
книги студ / color atlas of physiology 5th ed[1]. (a. despopoulos et al, thieme 2003)
.pdf
11 Hormones and Reproduction
288
!
Hypothyroidism occurs when TSH-driven thyroid enlargement is no longer able to compensate for the T3/T4 deficiency (hypothyroid goiter). This type of goiter can also occur due to a congenital disturbance of T3/T4 synthesis (see below) or thyroid inflammation. Hyperthyroidism occurs when a thyroid tumor (hot node) or diffuse struma (e.g., in Grave’s disease) results in the overproduction of T3/T4, independent of TSH. In the latter case, an autoantibody against the TSH receptor binds to the TSH receptor. Its effects mimic those of TSH, i.e., it stimulates T3/T4 synthesis and secretion.
T3/T4 transport. T3 and T4 occur at a ratio of 1 : 40 in the plasma, where !99% of them (mainly T4) are bound to plasma proteins: thy- roxine-binding globulin (TBG), thyroxine-bind- ing prealbumin (TBPA), and serum albumin. TBG transports two-thirds of the T4 in the blood, while TBPA and serum albumin transport the rest. Less than 0.3% of the total T3/T4 in blood occurs in an unbound (free) form, although only the unbound molecules have an effect on the target cells. Certain drugs split T3 and T4 from protein bonds, resulting in increased plasma concentrations of the free hormones.
Potency of T3/T4. T3 is 3–8 times more potent than T4 and acts more rapidly (half-life of T3 is 1 day, that of T4 7 days). Only ca. 20% of all circulating T3 originate from the thyroid; the other 80% are produced by the liver, kidneys, and other target cells that cleave iodide from T4. The conversion of T4 to T3 is catalyzed by microsomal 5!-deiodase, which removes iodine from the 5! position on the outer ring (!D). T3 is therefore the more potent hormone, while T4 is mainly ascribed a storage function in plasma.
The inactive form of T3 called reverse T3 (rT3) is produced from T4 when the iodine is split from the inner ring with the aid of a 5- (not 5!-)deiodase. Approximately equal amounts of T3 and rT3 are normally produced in the periphery (ca. 25 µg/day). When a person fasts, the resulting inhibition of 5!-deiodase decreases T3 synthesis (to save energy, see below) while rT3 synthesis increases. Pituitary 5!-deiodase is not inhibited, so TSH secretion (unwanted in this case) is suppressed by the negative feedback.
T3/T4 receptors are hormone-sensitive transcription factors located in the cell nuclei. Hor- mone–receptor complexes bind to regulator
proteins of certain genes in the nuclei and influence their transcription.
The actions of T3/T4 are numerous and mainly involve the intermediate metabolism. The thyroid hormones increase the number of mitochondria and its cristae, increase Na+-K+- ATPase activity and modulate the cholesterol metabolism. This results in an increase in energy turnover and a corresponding rise in O2 consumption and heat production. T3 also specifically stimulates heat production by increasing the expression of the uncoupling protein thermogenin in brown fat (!p. 222). T3 also influences the efficacy of other hormones. Insulin, glucagon, GH and epinephrine lose their energy turnover-increasing effect in hypothyroidism, whereas the sensitivity to epinephrine increases (heart rate increases, etc.) in hyperthyroidism. T3 is thought to increase the density of !-adrenoceptors. T3 also stimulates growth and maturation, especially of the brain and bones.
Cretinism occurs due to neonatal T3/T4 deficiencies and is marked by growth and maturation disorders (dwarfism, delayed sexual development, etc.) and central nervous disorders (intelligence deficits, seizures, etc.). The administration of thyroid hormones in the first six months of life can prevent or reduce some of these abnormalities.
Iodine metabolism (!D). Iodine circulates in the blood as either (1) inorganic I– (2–10 µg/L),
(2)organic non-hormonal iodine (traces) and
(3)protein-bound iodine (PBI) within T3 and T4 (35–80 µg iodine/L). The average daily requirement of iodine is ca. 150 µg; larger quantities are required in fever and hyperthyroidism (ca. 250–500 µg/day). Iodine excreted from the body must be replaced by the diet (!D). Sea salt, seafood, and cereals grown in iodine-rich soil are rich in iodine. Iodized salt is often used to supplement iodine deficiencies in the diet. Since iodine passes into the breast milk, nursing mothers have a higher daily requirement of iodine (ca. 200 µg/day).
Despopoulos, Color Atlas of Physiology © 2003 Thieme
All rights reserved. Usage subject to terms and conditions of license.
