lower the downslope skid or wheel using combined movements of cyclic and collective. If the helicopter rolls approximately 5 to 8° to the upslope side, decrease collective to correct the bank angle and return to level attitude, then start the landing procedure again.
USE OF COLLECTIVE
The collective is more effective in controlling the rolling motion than lateral cyclic, because it reduces the main rotor thrust (lift). A smooth, moderate collective reduction, at a rate less than approximately full up to full down in two seconds, is adequate to stop the rolling motion. Take care, however, not to dump collective at too high a rate, as this may cause a main rotor blade to strike the fuselage. Additionally, if the helicopter is on a slope and the roll starts to the upslope side, reducing collective too fast may create a high roll rate in the opposite direction. When the upslope skid/wheel hits the ground, the dynamics of the motion can cause the helicopter to bounce off the upslope skid/wheel, and the inertia can cause the helicopter to roll about the downslope ground contact point and over on its side. [Figure 11-8]
The collective should not be pulled suddenly to get airborne, as a large and abrupt rolling moment in the opposite direction could occur. Excessive application of collective can result in the upslope skid rising sufficiently to exceed lateral cyclic con-
Figure 11-9. In a low G condition, improper corrective action could lead to the main rotor hub contacting the rotor mast. The contact with the mast becomes more violent with each successive flapping motion. This, in turn, creates a greater flapping displacement. The result could be a severely damaged rotor mast, or the main rotor system could separate from the helicopter.
trol limits. This movement may be uncontrollable. If the helicopter develops a roll rate with one skid/wheel on the ground, the helicopter can roll over on its side.
PRECAUTIONS
The following lists several areas to help you avoid dynamic rollover.
1.Always practice hovering autorotations into the wind, but never when the wind is gusty or over 10 knots.
2.When hovering close to fences, sprinklers, bushes, runway/taxi lights, or other obstacles that could catch a skid, use extreme caution.
3.Always use a two-step liftoff. Pull in just enough collective pitch control to be light on the skids and feel for equilibrium, then gently
lift |
the |
helicopter into the air. |
|
4.When practicing hovering maneuvers close to the ground, make sure you hover high enough to have adequate skid clearance with any obstacles, especially when practicing sideways or rearward flight.
5.When the wind is coming from the upslope direction, less lateral cyclic control will be available.
6.Tailwind conditions should be avoided when conducting slope operations.
7.When the left skid/wheel is upslope, less lateral cyclic control is available due to the translating tendency of the tail rotor. (This is true for counter-rotating rotor systems)
8.If passengers or cargo are loaded or unloaded, the lateral cyclic requirement changes.
9.If the helicopter utilizes interconnecting fuel lines that allow fuel to automatically transfer from one side of the helicopter to the other, the gravitational flow of fuel to the downslope tank could change the center of gravity, resulting in a different amount of cyclic control application to obtain the same lateral result.
10.Do not allow the cyclic limits to be reached. If the cyclic control limit is reached, further lowering of the collective may cause mast bumping. If this occurs, return to a hover and select a landing point with a lesser degree of slope.
11.During a takeoff from a slope, if the upslope skid/wheel starts to leave the ground before the downslope skid/wheel, smoothly and

Ch 11.qxd 7/15/2003 9:14 AM Page 11-11
gently lower the collective and check to see if the downslope skid/wheel is caught on something. Under these conditions vertical ascent is the only acceptable method of liftoff.
12.During flight operations on a floating platform, if the platform is pitching/rolling while attempting to land or takeoff, the result could be dynamic rollover.
LOW G CONDITIONS AND MAST BUMPING
For cyclic control, small helicopters depend primarily on tilting the main rotor thrust vector to pro- d u c e control moments about the aircraft center of gravity (CG), causing the helicopter to roll or pitch in the desired direction. Pushing the cyclic control forward abruptly from either straight-and-level flight or after a climb can put the helicopter into a low G (weightless) flight condition. In forward flight, when a push-over is performed, the angle of attack and thrust of the rotor is reduced, causing a low G or weightless flight condition. During the low G condition, the lateral cyclic has little, if any, effect because the rotor thrust has been reduced. Also, in a counter-clock- wise rotor system (a clockwise system would be the reverse), there is no main rotor thrust component to the left to counteract the tail rotor thrust to the right, and since the tail rotor is above the CG, the tail rotor thrust causes the helicopter to roll rapidly to the right, If you attempt to stop the right roll by applying full left cyclic before regaining main rotor thrust, the rotor can exceed its flapping limits and cause structural failure of the rotor shaft due to mast bumping, or it may allow a blade to contact the airframe. [Figure 11-9]
Since a low G condition could have disastrous results, the best way to prevent it from happening is to avoid the conditions where it might occur. This means avoiding turbulence as much as possible. If you do encounter turbulence, slow your forward airspeed and make small control inputs. If turbulence becomes excessive, consider making a precautionary landing. To help prevent turbulence induced inputs, make sure your cyclic arm is properly supported. One way to accomplish this is to brace your arm against your leg. Even if you are not in turbulent conditions, you should avoid abrupt movement of the cyclic and collective.
