
Mechanical Properties of Ceramics and Composites
.pdf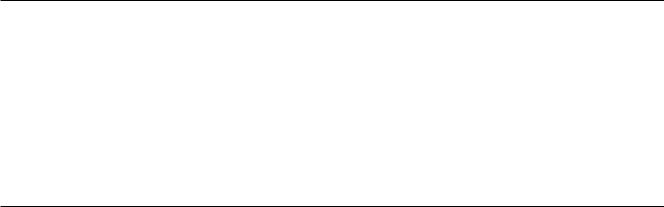
480
Table 8.2 Comparison of Mechanical Properties of Some SiC Whisker Composites with Different Matricesa
Matrix |
E (GPa) |
E (%) |
K (MPam1/2) |
K (%) |
σ (MPa) |
Δσ (%) |
Investigator |
|||
|
|
|
|
|
|
|
|
|
|
|
Cordie- |
— |
— |
1.25 |
330 |
|
85 |
|
365 |
|
Gadkaree [171] |
rite |
140 |
35 |
1.9 |
74 |
|
170 |
|
41 |
|
Wadsworth and Stevens [58] |
|
90 |
50 |
2.0 |
115 |
|
220 |
|
86 |
|
Ashizuka et al. [57] |
Mullite |
|
|
2.7 |
160 |
(85) |
320 |
|
190 |
(122) |
Wu et al. [172] |
|
210 |
— |
2.0 |
40 |
|
440 |
|
31 |
|
Kumazawa [60] |
|
|
|
2.2 |
105 |
|
— |
|
— |
|
Becher et al. [163] |
Si3N4 |
335 |
1–2 |
5.5 (7.1) |
45 |
(46) |
400 |
(650) |
–25 (–30) |
Shalek et al. [63] |
|
|
|
(φ=0.2) |
6 |
–18 |
|
900 |
|
–38 |
|
Lundberg et al. [173] |
|
|
|
3.7 (4.7) |
27 |
(36) |
780 |
|
26 |
|
Buljan et al. [174] |
Al2O3 |
395 |
3 |
4.5 |
27–56 |
560 |
|
18 |
|
Yang and Stevens [59] |
|
|
|
|
4.2 |
107 |
|
300 |
|
120 |
|
Becher et al. [163] |
|
|
|
4.0 |
75 |
|
460 |
|
60 |
|
Iio et al. [175] |
= the incremental change (in %), i.e if a value doubles its incremental change is 100%. Note some decreases in properties. All changes are for φ=0.3, except data of Lundberg et al. for φ=0.2.
8 Chapter
Particle (and Grain) Effects |
481 |
whisker content in a Si3N4 matrix for different hot pressing conditions, showing differences of >10% with no whiskers. For the two hot pressing temperatures with the most data, one first decreased and one steadily increased, with both reaching maxima at φ=0.2, one at the E value for φ=0 and the other nearly 15% above the φ=0 value. Desmarres et al. [64] reported that addition of 30 v/o SiC whiskers to Si3N4 resulted in very little anisotropy, i.e. E was 348 and 340 GPa parallel respectively to the hot pressing axis and the plane of pressing, which represented an 20% increase over the Si3N4 alone (280 GPa).
While composite moduli values can often be reasonably estimated from rule of mixtures, or more precise, bounds, there are various problems that can arise and limit the utility of more precise models. A basic problem is whether some, especially unknown, microcracking has occurred, e.g. as noted earlier for Al2O3-ZrO2. Another important factor can be the degree of preferred orientation, especially of morphologically shaped single crystal dispersions such as whiskers or platelets, since these introduce significant (not always well documented) crystalline anisotropies and often incompletely characterized degrees and character of preferred orientation. Other issues in the measuring, and especially the estimation, of elastic moduli are the degrees of reaction and bonding between the matrix and the dispersed phase, accurate data for the properties of the phases involved, and the amount and character of any porosity in the bodies.
IV. PARTICLE (AND GRAIN) PARAMETER EFFECTS ON CRACK PROPAGATION
Of the four aspects of crack propagation of fracture mode, i.e. interor transgranular fracture (IGF or TGF), slow crack growth (SCG) due to environmental effects, more macro behavior such as crack branching, and fracture energy/toughness, only the latter has received substantial attention, which is discussed in the next section. This section addresses the limited information on the first three noted aspects of crack propagation.