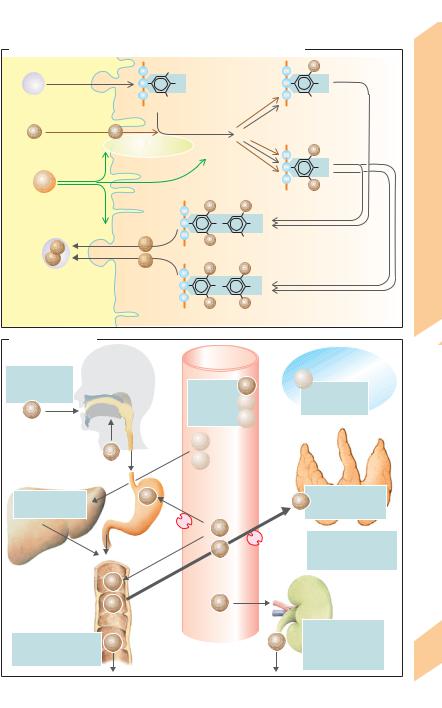
C. Synthesis, storage and mobilization of the thyroid hormones
|
|
|
|
|
|
|
I |
|
Exocytosis |
|
|
OH |
|
|
OH |
|
|
|
|
|
|
||
Thyro- |
|
Tyrosyl residue |
|
|
Monoiodotyrosyl |
||
globulin |
|
|
|
|
|
||
|
|
|
|
Iodination |
|
|
residue (MIT) |
I– |
I0 |
|
|
|
|
||
|
|
|
|
|
|||
|
|
Thyroid peroxidase |
|
|
I |
||
|
|
|
|
|
|
|
|
|
|
|
|
|
|
|
OH |
|
TSH |
|
|
Stored form |
I |
||
|
|
|
|
Diiodotyrosyl |
|||
|
|
|
|
|
|
|
|
|
|
|
|
I |
|
I |
residue (DIT) |
|
|
|
|
3 |
O |
3’ |
|
|
Endocytosis |
|
|
5 |
5’OH |
|
|
|
T3 |
|
T3 |
I |
|
|
|
See B.3 |
|
Triiodothyronyl residue |
Coupling |
||||
|
|
||||||
T4 |
|
T4 |
|||||
|
|
I |
|
I |
|
||
|
|
|
|
|
|
||
|
|
|
|
|
O |
OH |
|
|
|
|
|
I |
|
I |
|
|
Follicular cell |
|
Colloid |
Tetraiodothyronyl residue |
|
||
|
|
|
|
|
|
||
D. Iodine balance |
|
|
|
|
|
|
|
|
|
|
|
Blood |
|
Extracellular |
|
Iodine intake |
|
|
|
|
|
fluid (ECF) |
|
|
|
|
|
|
T4 |
||
150 g/day |
|
|
2–10 g/L |
I– |
|||
|
|
T4 stores |
|||||
(varies greatly) |
|
|
1–2 g/L |
T3 |
|||
I– |
|
|
|
500 g |
|||
|
|
|
35–80 g/L T4 |
|
|||
|
|
|
|
|
|||
|
|
|
|
T3 |
|
|
Thyroid gland |
|
|
I– |
|
|
|
|
|
|
|
|
T4 |
|
|
|
|
|
|
|
|
|
|
|
|
|
10–15 g/day |
|
|
|
|
|
|
T4 glucuronide, |
|
I– |
|
|
|
I– Total iodine content |
|
T3 sulfates |
|
|
|
|
|
5000–7000 g |
|
Liver |
|
|
|
|
I– |
|
|
|
|
|
|
I– |
|
I– loss increases |
|
|
|
|
|
|
|
when breast-feeding |
|
|
|
|
|
|
|
|
(milk contains iodine) |
|
|
I– |
|
|
|
|
|
|
|
I– |
|
|
I– |
|
Kidney |
|
|
|
|
|
|
|
Urinary excretion |
Fecal excretion |
I– |
|
|
|
I– |
(increases with |
|
(increases with diarrhea) |
|
|
|
|
|
proteinuria) |
|
|
10 g/day |
|
|
|
|
|
150 g/day |
Plate 11.12 Thyroid Hormones II
289
Despopoulos, Color Atlas of Physiology © 2003 Thieme
All rights reserved. Usage subject to terms and conditions of license.

11 Hormones and Reproduction
290
Calcium and Phosphate Metabolism
Calcium, particularly ionized calcium (Ca2+), plays a central role in the regulation of numerous cell functions (!pp. 36, 62ff., 192, 276). Calcium accounts for 2% of the body weight. Ca. 99% of the calcium occurs in bone while 1% is dissolved in body fluids. The total calcium conc. in serum is normally 2.1–2.6 mmol/L. Ca. 50% of it is free Ca2+ (1.1–1.3 mmol/L) while ca. 10% is bound in complexes and 40% is bound to proteins (mainly albumin; !p. 178). Calcium protein binding increases as the pH of the blood rises since the number of Ca2+ binding sites on protein molecules also rises with the pH. The Ca2+ conc. accordingly decreases in alkalosis and rises in acidosis (by about 0.21 mmol/L Ca2+ per pH unit). Alkalosis (e.g., due to hyperventilation) and hypocalcemia (see below) can therefore lead to tetany.
The calcium metabolism is tightly regulated to ensure a balanced intake and excretion of Ca2+ (!A). The dietary intake of Ca2+ provides around 12–35 mmol of Ca 2+ each day (1 mmol = 2 mEq = 40 mg). Milk, cheese, eggs and “hard” water are particularly rich in Ca2+. When calcium homeostasis is maintained, most of the ingested Ca2+ is excreted in the feces, while the remainder is excreted in the urine (!p. 178). When a calcium deficiency exists, up to 90% of the ingested Ca2+ is absorbed by the intestinal tract (!A and p. 262).
Pregnant and nursing mothers have higher Ca2+ requirements because they must also supply the fetus or newborn infant with calcium. The fetus receives ca. 625 mmol/day of Ca2+ via the placenta, and nursed infants receive up to 2000 mmol/day via the breast milk. In both cases, the Ca2+ is used for bone formation. Thus, many women develop a Ca2+ deficiency during or after pregnancy.
Phosphate metabolism is closely related to calcium metabolism but is less tightly controlled. The daily intake of phosphate is about 1.4 g; 0.9 g of intake is absorbed and usually excreted by the kidneys (!p. 178). The phosphate concentration in serum normally ranges from 0.8–1.4 mmol/L.
Calcium phosphate salts are sparingly soluble. When the product of Ca2+ conc. times phosphate conc. (solubility product) exceeds a certain threshold, calcium phosphate starts to
precipitate in solutions, and the deposition of calcium phosphate salts occurs. The salts are chiefly deposited in the bone, but can also precipitate in other organs. The infusion of phosphate leads to a decrease in the serum calcium concentration since calcium phosphate accumulates in bone. Conversely, hypophosphatemia leads to hypercalcemia (Ca2+ is released from bone).
Hormonal control. Calcium and phosphate homeostasis is predominantly regulated by parathyroid hormone and calcitriol, but also by calcitonin to a lesser degree. These hormones mainly affect three organs: the intestines, the kidneys and the bone (!B and D).