If you do find yourself in a low G condition, which can be recognized by a feeling of weightlessness
and an uncontrolled roll to the right, you should immediately and smoothly apply aft cyclic. Do not attempt to correct the rolling action with lateral cyclic. By applying aft cyclic, you will load the rotor system, which in turn produces thrust. Once thrust is restored, left cyclic control becomes effective, and you can roll the helicopter to a level attitude.
LOW ROTOR RPM AND BLADE STALL
As mentioned earlier, low rotor r.p.m. during an autorotation might result in a less than successful maneuver. However, if you let rotor r.p.m. decay to the point where all the rotor blades stall, the result is usually fatal, especially when it occurs at altitude. The danger of low rotor r.p.m. and blade stall is greatest in small helicopters with low blade inertia. It can occur in a number of ways, such as simply rolling the throttle the wrong way, pulling more collective pitch than power available, or when operating at a high density altitude.
When the rotor r.p.m. drops, the blades try to maintain the same amount of lift by increasing pitch. As the pitch increases, drag increases, which requires more power to keep the blades turning at the proper r.p.m. When power is no longer available to maintain r.p.m., and therefore lift, the helicopter begins to descend. This changes the relative wind and further increases the angle of attack. At some point the blades will stall unless r.p.m. is restored. If all blades stall, it is almost impossible to get smooth air flowing across the blades.
Even though there is a safety factor built into most helicopters, anytime your rotor r.p.m. falls below the green arc, and you have power, simultaneously add throttle and lower the collective. If you are in forward flight, gently applying aft cyclic loads up the rotor system and helps increase rotor r.p.m. If you are without power, immediately lower the collective and apply aft cyclic.
RECOVERY FROM LOW ROTOR RPM
Under certain conditions of high weight, high temperature, or high density altitude, you might get into a situation where the r.p.m. is low even though you are using maximum throttle. This is usually the result of the main rotor blades having an angle of attack that has created so much drag that engine power is not sufficient to maintain or attain normal operating r.p.m.
If you are in a low r.p.m. situation, the lifting power of the main rotor blades can be greatly diminished. As
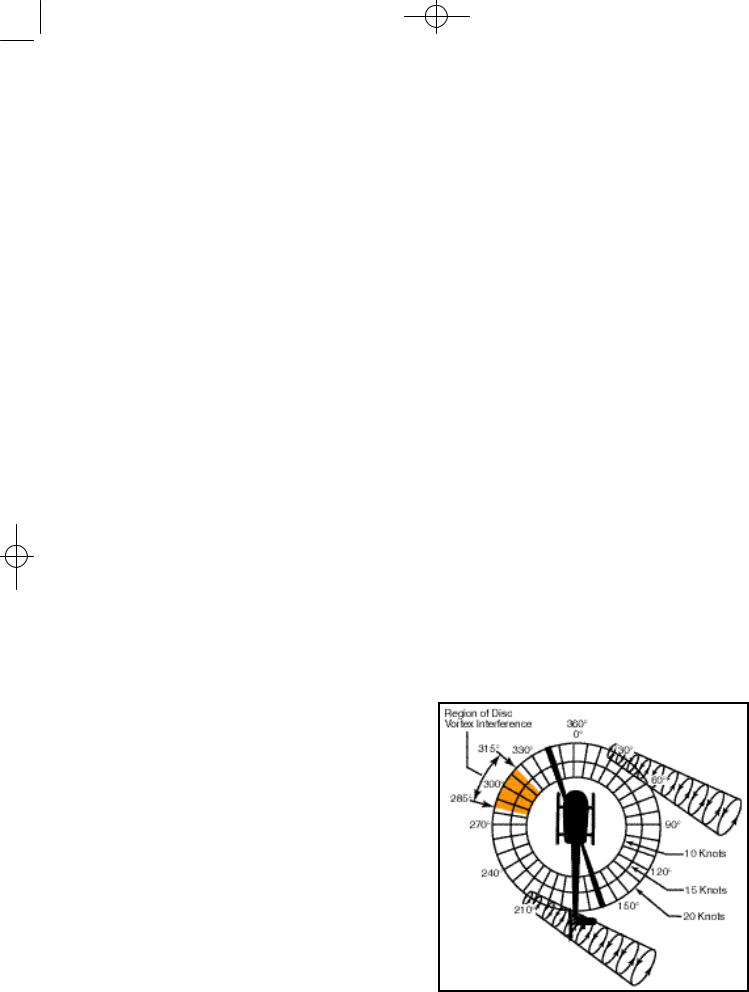
Ch 11.qxd 7/15/2003 9:14 AM Page 11-12
soon as you detect a low r.p.m. condition, immediately apply additional throttle, if available, while slightly lowering the collective. This reduces main rotor pitch and drag. As the helicopter begins to settle, smoothly raise the collective to stop the descent. At hovering altitude you may have to repeat this technique several times to regain normal operating r.p.m. This technique is sometimes called “milking the collective.” When operating at altitude, the collective may have to be lowered only once to regain rotor speed. The amount the collective can be lowered depends on altitude. When hovering near the surface, make sure the helicopter does not contact the ground as the collective is lowered.
