
brechignac_f_desmet_g_eds_equidosimetry_ecological_standardi
.pdf
423
statistical significant difference between the single-strand DNA breaks levels in blood leukocytes of animals sampled from studied areas was not found.
|
2 , 5 |
|
|
|
|
|
S M Z |
|
|
|
|
|
|
|
|
% |
2 |
|
* |
|
|
|
S P Z |
|
|
|
|
|
|||
|
|
* |
|
|
* |
||
DPC, |
|
|
|
|
|
||
1 , 5 |
* |
|
|
|
|
|
|
|
|
|
|
|
|
|
|
|
1 |
|
|
|
|
|
|
|
0 , 5 |
|
|
|
|
|
|
|
0 |
|
|
|
|
|
|
|
|
1 9 9 6 |
1 9 9 7 |
1 9 9 8 |
1 9 9 9 |
2 0 0 0 |
2 0 0 1 |
|
|
|
|
|
|
|
y e a r s |
Fig. 1. The levels of DNA-protein cross-links in white blood cells of voles sampled from the areas of the strict mode zone (SMZ) and the sanitary-protective zone (SPZ) of the SRW disposal site for several years (The data presented as the Meanρ SE). * - statistically significant with p<0.05.
The metabolic processes in cells depend on the state of the antioxidant defence systems of cells. For the estimation of the antioxidant systems in spleen lymphocytes of bank voles we use the treatment of cell suspension by hydrogen peroxide [7].
The results showed that spleen lymphocytes of bank voles sampled from the SMZ were more resisted to hydrogen peroxide compared to lymphocytes of animals from the SPZ (Fig 2). It is possible, that living of these animals in radionuclide contaminated areas results in a formation of an animals population with higher activity of the antioxidant defence systems.
|
5 0 |
S M Z |
|
|
DNA |
4 0 |
S P Z |
|
|
|
|
|
||
|
|
|
|
|
single-sranded |
3 0 |
|
|
|
2 0 |
|
|
|
|
1 0 |
|
|
|
|
% |
|
|
|
|
|
|
|
|
|
|
0 |
|
|
|
|
|
0 |
0 ,5 |
5 |
|
|
|
C on c en tration of h yd rog en p eroxid e , ɦɆ |
|
Fig. 2. The hydrogen peroxide - induction of the single strand DNA breaks in spleen lymphocytes of bank voles inhabited at the strict mode zone (SMZ) and the sanitaryprotective zone (SPZ) of the SRW disposal site. (The data presented as the Meanρ SE).
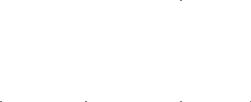
424
3.5. METALLOTHIONEIN CONTENT IN KIDNEY OF RODENTS
The increase in the metallothionein content in animal tissues is a well known indicator for environmental pollution [8]. This study showed that bank voles from the radioactive polluted areas have a tendency to increase the metallothionein content in their kidneys. However, no difference was noticeable for mice. It is easyly explained by the lower radionuclide accumulation for this spices of mice (Apodemus agrarius) in comparison to bank voles.
Table 7. The metallothionein content (mg/kg) in kidney of rodents sampled from different areas (The data presented as the Meanρ SD).
Areas |
mice |
bank voles |
Strict mode zone |
12.5±5.4 |
23.0±10.6 |
|
|
|
Sanitary-protective zone |
13.5±12.0 |
15.6±4.4 |
|
|
|
3.6. STUDY OF APOPTOSIS AND CYTOGENETIC DISTURBATIONS IN RODENTS TISSUES
In collaboration with Institute of Genetics & Cytology ( Belarus) we have studied the frequencies of the apoptotic cells and the cells with a micronucleus in rodents tissues. Bank voles were sampled from Belarus state biosphere natural reservation (BSBNR – control areas), from Polessky state radiation ecological reservation (PSRER) as well as from the strict mode zone of the Sergievo-Posadsky SRW disposal site
The analysis of the frequency of the cells with micronucleus and apoptosis markers in bone marrow showed the increase in the number of leukocytes with cytogenetic distributions and the decrease in the number of the apoptotic leukocytes (Table 8). A different ratio between these criteria in bone marrow was shown. In estimating the frequency of cells with different damage markers in thymus an other pattern have been noted. A positive correlation (r=0,76 P<0,01) between the frequency of cells with micronucleus and apoptotic cells in thymus was marked for rodents from the radioactive contaminated areas.
Table 8. The frequencies of apoptotic cells and cells with micronucleus in bone morrow and thymus of bank voles.