Parathyrin or parathyroid hormone (PTH) is a peptide hormone (84 AA) secreted by the parathyroid glands. Ca2+ sensors in cells of the parathyroid glands regulate PTH synthesis and secretion in response to changes in the plasma concentration of ionized Ca2+ (!p. 36). More PTH is secreted into the bloodstream whenever the Ca2+ conc. falls below normal (hypocalcemia). Inversely, PTH secretion decreases when the Ca2+ level rises (!D, left panel). The primary function of PTH is to normalize decreased Ca2+ conc. in the blood (!D). This is accomplished as follows: (1) PTH activates osteoclasts, resulting in bone breakdown and the release of Ca2+ (and phosphate) from the bone; (2) PTH accelerates the final step of calcitriol synthesis in the kidney, resulting in increased reabsorption of Ca2+ from the gut; (3) in the kidney, PTH increases calcitriol synthesis and Ca2+ reabsorption, which is particularly important due to the increased Ca2+ supply resulting from actions (1) and (2). PTH also inhibits renal phosphate reabsorption (!p. 178), resulting in hypophosphatemia. This, in turn, stimulates the release of Ca2+ from the bone or prevents the precipitation of calcium phosphate in tissue (solubility product; see above).
Hypocalcemia occurs due to a deficiency (hypoparathyroidism) or lack of efficiency (pseudohypoparathyroidism) of PTH, which can destabilize the resting potential enough to produce muscle spasms and tetany. These deficiencies can also lead to a secondary calcitriol deficiency. An excess of PTH (hyperparathyroidism) and malignant osteolysis overpower the Ca2+ control mechanisms, leading to hypercalcemia. The long-term elevation of Ca2+ results in cal-
Despopoulos, Color Atlas of Physiology © 2003 Thieme |
! |
|
|
All rights reserved. Usage subject to terms and conditions of license. |
|
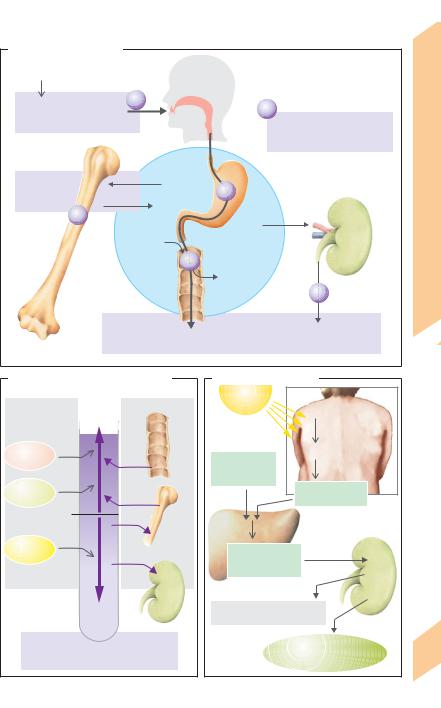
A. Calcium metabolism |
|
|
|
|
Milk, cheese, eggs |
|
|
|
|
and hard water |
|
|
|
I |
Calcium intake |
Ca |
calcium requirements |
Metabolism |
|
20 [12–35] mmol/day* |
|
Ca |
|
|
|
Increased |
|
|
|
|
|
|
|
|
|
|
during pregnancy and nursing |
Phosphate |
|
99% |
|
Ca |
|
|
of total body calcium |
|
|
and |
|
|
|
|
||
Ca |
|
|
|
|
|
|
|
Calcium |
|
|
Approx. |
Extracellular |
Kidney |
|
|
1mmol/day |
|
||
|
fluid |
|
|
|
|
|
|
|
|
|
Ca |
Approx. |
|
|
11.13 |
|
|
|
|
|
||
|
|
|
3mmol/day |
|
|
|
|
|
|
|
|
|
|
Bone |
|
|
|
Ca |
|
Plate |
|
|
|
|
|
||
|
|
|
|
|
|
|
|
Calcium excretion |
in feces |
in urine |
|
||
|
|
18mmol/day |
2mmol/day |
|
||
|
(at an intake of 20 mmol/day) |
|
|
|
|
|
* 1mmol Ca2+ = 2mEq Ca2+ = 40mg Ca2+ |
|
|
|
|
|
|
B. Factors affecting the blood Ca2+ |
C. Calcitriol synthesis |
|
|
|||
concentration |
|
UV light |
|
|
|
|
Hormones |
|
Organs |
|
|
|
|
|
|
7-dehydrocholesterol |
|
|||
|
|
|
|
|
||
|
|
|
|
Previtamin D |
skin |
|
PTH |
Ca2+ |
|
Cholecalciferol |
the |
|
|
Gut |
|
|
||||
|
|
|
(vitamin D3) |
|
In |
|
Raise |
|
|
|
|
|
|
|
|
in the diet |
|
|
|
|
|
|
|
|
|
|
|
Calcitriol |
|
|
|
Cholecalciferol |
|
|
|
|
|
(calciol) |
|
|
|
|
|
|
|
|
|
|
1.25 |
Ca2+ |
|
|
|
|
|
mmol/L |
|
Bone |
Liver |
|
|
|
|
|
|
|
|
||
Lowers |
|
|
|
|
|
|
|
|
|
|
|
|
|
Calcitonin |
|
|
25-OH- |
|
|
|
|
|
|
cholecalciferol |
|
|
|
|
Ca2+ |
|
(calcidiol) |
|
|
|
|
|
|
|
Kidney |
|
|
|
|
|
|
|
|
|
|
|
Kidney |
|
|
|
|
|
|
|
24,25-(OH)2-cholecalciferol |
|
|
|
|
|
|
(inactive form) |
|
|
|
Blood Ca2+ concentration |
|
|
Calcitriol |
|
|
|
|
(1α,25-(OH)2-cholecalciferol |
291 |
||||
|
(ionized) |
|
||||
|
|
|
= active form) |
|
|
Despopoulos, Color Atlas of Physiology © 2003 Thieme
All rights reserved. Usage subject to terms and conditions of license.