Since the tail rotor is geared to the main rotor, low main rotor r.p.m. may prevent the tail rotor from producing enough thrust to maintain directional control. If pedal control is lost and the altitude is low enough that a landing can be accomplished before the turning rate increases dangerously, slowly decrease collective pitch, maintain a level attitude with cyclic control, and land.
SYSTEM MALFUNCTIONS
The reliability and dependability record of modern helicopters is very impressive. By following the manufacturer’s recommendations regarding peri- o d i c maintenance and inspections, you can eliminate most systems and equipment failures. Most malfunctions or failures can be traced to some error on the part of the pilot; therefore, most emergencies can be averted before they happen. An actual emergency is a rare occurrence.
ANTITORQUE SYSTEM FAILURE
Antitorque failures usually fall into two categories. One focuses on failure of the power drive portion of the tail rotor system resulting in a complete loss of antitorque. The other category covers mechanical control failures where the pilot is unable to change or control tail rotor thrust even though the tail rotor may still be providing antitorque thrust.
Tail rotor drive system failures include driveshaft failures, tail rotor gearbox failures, or a complete loss of the tail rotor itself. In any of these cases, the loss of antitorque normally results in an immediate yawing of the helicopter’s nose. The helicopter yaws to the right in a counter-clockwise rotor system and to the left in a clockwise system. This discussion assumes a helicopter with a counter-clockwise rotor system. The severity of the yaw is proportionate to the amount of power being used and the airspeed. An a n t i t o r q u e
failure with a high power setting at a low airspeed results in a severe yawing to the right. At low power settings and high airspeeds, the yaw is less severe. High airspeeds tend to streamline the helicopter and keep it from spinning.
If a tail rotor failure occurs, power has to be reduced in order to reduce main rotor torque. The tech- n i q u e s differ depending on whether the helicopter is in f l i g h t or in a hover, but will ultimately require an autorotation. If a complete tail rotor failure occurs while hovering, enter a hovering autorotation by rolling off the throttle. If the failure occurs in forward flight, enter a normal autorotation by lowering the collec- t i v e and rolling off the throttle. If the helicopter has enough forward airspeed (close to cruising speed) when the failure occurs, and depending on the helicopter design, the vertical stabilizer may provide enough directional control to allow you to maneuver the helicopter to a more desirable landing sight. Some of the yaw may be compensated for by applying slight cyclic control opposite the direction of yaw. This helps in directional control, but also increases drag. Care must be taken not to lose too much forward airspeed because the streamlining effect diminishes as airspeed is reduced. Also, more altitude is required to accelerate to the correct airspeed if an autorotation is entered into at a
low airspeed.