The limited data on fracture mode in particulate and related composites is summarized here from the review by Rice [65] based on his own and literature observations. He noted that there was considerable TGF in many particulate and whisker composites fractured at nominally 22°C, with much of this in the matrix (commonly Al2O3, G 1–10 m), but also frequently of the dispersed phase. Thus laboratory and commercial Al2O3-TiC (G 2–5 m, strength >700 MPa) showed >50% TGF, which is consistent with Krell and Blank’s [66] observation of considerable TGF in their Al2O3-TiC. While the ready cleavage of some dispersed phases such as TiC may be a factor in the often substantial TGF of the Al2O3 matrix in these composites, there seems to be a broader effect, since increased TGF is seen in other composites with less distinct cleavage of the dispersed phase. Thus Rice reports that Al2O3-ZrO2 bodies (G 2–5 m, strength 700 MPa)

482 |
Chapter 8 |
commonly showed 10–30% or more TGF, i.e. similar to, possibly more than in, bodies of either phase alone with the same G along with similar results of hot pressed ThO2 (3 wt% Y2O3) with 25 m/o ZrO2 (again with some reduction in grain size). Further, there clearly also was substantial TGF in the Al2O3 matrix with SiC whiskers, which frequently fractured both transversely and along the Al2O3-SiC interface (Fig. 8.14). While the TGF preferentially occurred in the larger grains on the fracture surface, as in monolithic ceramics, the degree of TGF in the composite matrix is typically higher than in the matrix alone (of the same G). This was clearly shown by the work of Baek and Kim [67] where the matrix alone (G 6m) had predominantly IGF, but with 20% SiC whiskers the matrix (with the same G) had mainly TGF. Dauskardt et al. [68] also reported essentially 100% TGF in the Al2O3 matrix (G 2 m) of Al2O3-SiC whisker composites fractured in fatigue tests.
FIGURE 8.14 Flexure fracture of commercial (Cercom) Al2O3-SiC whisker composite at 22°C. Note substantial TGF in the Al2O3 and considerable transverse fracture of the whiskers, as well as considerable interfacial fracture (i.e. IGF) around part of many SiC whiskers.
Particle (and Grain) Effects |
483 |
WC-Co composites, which have been extensively studied, also show substantial TGF. Thus earlier flexure strength studies of Gurland and Bardzil [69] showed 50–75% TGF of WC grains for G 1–4 m, and while Murray [70] showed 10% for similar G material in KIC tests, Hara et al. [71] reported a transition from IGF to TGF as G increased. Pickens [72], Pickens and Gurland [73], and Roebuck and Almond [74] concluded from their own studies and surveys of WC-Co data that TGF increases with G and the amount of Co. Pickens was more quantitative, indicating more IGF for G < 2 m and more TGF for G ≥ 2 m; he noted more TGF of larger grains in a given body (which thus can vary TGF with G distribution) and cited possible effects of crack velocity and of G measurements. Comparison with the one data point for pure WC [75] suggests that WCCo has more TGF at comparable G. An important factor in the fracture mode of such WC-Co composites is the degree of contiguity of the phases, with increased contiguity of the WC phase enhancing TGF of the WC [76].
The overall fracture mode provides some important indications about the fracture process, e.g. transgranular fracture of the dispersed phase implies less effect of crack bridging, since this is typically enhanced by intergranular fracture. However, other information is typically needed in conjunction with fracture mode, such as particle sizes, spacings, agglomeration, etc., important both generally, e.g. for effects on toughening mechanisms, and locally for effects on failure initiation. Such information is typically lacking, as is that on another factor that can be important, namely the effectiveness of the second phase in interacting with the crack, e.g. since, as illustrated in Fig. 8.8, limited crack variations can reduce crack–second phase particle interactions significantly. Though almost totally neglected, such interactions can vary as a function of a number of material and fracture parameters, e.g. crack velocity.
There has been limited study of SCG in ceramic composites, despite the important role that such crack growth can play in monolithic ceramics and the possible contribution that composite stresses between phases may contribute to such growth, at least on a local basis. From results of nominally single phase ceramics, composites with all phases susceptible to SCG are likely to have SCG, but affected by the microstructure and related stresses, and composites of phases having no SCG would have none (provided no grain boundary effects override such exclusion). The extent of SCG in composites having at least one phase susceptible to SCG probably depends on both the extent and the variation of contiguity of the SCG susceptible phase (e.g. as indicated in SiC and Si3N4 with oxide containing grain boundary phases, Chap. 2, Sec. III.B). However, there are a few studies of ceramic composites with oxide matrices susceptible to SCG that show that the composites can have significantly reduced SCG.