!
cium deposition (e.g., in the kidneys). Ca2+ conc. exceeding 3.5 mmol/L lead to coma, renal insufficiency and cardiac arrhythmias.
|
Calcitonin (CT), or thyrocalcitonin, is a peptide |
||||
|
hormone (32). It is mainly synthesized in the |
||||
|
parafollicular cells (C cells) of the thyroid |
||||
|
gland, |
which |
also contain |
Ca2+ sensors |
|
|
(!p. 36). Hypercalcemia increases the plasma |
||||
|
calcitonin conc. (!D, right panel), whereas |
||||
Reproduction |
calcitonin can no longer be detected when the |
||||
PTH). Calcitonin therefore increases the up- |
|||||
|
calcium conc. [Ca2+] falls below 2 mmol/L. Cal- |
||||
|
citonin normalizes elevated serum Ca2+ conc. |
||||
|
mainly by acting on bone. Osteoclast activity is |
||||
|
inhibited by calcitonin (and stimulated by |
||||
and |
take of Ca2+ by the bone—at least temporarily |
||||
(!D5). Some gastrointestinal hormones accel- |
|||||
Hormones |
|||||
fect of calcitonin on digestive activities) func- |
|||||
|
erate calcitonin secretion, thereby enhancing |
||||
|
the postprandial absorption of Ca2+ by bone. |
||||
|
These effects (and perhaps the restraining ef- |
||||
11 |
tion to |
prevent |
postprandial |
hypercalcemia |
|
and the (unwanted) inhibition of PTH secre- |
|||||
|
tion and increased renal excretion of the just absorbed Ca2+. Calcitonin also acts on the kidneys (!D6).
Calcitriol (1,25-(OH)2-cholecalciferol) is a lipophilic, steroid-like hormone synthesized as follows (! C): Cholecalciferol (vitamin D3) is produced from hepatic 7-dehydrocholesterol in the skin via an intermediate product (previtamin D) in response to UV light (sun, tanning lamps). Both substances bind to vitamin D-binding protein (DBP) in the blood, but cholecalciferol is preferentially transported because of its higher affinity. Previtamin D therefore remains in the skin for a while after UV light exposure (short-term storage). Calcidiol (25-OH-cholecalciferol) and calcitriol bind to DBP. An estrogen-dependent rise in DBP synthesis occurs during pregnancy.
Cholecalciferol (vitamin D3) is administered to compensate for inadequate UV exposure. The recommended daily dosage in children is approximately 400 units = 10 µg; adults receive half this amount. Plant-derived ergocalciferol (vitamin D2) is equally effective as animal-derived vitamin D3. The following actions apply for both forms.
Cholecalciferol is converted to calcidiol (25- 292 OH-cholecalciferol) in the liver. Vitamin D is mainly stored as calcidiol because the plasma
conc. of calcidiol is 25 µg/L, and its half-life is 15 days. Calcitriol (1,25-(OH)2-cholecalciferol), the hormonally active form, is mainly synthesized in the kidneys (!C), but also in the placenta. The plasma conc. of calcitriol is regulated by renal 1-α-hydroxylase (final step of synthesis) and by 24-hydroxylase, an enzyme that deactivates calcitriol.
The calcitriol concentration rises in response to hy- pocalcemia-related PTA secretion (!D2), to phosphate deficiency and to prolactin (lactation). All three inhibit 24-hydroxylase and activate 1-α-hy- droxylase. It decreases due to several negative feedback loops, i.e. due to the fact that calcitriol (a) directly inhibits 1-α-hydroxylase, (b) inhibits parathyroid hormone secretion, and (c) normalizes the (decreased) plasma conc. of Ca2+ and phosphate by increasing the intestinal absorption of Ca2+ and phosphate (see below). Calcium and phosphate inhibit 1-α-hydroxylase, while phosphate activates 24hydroxylase.
Target organs. Calcitriol’s primary target is the gut, but it also acts on the bone, kidneys, placenta, mammary glands, hair follicles, skin etc. It binds with its nuclear receptor and induces the expression of calcium-binding protein and Ca2+-ATPase (! pp. 278 and 36). Calcitriol has also genomic effects. Calcitriol increases the intestinal absorption of Ca2+ (!D4) and promotes mineralization of the bone, but an excess of calcitriol leads to decalcification of the bone, an effect heightened by PTH. Calcitriol also increases the transport of Ca2+ and phosphate at the kidney (!p. 178), placenta and mammary glands.
In transitory hypocalcemia, the bones act as a temporary Ca2+ buffer (!D) until the Ca2+ deficit has been balanced by a calcitriol-mediated increase in Ca2+ absorption from the gut. If too little calcitriol is available, skeletal demineralization will lead to osteomalacia in adults and rickets in children. Vitamin D deficiencies are caused by inadequate dietary intake, reduced absorption (fat maldigestion), insufficient UV light exposure, and/or reduced 1-α- hydroxylation (renal insufficiency). Skeletal demineralization mostly occurs due to the prolonged increase in parathyroid hormone secretion associated with chronic hypocalcemia (compensatory hyperparathyroidism).
Despopoulos, Color Atlas of Physiology © 2003 Thieme
All rights reserved. Usage subject to terms and conditions of license.