Areas of rodents |
Apoptotic cells, % |
Cells with micronucleus, % |
||
|
|
|
|
|
sampling |
Bone |
Thymus |
Bone marrow |
thymus |
|
marrow |
|||
|
|
|
|
|
Strict mode zone |
0.07±0.05 |
1.04±0.20 |
0.40±0.04 |
0.44±0.13 |
PSRER |
0.26±0.05 |
1.41±0.09 |
0.24±0.05 |
0.10±0.02 |
|
|
|
|
|
BSBNR (control) |
0.51±0.10 |
0.79±0.06 |
0.09±0.03 |
0.08±0.02 |
|
|
|
|
|
|
P1-3<0.01 |
P2-3<0.01 |
P1-3<0.05 |
P1-3<0.05 |
|
P2-3<0.02 |
P2-3>0.05 |
P2-3<0.05 |
P2-3>0.05 |
425
4. Conclusions
In summary, constant inhabiting of rodents at the SMZ of the SergievoPosadsky radioactive waste disposal site induces some genetic effects. However, these changes are not typical only for ionizing factors. Pathological-morphological changes in internal organs, muscle and osteal tissues were not noticed. Statistical significant changes in the haematological parameters and clinical status of animals were not detected. The data on the myeloperoxidase activity in blood neutrophiles and the sensitivity of spleen lymphocytes to hydrogen peroxide prove a development of some adaptive processes in bank voles from the SMZ of the Sergievo-Posadsky radioactive waste disposal site.
5. References
1.Radiation protection recommendations as applied to the disposal of long-lived solid radioactive waste. A report of The International Commission on Radiological Protection. Ann ICRP, 1998, V. 28(4), P. 1- 25
2.V. Maslov About carrying out of the complex radioecologic studies in biogeocenoses with a high radioactivity. In “Radioecological studies in natural biogeocenoses”. Ed. I. N. Verchovskay. M., Nauka, 1972, P. 9-21 (in Russian).
3.A. Zhitkovich, M. Costa A simple, sensitive assay to detect DNA-proten cross-links in intact cells and in vivo. Carcinogenesis, 1992, V. 13, P.1485-1489.
4.P. Kanter, H. Shwartz A fluorescence enhancement assay for cellular DNA damage. Mol. Pharmacol., 1982, V. 22, P.145-151.
5.R. Nartsissov Estimates of the mieloperoxidase activity in blood neutrophiles by Cytochemical assay for Laboratornoe delo, 1964, No 3, P. 150-153 (in Russian).
6.D. Eaton, M. Cherian Determination of metallothionein in tissues by cadmium-haemoglobin affinity assay.
Methods. Enzymol. 1991, V. 205. P. 83-90.
7.A. Osipov, A. Elakov, P. Puchkov et al. Estimation of the molecular and cytogenetic effects in mice chronically exposed to low dose-rate gamma-radiation. Russian Journal of Genetics , 2002 (in press).
8.G. Rott, B. Romantseva, B. Synzynys The metallothionein content in freshwater molluscs inhabited in reservoirs of the Middle Russia Russian Journal of Ecology, 1999, No. 4. P. 306-308.
USE OF PHOTOSYNTHESIS AND RESPIRATION OF HYDROPHYTES FOR WATER TOXICITY DEFINITION
E. PASICHNA
Institute of Hydrobiology, NASU, Geroyiv Stalingrada St., 12, Kiev, 04210, UKRAINE
1. Introduction
The problem of pollution of natural waters by heavy metals and other chemical elements is very acute today. It is therefore necessary to investigate the effects of such substances on different species of water organisms. Besides, it is very important to study the possibility of environmental toxicity estimation using physiological and biochemical processes of these organisms.
Hydrophytes are the production basis and the energy providing link in trophic chains of water ecosystems. Besides, the processes of photosynthesis and respiration of aquatic plants reflect a very important aspect of metabolism and an ability for generation of energy and synthesis of ATP [1]. A large sensitivity of gas exchange functions of hydrophytes to increased concentrations of toxic substances in water medium has been observed previously [2]. It is known that heavy metals are the ones of the prime pollutants of natural waters [3]. So, our intention is to investigate the effect of increased concentrations of copper and manganese on photosynthesis and respiration of water plants. Also, we tried to define a possibility of using their gas exchange functions for water toxicity biotesting.
2. Materials and methods
The hydrophytes used were submerged the higher aquatic plants Najas guadelupensis L. and Ceratophyllum demersum L. which have a significant surface of contact with water.
Investigations of gas exchange in the processes of photosynthesis and respiration were carried out by the method of electrometric oxygen determination. For this purpose, we use the system allowing the study of the physiological state of aquatic plants under a wide range of environmental conditions [4].
Copper (Cu2+) was added to the water medium, in which aquatic plants were grown, in concentrations 0.0005, 0.005, 0.02 mg/l, manganese (Mn2+) – 0.005, 0.02, 0.1, 0.2 mg/l.
Photosynthesis and respiration intensities were determined after 14-days experiments, when organisms had passed all phases of toxicities and adaptation (sometimes indeed, in short-term experiments we can observe a stimulation of physiological functions under the influence of toxic substances as a protective reaction of aquatic organisms to the destructive effect of the toxins).
3. Results and discussion
The results of the investigations show some regularity in changes of
photosynthesis and respiration intensity under influence of increased concentrations of Cu2+ or Mn2+.
427
F. Brechignac and G. Desmet (eds.), Equidosimetry, 427–431.
© 2005 Springer. Printed in the Netherlands.