One set of materials on which there has been some SCG study are those composites that have a silicate glass matrix. Carlström et al. [77] reported that
484 |
Chapter 8 |
dynamic fatigue tests of four alumina-containing electrical porcelains gave n values of 26–35 and 31–48 at two different loading rate ranges. The ranges of these values thus encompass the n values that Soma et al. [78] measured on an electrical porcelain containing crystallites of mullite, quartz, and alumina. Smyth and Magida [79] using dynamic fatigue found n 30 for a commercial crystallized glass (MACOR ). Cook et al. [80] reported n values of 24–27 for three different bodies crystallized from LiO2-SiO2 glasses.
Jessen and Lewis [81] showed that composites of 1–10 v/o dispersed spherical particles of a Fe-Ni-Co alloy ( 44–75 m dia.) hot pressed in a borosilicate sealing glass of matching thermal expansion exhibited SCG, i.e. n values of 30 similar to the glass matrix itself (see Sec. 3 for elastic properties [34]). However, the stress intensities required for equivalent crack velocities in the composite as in the glass alone were increased by 50%, so there was some reduction in the net SCG for a given stress intensity in the composite versus the glass alone. However, tests of composites made with 2.5 to 10 v/o preoxidized particles (giving an oxide layer 1–2 m thick; the above original composites were made without any oxidation of the alloy particles) greatly increased the n values, e.g. to 400–1000 (while also increasing stress intensities required for equivalent crack velocities in the composite as in the pure glass matrix). Thus SCG was essentially stopped by controlling composite character, specifically by substantial particle–matrix bonding.
Jessen and Lewis also conducted other studies of crack propagation and fracture in their Fe-Ni-Co alloy particles–glass matrix with two additional important crack propagation results. First, they showed that lower velocity cracks, especially SCG, had more opportunity to avoid intersecting the dispersed particles than fast cracks, e.g. lower velocity cracks intersected only about 1/2 of the particles in the crack path compared to fast cracks [82]. Second, they showed that DCB fracture toughnesses measured in samples with gradations of particle volume fraction were determined mainly by the composite character in the area of initial crack propagation, with subsequent crack propagation encountering composite areas with either less or more metal particles than the starting area having limited effect on toughness and crack propagation character [83,84]. Thus while the mechanisms and interrelation of these effects are not clearly established, they show that the initial composite character where fracture initiates has a dominating effect on failure and that crack–particle interactions, and hence composite behavior, change as crack velocity increases.
Becher et al. [85] showed similar n values for SCG in both Al2O3 and Al2O3-SiC whisker composites, but the former occurred at substantially lower K and thus had more SCG. Subsequently, dynamic fatigue tests of indented discs of Al2O3 with 25 weight% SiC whiskers in biaxial flexure in water at room temperature of Zeng et al. [86] overall showed similar results. Thus though compli-
Particle (and Grain) Effects |
485 |
cated by stress test issues, overall the composite had less susceptibility to SCG than comparable Al2O3 with the same porosity and grain size, in part due to n values 50–100% greater in the composite. They reported that while the whisker composite was more susceptible to initiation of SCG, it also had greater resistance to low-velocity crack propagation that dominates SCG than did pure Al2O3. What the results may be for crack propagation in the direction of the typical alignment texture of the whiskers is apparently unknown (the above tests were with cracks normal to the whisker texture, i.e. the cracks were parallel to the hot pressing direction).
Improved understanding and documentation of both fracture modes, SCG, and of other aspects of composite behavior should be enhanced by studies of crack propagation parallel with phase interfaces. More recently developed tests for such crack propagation make this much more feasible and meaningful. One example of this for ceramic–ceramic (glass–alumina) interfaces is that of Cazzato and Faber [87], and examples of some for metal–ceramic interfaces (also of interest for electronics) are for Al2O3-Nb [88,89] and Al2O3-Cu [90] interfaces.
While examples of crack bridging by dispersed particles in ceramic composites are common, as was noted earlier, very little study of larger scale behavior and character of cracks in ceramic composites has been made. However, Wu et al. [91,92] showed, using their microradiographic technique, increasing deflection, wandering, branching, and hence diffuseness of cracks resulting from a single crack introduced in ZTA (i.e. Al2O3-ZrO2) composites as the ZrO2 content increased (Fig. 8.15A–C). The resulting diffusiveness and complexity of such large-scale crack character was ≥ that of much coarser grain structure Al2O3 (Fig. 2.4). These same investigators also showed that such microradiographic examination of cracks in jade with its natural fibrous structure both fine and substantially oriented and a synthetic uniaxial fiber composite with fine SiC-based fibers were the only ceramics having more widely separated and complex crack branching on such a scale (Fig. 8.15D and E) [93].