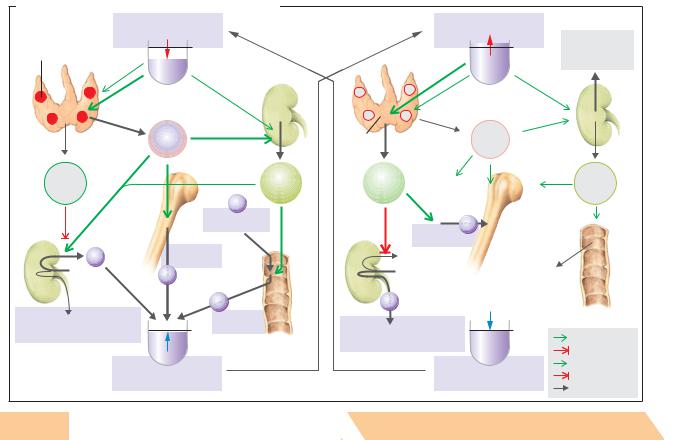
Usage .reserved rights All |
Atlas Color Despopoulos, |
D. Hormonal regulation of the blood Ca2+ concentration |
|
|
|
|
|
||||
|
|
Serum Ca2+ (ionized) |
|
|
Serum Ca2+ (ionized) |
|
|||||
|
|
falls below normal |
|
|
rises above normal |
Inactive |
|||||
Parathyroid glands |
1.25 mmol/L* |
|
|
|
|
|
|||||
|
|
|
|
|
24,25-(OH)2- |
||||||
(epithelial bodies) |
|
|
|
|
|
|
|
cholecaciferol |
|||
|
|
Ca2+ |
|
|
|
|
|
Ca2+ |
? |
||
|
|
|
Kidney |
|
|
|
|
|
|||
|
|
|
2 |
|
|
|
|
|
|
||
terms to subject |
© Physiology of |
|
|
PTH |
|
Thyroid |
|
|
PTH |
|
|
|
|
|
|
|
|
|
|||||
|
|
|
|
|
gland |
|
|
|
|
||
|
|
|
|
|
(C cells) |
|
|
|
|
||
Calcitonin |
|
|
|
Calcitriol |
Calcitonin |
|
|
|
Calcitriol |
||
|
|
1 |
Ca2+ |
|
5 |
|
|
|
|
||
conditions and |
Thieme 2003 |
|
|
|
|
|
|
|
|||
|
|
Bone |
Dietary Ca2+ |
|
|
Ca |
2+ |
Bone |
|
||
Kidney |
3 |
|
|
Storage of |
|
||||||
|
|
|
|
|
|||||||
|
|
|
|
|
Ca2+ |
|
|
|
|
||
|
Ca2+ |
Demineral- |
|
|
|
|
|
|
|||
|
ization |
Gut |
|
|
|
|
|
|
|||
|
|
Ca2+ |
4 |
|
6 |
|
|
|
Decreased |
||
|
|
|
|
|
|
|
|
||||
of |
|
|
|
|
|
|
|
Ca2+ absorption |
|||
.license |
|
|
|
|
Ca2+ |
|
Ca2+ |
|
|
|
|
|
Decreased Ca2+ excretion |
|
Increased |
|
|
|
|
|
|
||
|
|
(Increased PO4– excretion) |
|
absorption |
|
Increased Ca2+ excretion |
|
|
|
Stimulates |
|
|
|
|
|
|
|
|
(Decreased PO4– excretion) |
|
|
|
|
|
|
|
|
|
|
|
|
|
|
|
Inhibits |
|
|
|
|
|
|
|
|
|
|
|
No stimulation |
|
|
|
|
Serum Ca2+ (ionized) |
|
|
Serum Ca2+ (ionized) |
No inhibition |
|||
|
|
|
|
returns to normal |
|
* 1mmol Ca2+ = 2mEq Ca2+ = 40mg Ca2+ |
returns to normal |
Secretion, absorption |
293
Plate 11.14 Calcium and Phosphate Metabolism II

11 Hormones and Reproduction
294
Biosynthesis of Steroid Hormones
Cholesterol is the precursor of steroid hormones (!A). Cholesterol is mainly synthesized in the liver. It arises from acetylcoenzyme A (acetyl-CoA) via a number of intermediates (e.g., squalene, lanosterol) and is transported to the endocrine glands by lipoproteins (!p. 256). Cholesterol can be synthesized de novo also in the adrenal cortex, but not in the placenta (!p. 304). Since only small quantities of steroid hormones are stored in the organs of origin, i.e., the adrenal cortex, ovaries, testes and placenta (!p. 304), they must be synthesized from the cellular cholesterol pool as needed.
Cholesterol contains 27 carbon atoms. Pregnenolone (21 C atoms; !A, a), the precursor of steroid hormones, arises from cholesterol via numerous intermediates. Pregnenolone also yields progesterone (!A, b), which is not only a potent hormone itself (female sex hormone; !p. 298ff.), but can act as the precursor of all other steroid hormones, i.e., (1) the adrenocortical hormones with 21 carbon atoms (!A, yellow and orange fields); (2) male sex hormones (androgens, 19 carbon atoms) synthesized in the testes (!p. 306), ovaries and adrenal cortex (!A, green and blue fields); and (3) female sex hormones (estrogens, 18 carbon atoms; !p. 29 B ff.) synthesized in the ovaries (!A, red zones).
The precursors for steroid hormone synthesis are present in all steroid hormone glands. The type of hormone produced and the site of hormone synthesis depend on (1) the type of receptors available for the superordinate control hormones (ACTH, FSH, LH, etc.) and (2) the dominant enzyme responsible for changing the structure of the steroid molecule in the hormone-producing cells of the gland in question. The adrenal cortex contains 11-, 17and 21-hydroxylases—enzymes that introduce an OH group at position C21, C17 or C11, respectively, of the steroid molecule (!A, top left panel for numerical order). Hydroxylation at C21 (!A, c)—as realized in the glomerular zone of the adrenal cortex—makes the steroid insensitive to the effects of 17-hydroxylase. As a result, only mineralocorticoids like corticosterone and aldosterone (A, d ! e; see also
p. 182) can be synthesized. Initial hydroxylation at C17 (! A, f or g) results in the synthesis of glucocorticoids—realized mainly in the fascicular zone of the adrenal cortex (!A, h ! j ! k)—and 17-ketosteroids, steroids with a keto group at C17 (!A, l and m). Glucocorticoids and 17-ketosteroids can therefore be synthesized from 17α-hydroxypregnenolone without the aid of progesterone (!A, n ! h ! j).
The estrogens (!p. 302) estrone and estradiol can be directly or indirectly synthesized from 17-ketosteroids (!A, o ! p); they are produced indirectly by way of testosterone (!A, q ! r ! p). The true active substance of certain target cells for androgens (e.g., in the prostate) is either dihydrotestosterone or estradiol ; both are synthesized from testosterone (!A,s and A,r, respectively).
17-ketosteroids are synthesized by the gonads (testes and ovaries) and adrenal cortex. Since they are found in the urine, the metyrapone test of pituitary function is used to assess the ACTH reserve based on urinary 17-ketosteroids levels. ACTH secretion is normally subject to feedback control by glucocorticoids (!p. 296). Metyrapone inhibits 11-hydroxy- lase activity (!A, d and j), which leaves ACTH unsuppressed in healthy subjects. Urinary 17-ke- tosteroid levels should therefore increase after metyrapone administration. An abnormality of ACTH secretion can be assumed when this does not occur in patients with a healthy adrenal cortex.