A mechanical control failure limits or prevents control of tail rotor thrust and is usually caused by a stuck or broken control rod or cable. While the tail rotor is still producing antitorque thrust,
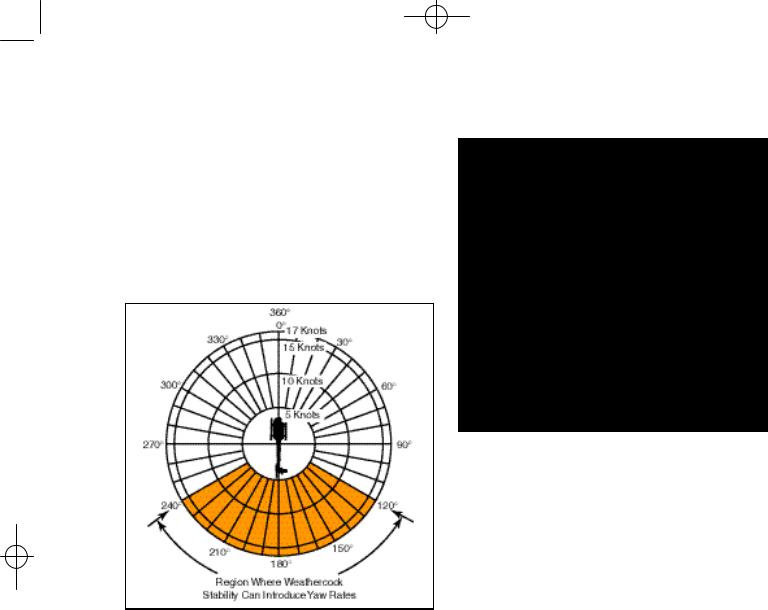
Ch 11.qxd 7/15/2003 9:14 AM Page 11-13
it cannot be controlled by the pilot. The amount of antitorque depends on the position where the controls jam or fail. Once again, the techniques differ depending on the amount of tail rotor thrust, but an autorotation is generally not required.
LANDING—STUCK LEFT PEDAL
Be sure to follow the procedures and techniques outlined in the FAA-approved rotorcraft flight manual for the helicopter you are flying. A stuck left pedal, such as might be experienced during takeoff or climb conditions, results in the heli -
copter’s nose yawing to the left when power is reduced. Rolling off the throttle and entering an autorotation only makes matters worse. The landing profile for a stuck left pedal is best described as a normal approach to a momentary hover at three to four feet above the surface. Following an analysis, make the landing. If the helicopter is not turning, simply lower t h e helicopter to the surface. If the helicopter is turning to the right, roll the throttle toward flight idle the amount necessary to stop the turn as you land. If the helicopter is beginning to turn left, you should be able to make the landing prior to the turn rate becoming excessive. However, if the turn rate becomes excessive prior to the landing, simply execute a takeoff and return for another landing.
LANDING—STUCK NEUTRAL OR RIGHT PEDAL The landing profile for a stuck neutral or a stuck right pedal is a low power approach or descent with a running or roll-on landing. The approach profile can best be described as a steep approach with a
flare at the bottom to slow the helicopter. The power should be low enough to establish a left yaw during the descent. The left yaw allows a mar-
gin of safety due to the fact that the helicopter will turn to the right when power is applied. This allows the momentary use of power at the bottom of the approach. As you apply power, the helicopter rotates to the right and becomes aligned with the landing area. At this point, roll the throttle to flight idle and make the landing. The momentary use of power helps stop the descent and allows additional time for you to level the helicopter prior to closing the throttle.
If the helicopter is not yawed to the left at the conclusion of the flare, roll the throttle to flight idle and use the collective to cushion the touchdown. As with any running or roll-on landing, use the cyclic to maintain the ground track. This technique results in a longer ground run or roll than if the helicopter was yawed to the left.
UNANTICIPATED YAW / LOSS OF TAIL ROTOR EFFECTIVENESS (LTE)
Unanticipated yaw is the occurrence of an uncommanded yaw rate that does not subside of its own accord and, which, if not corrected, can result in the loss of helicopter control. This uncommanded yaw rate is referred to as loss of tail rotor effectiveness (LTE) and occurs to the right in helicopters with a counter-clockwise rotating main rotor and to the left in helicopters with a clockwise main rotor rotation. Again, this discussion covers a helicopter with a counterclockwise rotor system and an antitorque rotor.
LTE is not related to an equipment or maintenance malfunction and may occur in all single-rotor heli-