The above discussion has focused on crack propagation under static or continuously increasing load. An important area of crack propagation that is being studied more is under cyclical loading, i.e. fatigue crack propagation, where again the issue of crack size can be important; most of the data are for large cracks in typical toughness test specimens such as CT and NB. While some monolithic ceramics exhibit net crack growth under cyclic loading that can be explained by SCG under continuous loading, some cannot. Greater cyclic growth of large cracks may arise from microstructural effects as is discussed in Chap. 2, Sec. III.G. Microstructural effects such as microcracking, crack branching, and especially bridging in the crack wake zone are commonly more prevalent or extensive in composite versus monolithic ceramics, so fatigue crack

FIGURE 8.15 Microradiographic images of the character of cracks propagating from a notch in DCB specimens of Al2O3 with (A)–(C) 0.5, 9, and 19 v/o ZrO2, (notch, right center, darker area), (D) a fine grain (fiber) jade, and (E) a uniaxial fiber (Nicalon)-ZrO2 ceramic composite with the fibrous structures aligned normal to the crack (notch, left center in D and E). Note the increasing tortuousness, complexity, width, and diffuseness of the resulting crack character as the content of unstabilized ZrO2 increases and in the fibrous structures. Compare to Fig. 2.4. ((A) to (C) after Wu et al. [91], published with permission of the ASTM; (D) and (E) from Ref. 93. Published with permission of the American Ceramic Society.)
Particle (and Grain) Effects |
487 |
propagation is frequent and substantial in them. Again, such crack growth can typically be characterized by the Paris relation:
dc/dN = C( K)m |
(8.6) |
where dc/dN is the rate of crack growth with the number of cycles (N), C and m are constants for a given body, and K is the increment of stress intensity. Values of m for metals are typically 2–4, but for ceramics they can range to substantially higher values, e.g. 20, though with at least some ceramic composites having lower values, and often higher K values for crack propagation [94]. While much of the fatigue study of composites has been to establish basic aspects of its occurrence rather than details of its microstructural dependence, some information on the latter is available.
Suresh [94] has reviewed fatigue behavior and noted some microstructural effects. Thus Mg-PSZ may have somewhat lower m values in the over-aged condition versus the peak strength condition (e.g. 21 versus 24), and Al2O3 with SiC particles lower values than Al2O3 by itself (e.g. 7 versus 8–14), and still less with the SiC as whiskers (e.g. m 4). However, few or no details on effects of differing concentrations, sizes, orientations, etc. are available. One partial exception to this is data on WC-Co, e.g. Suresh noted that increased Co content or in the Co mean free path (hence also an increase in G for WC) decreased the rate of fatigue crack growth, and that the fracture path is mainly intergranular along the Co binder, but that transgranular fracture of the WC increases as the WC grain size increases. Again, however it must be noted that documentation and understanding of the fatigue behavior of natural strength controlling flaws in ceramics is limited, especially on specifics of the microstructure, e.g. as noted by Grathwohl and Liu [95].
Consider now some crack propagation studies in ceramic composites; the first and most basic being some model studies of Nadeau and Dickson [96]. They made DT specimens of a commercial soda lime glass (SLG) such that some specimens had grooves of varying width and depths, as well as spacings that were ahead of and normal to the subsequently introduced sharp crack. The grooves were filled with a low-melting sealing glass that had a thermal expansion of 6 versus 8.5 ppm°C-1 for the SLG. Their studies of propagating a sharp crack into the region of such artificial microstructure showed that crack arrest and stress intensity for repropagation through the grooves filled with the sealing glass increased as the cross-sectional dimensions of the filled groves increased (e.g. from a fraction to 1 mm), and their spacing increased from a few to tens of mm. The strongest interaction was when the crack bifurcated into two branches nearly parallel with the filled groove, which increased the stress intensity for repropagation to 1.8 MPa·m1/2, i.e three times the toughness of the SLG. Crack interaction was velocity dependent; slow moving cracks were not
488 |
Chapter 8 |
retarded while fast ones were significantly retarded or arrested. These results are clearly consistent with the larger cracks having more interaction, especially with larger particles.