Degradation of steroid hormones occurs mainly in the liver. Their OH groups are usually linked to sulfate or glucuronic acid molecules and are ultimately excreted in the bile or urine (!pp. 160, 183 and 250). The chief urinary metabolite of the estrogens is estriol, while that of the gestagens (mainly progesterone and 17α-hydroxyprogesterone) is pregnanediol (! p. 304). Pregnanediol levels in urine can be measured to confirm or exclude pregnancy test (pregnanediol test). Chronically increased estrogen levels due, for example, to decreased estrogen degradation secondary to liver damage, can lead to breast development (gynecomastia) in the male, among other things. For normal estrogen ranges, see table on p. 302.
Despopoulos, Color Atlas of Physiology © 2003 Thieme
All rights reserved. Usage subject to terms and conditions of license.
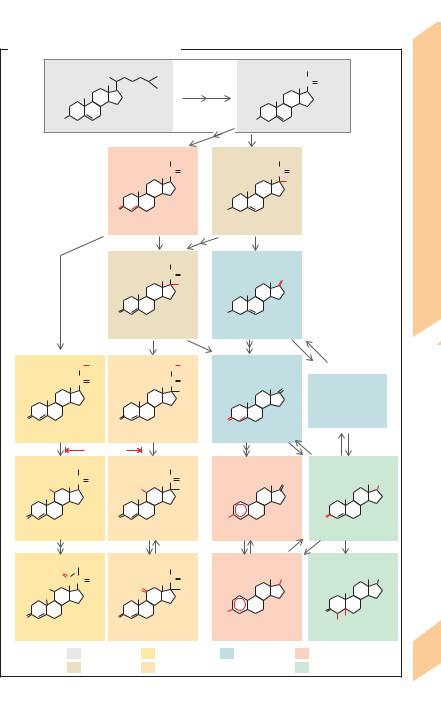
A. Biosynthesis of steroid hormones |
|
|
|
|
|
|
|
|||||||||||
|
C27 |
|
|
|
|
|
21 |
22 |
24 |
|
Precursors |
C21 |
|
CH3 |
|
|||
|
|
|
|
|
|
26 |
|
|
|
C |
O |
|
||||||
|
|
|
|
|
12 |
18 |
20 23 |
|
|
|
|
|
|
|||||
|
|
|
|
11 |
25 |
27 |
|
a |
|
|
|
|||||||
|
|
|
|
|
|
|
17 |
|
|
|
|
|
|
Hormones |
||||
|
2 |
|
1 19 |
9 |
|
13 |
16 |
|
|
|
|
|
|
|
|
|
||
|
|
|
814 15 |
|
|
|
|
|
|
|
|
|
||||||
|
3 |
10 |
|
|
|
|
|
|
|
|
|
|
||||||
|
|
|
|
|
|
|
|
|
|
|
|
|||||||
|
HO |
4 5 |
|
|
7 |
|
|
|
|
|
|
HO |
|
|
|
|||
|
|
|
|
|
|
|
|
|
|
Pregnenolone |
||||||||
|
|
6 |
|
|
Cholesterol |
|
|
|
||||||||||
|
|
|
|
|
|
|
|
|
|
|
|
|
b |
g |
|
|
|
|
|
|
|
|
|
|
|
|
|
|
|
|
|
|
|
|
of Steroid |
||
|
|
|
|
|
|
|
|
|
|
|
|
|
|
|
|
|
||
|
|
|
|
|
|
|
C21 |
|
|
CH3 |
|
C21 |
CH3 |
|
|
|||
|
|
|
|
|
|
|
|
|
|
|
C |
O |
|
C |
O |
|
|
|
|
|
|
|
|
|
|
|
|
|
|
|
|
|
17 |
OH |
|
|
|
|
|
|
|
|
|
|
|
3 |
|
|
|
|
|
|
|
|
|
Biosynthesis |
|
|
|
|
|
|
|
O |
|
|
|
|
|
HO |
17α-OH- |
|
|
||
|
|
|
|
|
|
|
|
|
Progesterone |
|
|
|
||||||
|
|
|
|
|
|
|
|
|
|
pregnenolone |
|
|
||||||
|
|
|
|
|
|
|
|
|
|
|
|
|
|
|
|
|||
|
|
|
|
|
|
|
|
|
|
f |
|
n |
|
m |
|
|
|
|
|
|
|
|
|
|
|
|
|
|
|
|
|
|
|
|
|
|
|
|
|
|
|
|
|
|
C21 |
|
|
CH3 |
|
C19 |
|
|
|
|
11.15 |
|
|
|
|
|
|
|
|
|
|
|
|
C |
O |
|
O |
|
|
||
|
c |
|
|
|
|
|
|
|
|
|
17 |
OH |
|
17 |
|
|
|
|
|
|
|
|
|
|
|
|
|
|
|
|
|
|
Plate |
||||
|
|
|
|
|
|
|
|
|
|
|
|
|
|
|
||||
|
|
|
|
|
|
|
O |
|
|
|
|
|
HO |
|
|
|
|
|
|
|
|
|
|
|
|
|
|
|
17α-OH- |
Dehydroepi- |
|
|
|
||||
|
|
|
|
|
|
|
|
|
|
|
|
|
|
|
||||
|
|
|
|
|
|
|
|
|
progesterone |
androsterone (DHEA) |
|
|
|
|||||
|
|
|
|
|
|
|
|
|
h |
|
l |
|
|
|
|
|
|
|
C21 |
|
H2C |
OH |
C21 |
|
|
H2C |
OH |
C19 |
|
|
|
|
|
||||
|
|
|
C |
O |
|
|
|
|
|
C |
O |
|
|
O |
|
|
|
|
|
|
|
|
|
|
|
|
|
|
|
|
OH |
|
|
|
|
|
|
|
|
|
|
|
|
|
|
|
|
|
|
|
|
|
|
Other |
|
|
|
|
|
|
|
|
|
|
|
|
|
|
|
|
|
|
|
|
|
|
|
|
|
|
|
|
|
|
|
|
|
|
|
3 |
|
|
17-ketosteroids |
|
O |
|
|
|
|
|
|
O |
|
|
|
|
|
O |
|
|
|
|
|
11-desoxy- |
|
|
|
|
|
|
|
|
|
|
|
|||||||
|
|
|
11-desoxycortisol |
|
Androstenedione |
|
|
|
||||||||||
corticosterone |
|
|
|
|
|
|
||||||||||||
|
|
|
|
|
|
|
|
|
|
|
|
|
||||||
|
d |
|
Metopyrone |
|
j |
|
|
|
o |
q |
|
|
|
|||||
C21 |
|
|
CH2OH |
|
C21 |
|
|
CH2OH |
C18 |
|
|
|
C19 |
|
||||
HO |
|
|
C |
O |
|
|
|
|
HO |
|
C |
O |
|
|
O |
|
OH |
|
11 |
|
|
|
|
|
|
|
11 |
|
OH |
|
|
|
|
17 |
|
||
|
|
|
|
|
|
|
|
|
|
|
|
|
|
|
|
|||
O |
|
|
|
|
|
|
O |
|
|
|
|
HO |
|
|
|
O |
|
|
Corticosterone |
|
|
|
|
|
Cortisol |
|
Estron (E1) |
|
Testosterone |
|
|||||||
|
e |
|
|
|
|
|
|
|
k |
|
|
|
p |
|
r |
s |
|
|
C21 |
O |
|
CH2OH |
|
C21 |
|
|
CH2OH |
C18 |
|
|
|
C19 |
|
||||
|
H |
|
|
|
|
|
|
|
C |
O |
|
OH |
|
OH |
|
|||
|
|
C C |
O |
|
|
|
|
|
|
|
|
|
||||||
HO |
|
|
|
|
|
|
|
|
|
|
|
|
|
|||||
18 |
|
|
|
|
|
|
O |
11 |
|
OH |
|
|
|
|
|
|
||
|
|
|
|
|
|
|
|
|
|
|
|
|
|
|
|
|||
O |
|
|
|
|
|
|
O |
|
|
|
|
|
HO |
|
|
|
O |
|
|
|
|
|
|
|
|
|
|
|
|
|
|
|
|
H2 H |
|
||
|
Aldosterone |
|
|
|
|
Cortisone |
|
Estradiol (E2) |
|
Dihydrotestosterone |
|
|||||||
|
|
|
Precursors |
|
|
|
Mineralocorticoids |
17-ketosteroids |
|
Female sex hormones |
295 |
|||||||
|
|
|
Intermediates |
|
|
Glucocorticoids |
|
|
|
|
Male sex hormones |
|
Despopoulos, Color Atlas of Physiology © 2003 Thieme
All rights reserved. Usage subject to terms and conditions of license.

Adrenal Cortex and Glucocorticoid
Synthesis
|
The mineralocortico(stero)ids |
aldosterone, |
|
|
corticosterone and 11-desoxycorticosterone |
||
|
(! pp. 182ff. and 294) are synthesized in the |
||
|
glomerular zone of the adrenal cortex (! A1), |
||
|
whereas the glucocortico(stero)ids cortisol |
||
|
(hydrocortisone) and cortisone |
(! p. 294, |
|
Reproduction |
small quantities) are synthesized in the fascic- |
||
ular zone (! A2). Androgens are synthesized |
|||
|
|||
|
in the reticular zone of the adrenal cortex (! |
||
|
A3). One of the androgens is dehydroepian- |
||
|
drosterone (DHEA), which is used (partly in its |
||
|
sulfated form, DHEA-S) to synthesize various |
||
and |
sex hormones in other tissues (! p. 304). |
||
Cortisol transport. Most of the plasma corti- |
|||
Hormones |
sol is bound to transcortin, or cortisol-binding |
||
|
|||
|
globulin (CBG), a specific transport protein |
||
|
with a high-affinity binding site for cortisol. |
||
|
Cortisol is released in response to confor- |
||
11 |
mational changes of CBG due to inflammation |
etc.
CRH and ACTH regulate cortisol synthesis and secretion (! A4, A5; see also p. 270). ACTH ensures also structural preservation of the adrenal cortex and supplies cortisol precursors, e.g., by forming cholesterol from its esters, by de novo synthesis of cholesterol and by converting it to progesterone and 17α-hy- droxyprogesterone (!pp. 256 and 294). ACTH secretion is stimulated by CRH and epinephrine and inhibited (negative feedback control) by cortisol with or without the aid of CRH (!A; see also p. 273 A).
A circadian rhythm of CRH secretion and thus of ACTH and cortisol secretion can be observed. The peak secretion is in the morning (!B, mean values). Continuous hormone conc. sampling at short intervals have shown that ACTH and cortisol are secreted in 2–3-hour episodes (!B).
Receptor proteins (!p. 278) for glucocorticoids can be found in virtually every cell. Glucocorticoids are vital hormones that exert numerous effects, the most important of which are listed below.
Carbohydrate and amino acid (AA) metabolism (see also pp. 283 A and 285 C): Cortisol uses AA derived from protein degradation to
296increase the plasma glucose concentration (gluconeogenesis), which can lead to the so-
called steroid diabetes in extreme cases. Thus, cortisol has a catabolic effect (degrades proteins) that results in the increased excretion of urea.
Cardiovascular function: Glucocorticoids increase myocardial contractility and vasoconstriction due to enhancement of catecholamine effects (!pp. 194 and 214). These are described as permissive effects of cortisol. Cortisol increases the synthesis of epinephrine in the adrenal medulla (!A6) and of angiotensinogen in the liver (!p. 184).
Especially when administered at high doses, glucocorticoids induce anti-inflam- matory and anti-allergic effects because they stabilize lymphokine synthesis and histamine release (!p. 100). On the other hand, inter- leukin-1, interleukin-2 and TNF-α (e.g., in severe infection) leads to increased secretion of CRH and high cortisol conc. (see below).