A second set of crack observations are those comparing crack propagation modes as a function of particle–matrix expansion differences. Davidge and Green [97], using ThO2 spherical particles (50–700 m) of differing sizes in various glass matrices showed crack propagation basically following the models of Fig. 8.9. Thus in composites with matrices of higher expansion than the dispersed particles, crack propagation tended to be through the particles, while in composites of matrices of lower expansion than the dispersed particles, crack propagation was around the particles. (See also the note at the end of this chapter.) With matrices of lower expansion than the ThO2 strengths varied inversely with the square root of the ThO2 particle size for each glass, Chap. 9, Sec. III.A, while those with the opposite expansion difference all fractured spontaneously, so no test results could be obtained. Frey and Mackenzie [31] also showed crack propagation tangentially around spherical ZrO2 particles with lower expansion than the glass matrix. Composites of spherical Al2O3 (125–150 m) particles in a high expanding glass formed many spontaneous cracks between and radial with the particles, but gross crack propagation tended to be by connection of preexisting cracks to follow an overall path that mostly went around the particles rather than intersecting them. Earlier work by Binns [32] showed that, when there was no expansion difference between the matrix and the dispersed phase, fracture was relatively flat and smooth and became more complex as expansion differences increased. He corroborated that cracks generally propagated around particles (but mainly in the glass) with expansions greater than the glass matrix. With irregularly shaped dispersed particles this tangential propagation occurred mainly at the angular corner extremities of the particles, with fracture further into the glass matrix between the extremities of the particles. When the glass matrix had higher thermal expansion than the dispersed particles, microcracks tended to form, often in an radial fashion, and macrocrack propagation occurred mostly by linking of the smaller cracks. Such microcracking was observed to occur above a threshold particle size at least qualitatively consistent with Eq. (2.4). Faber et al. [98] demonstrated substantially higher (I) toughness in composites of spherical Al2O3 particles ( 30 m) in a borosilicate (BS) versus an aluminosilicate (AS) glass. The former gave toughnesses up to four times the BS glass at φ= 0.3 (which was apparently a maximum), while the latter gave toughness linearly increasing to 2+ times that of the parent AS glass at the limits of composition tested of φ= 0.4. The higher toughness (and the apparent maximum) with the BS glass were attributed to microcracking (supported by independent observations) due to the greater expansion difference of 5.5 versus 4.4 ppm/°C. They also showed that their toughness versus volume content of dispersed Al2O3 with the AS glass was the same as for the composites of ThO2 particles in glasses of Davidge and Green above.
Particle (and Grain) Effects |
489 |
V.PARTICLE (AND GRAIN) EFFECTS ON FRACTURE TOUGHNESS IN CERAMIC COMPOSITES
A.Composites of Glass Matrices with Dispersed Ceramic Particles
This section addresses composites of ceramic particles dispersed in a (typically silicate) glass made by one of three methods that collectively give a range of composites and the ability to tailor their character. The most versatile method is forming a glass matrix around ceramic particles by dispersing the particles in a molten glass, or much more commonly consolidating a glass matrix from powder via sintering or hot pressing so the glass matrix forms around ceramic particles mixed with glass powder. The second method is via glass systems that undergo considerable crystallization on heat treatment after glass forming, which may give complex microstructures (Chap., 1, Sec. III) but can be a versatile and practical method. The third method is in part a forerunner of the second, namely a number of traditional ceramics such as whitewares, and especially porcelains which are natural composites of glass and crystalline ceramic phases, some of the latter from crystallization, but also frequently as residues from the starting mineral constituents. Though much of the investigation of these materials was done before extensive use of fracture mechanics and fracture toughness, there is some toughness data for these materials. More consideration of these materials will be given in conjunction with the more extensive data on their strengths (Chap. 9, Sec. III).
The dominant and pervasive second phase parameter in particulate composites besides the chemical composition, and hence the physical property differences between the matrix and the dispersed phase, is the volume fraction of second phase particles. There is substantial data to show that, while the quantitative values and their specific trends may vary with the methods and specifics of both fabrication and measurement, there is a broad trend for fracture toughness to increase with increasing amount, i.e. volume fraction, of added phase, φ. Many studies do not encompass a sufficient φ range to show the limits of such an increase, but those of broader φ ranges show toughness increases reaching a maximum, often at φ 0.5, and then decreasing. Such decreases are expected, e.g. since past φ= 0.5 many composites reverse the roles of matrix and dispersed phase. However, the maxima in toughness commonly found may occur at values of φ ≠ 0.5 for extrinsic or intrinsic reasons if other mechanisms are involved. Interaction of different dispersed phases, e.g. precipitation of different crystalline phases, or microcracking of the matrix (or of clusters of the original dispersed phase when it is the dominant phase), are examples in some glass matrix composites, and transformation effects are another.
Miyata et al. [99] measured (NB) toughnesses of hot pressed composites of glass matrices having thermal expansions greater (by 2.8 or 5 ppm/°C-1)