Renal function: Glucocorticoids delay the excretion of water and help to maintain a normal glomerular filtration rate. They can react also with aldosterone receptors but are converted to cortisone by 11!-hy- droxysteroid oxidoreductase in aldosterone target cells. Normal cortisol conc. are therefore ineffective at the aldosterone receptor. High conc., however, have the same effect as aldosterone (!p. 182).
Gastric function: Glucocorticoids weaken the protective mechanisms of the gastric mucosa. Thus, high-dose glucocorticoids or stress (see below) increase the risk of gastric ulcers (!p. 242).
Cerebral function: High glucocorticoid conc. change hypothalamic (!A) and electrical brain activity (EEG) and lead to psychic abnormalities.
Stress: Physical or mental stress increases cortisol secretion as a result of increased CRH secretion and increased sympathetic tone (!A). Many of the aforementioned effects of cortisol therefore play a role in the body’s response to stress (activation of energy metabolism, increase in cardiac performance, etc.). In severe physical (e.g., sepsis) or mental stress (e.g., depression), the cortisol plasma conc. remains at a very high level (up to 10 times the normal value) throughout the day.
Despopoulos, Color Atlas of Physiology © 2003 Thieme
All rights reserved. Usage subject to terms and conditions of license.
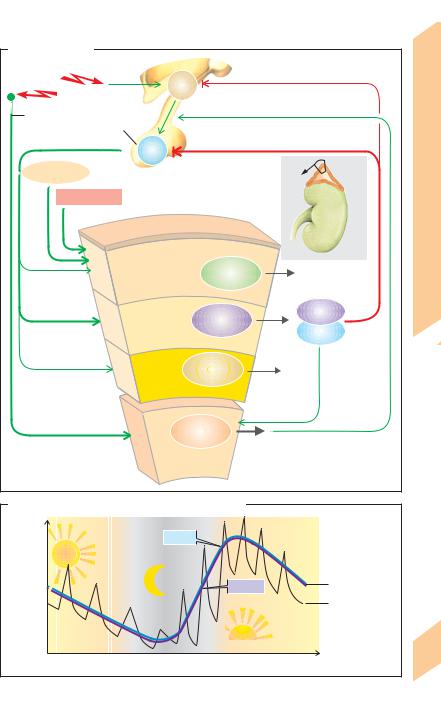
A. Adrenal gland |
|
|
|
|
Synthesis |
|
|
|
|
|
|
|
|
|
Hypothalamus |
|
|
Stress |
|
|
CRH |
Negative feedback |
|
|
|
5 |
Glucocorticoid |
||
|
|
|
|
||
|
|
|
|
|
|
Sympathetic |
Anterior |
4 |
|
Epinephrine stimulates |
|
|
|
|
|||
nervous system |
pituitary |
|
ACTH release |
|
|
|
|
|
|||
|
|
ACTH |
|
|
|
Angiotensin II |
|
|
|
Adrenal |
and |
|
|
|
gland |
||
Hyperkalemia |
|
|
Kidney |
Cortex |
|
|
|
|
|
||
|
|
|
|
|
Adrenal |
|
|
|
Mineralo- |
|
|
|
|
|
11.16 |
|
|
|
|
corticoids |
|
Aldosterone |
|
|
|
||
|
|
|
|
|
|
|
|
|
||
|
|
|
merular zone |
|
|
1 |
|
|||
|
|
|
|
|
|
Plate |
||||
|
Adre |
Glo |
|
|
|
|
|
|
||
|
|
|
|
|
|
|
Cortisol |
|||
|
nal |
|
Gluco- |
|
|
|
||||
|
|
|
Cortisol |
|
|
|||||
|
|
|
|
|
|
|
||||
|
c |
|
corticoids |
|
|
|
|
|||
|
ortex |
|
|
|
Transcortin |
|
||||
|
|
|
|
one |
|
2 |
|
|||
|
|
|
|
ular z |
|
|
|
|
|
|
|
|
|
scic |
|
|
|
|
|
|
|
|
|
Fa |
|
|
|
|
|
within the adrenal gland |
|
|
|
|
|
|
|
|
Anabolic |
|
Blood flow path |
|
|
|
|
|
|
|
|
steroids and |
|
|
||
|
|
|
|
|
sex hormones |
3 |
|
|||
|
|
|
|
|
r zone |
|
||||
|
|
|
|
|
|
|
||||
|
|
|
|
cula |
|
|
|
|||
|
|
|
Reti |
|
|
|
|
|||
|
|
A |
|
|
6 |
|
|
|||
|
|
d |
|
|
|
|
||||
|
|
|
|
|
|
|
|
|||
|
|
r |
|
|
|
|
|
|
||
|
|
ena |
|
Epinephrine |
|
|
|
|
||
|
|
|
Norepinephrine |
|
|
|
|
|||
|
|
|
l |
|
|
|
|
|
||
|
|
|
me |
|
|
|
|
|
||
|
|
|
|
|
|
|
|
|
|
|
|
|
|
d |
|
|
|
|
|
|
|
|
|
|
ulla |
|
|
|
|
|
|
|
B. Circadian rhythm of ACTH and cortisol secretion |
|
|
|
|
||||||
|
|
|
|
ACTH |
|
|
|
|
|
|
concentration |
|
|
|
|
|
Cortisol |
|
Mean |
|
|
|
|
|
|
|
|
|
|
|||
|
|
|
|
|
|
|
|
Short-term |
|
|
Plasma |
|
|
|
|
|
|
|
|
|
|
|
|
|
|
|
|
|
|
fluctuations |
|
|
|
|
|
|
|
|
|
|
|
|
|
12:00p.m. |
6:00p.m. |
|
12:00a.m. |
6:00a.m. |
|
12:00p.m. Time of day |
297 |
|||
|
|
|
Despopoulos, Color Atlas of Physiology © 2003 Thieme
All rights reserved. Usage subject to terms and conditions of license.