428
As we can see (Table 1) addition to water 0.0005 mg/l Cu2+ or 0.005, 0.02 mg/l Mn2+ has no toxic effect on gas exchanges processes and even increases their intensity. At the same time, presence of 0.02 mg/l Cu2+ or 0.1, 0.2 mg/l Mn2+ in water medium leads to inhibition both photosynthesis and respiration.
Table 1. Influence of Cu2+ and Mn2+ on photosynthesis and respiration intensity of aquatic plants (n=4–5, P<0.05)
Medium |
Test organism |
Oxygen (mg/l·hour) |
P |
Toxicity |
|
|
|
||||
samples |
|
|
|
R |
(T) |
|
Photosynthesis |
Respiration |
|||
|
|
|
|
||
|
|
(P) |
(R) |
|
|
|
|
|
|
|
|
Control |
Najas |
4.78±0.05 |
1.41±0.05 |
3.39 |
0 |
|
Ceratophyllum |
3.64±0.17 |
1.32±0.03 |
2.76 |
0 |
|
|
|
|
|
|
+0,0005 |
Najas |
5.92±0,04 |
1.53±0,04 |
3.87 |
-0.14 |
mg/l Cu2+ |
Ceratophyllum |
5.14±0,32 |
1.62±0,07 |
3.17 |
-0.15 |
|
|
|
|
|
|
+0,005 |
Najas |
3.34±0.07 |
1.89±0.07 |
1.77 |
0.48 |
mg/l Cu2+ |
Ceratophyllum |
2.97±0.30 |
1.73±0.09 |
1.72 |
0.38 |
|
|
|
|
|
|
+0,02 |
Najas |
1.48±0.05 |
1.10±0.05 |
1.35 |
0.60 |
mg/l Cu2+ |
Ceratophyllum |
1.59±0.27 |
0.98±0.11 |
1.62 |
0.41 |
|
|
|
|
|
|
+0,005 |
Najas |
5.52±0.30 |
1.62±0.05 |
3.41 |
-0.01 |
mg/l Mn2+ |
Ceratophyllum |
4.53±0.27 |
1.57±0.08 |
2.89 |
-0.05 |
|
|
|
|
|
|
+0,02 |
Najas |
6.52±0.33 |
1.83±0.08 |
3.56 |
-0.05 |
mg/l Mn2+ |
Ceratophyllum |
4.95±0.34 |
1.90±0.15 |
2.60 |
0.06 |
|
|
|
|
|
|
+0,1 |
Najas |
3.97±0.30 |
1.31±0.04 |
3.03 |
0.11 |
mg/l Mn2+ |
Ceratophyllum |
2.93±0.08 |
1.15±0.10 |
2.55 |
0.08 |
|
|
|
|
|
|
+0,2 |
Najas |
2.78±0.35 |
1.14±0.08 |
2.44 |
0.28 |
mg/l Mn2+ |
Ceratophyllum |
2.41±0.17 |
1.05±0.09 |
2.29 |
0.17 |
It is observed that copper ions in concentration of 0.005 mg/l depress photosynthesis simultaneously while enhancing respiration. Apparently, it is a protective reaction of the organisms against stress as far as respiration is a source of energy requested for the reparation of damage appearing under the action of the metal [5].
Based on the results of the investigations, we can draw the conclusion that ions of copper are much more toxic for water plants than ions of manganese. Also, it is obvious that N. guadelupensis is more acceptable as a test object because this species is more sensitive to the action of toxic substances than C. demersum.
So, we can see that the reaction of plant organisms to the influence of increased concentrations of the metals show some regularities: small quantities of Cu2+ and Mn2+ stimulate the processes of vital activity and great quantities of the ions depress them. Such two-phase impact on gas exchange functions is conditioned by the fact that these metals are necessary for the structure and activity

429
of many physiological systems including those which take part in the processes of photosynthesis and respiration. In the same time, it is proven that high concentrations of heavy metals inhibit the enzymes because of connection with their active centres [6].
Thus, the changes in the oxygen content in the water (because of gas exchange functions) gives us a possibility to assess the concentration of the metal in the solution. However, absolute values of photosynthesis and respiration intensities depend on some factors, including the physiological state of the plant, the conditions of the experiment, etc. So, we can make a more informative and precise definition of water quality by using the correlation between photosynthesis and respiration intensities.
In this connection the following formula for estimating water toxicity is proposed (T) [4]:
T 1 Pex / Re x
Pc / Rc
where Pex and Rex are the registered values of photosynthesis and respiration in the experiment under influence of the investigated concentrations of the substances;
Pc and Rc are the photosynthesis and respiration values for control water without any toxins.
This method gives us a possibility to estimate toxicity not only of the solution with well-known substances (Table 1), but also to define the quality of any type of water. It is very important, as often we do not know the chemical composition of the water solution. Also, we must take into account that substances which are toxic to organisms when they exist individually often produce unexpected effect when they are present in combination. So, this method allows us to estimate toxicity of solution of any chemical composition to water plants.It can be proven by the results of quality determination of different kinds of water with use of N. guadelupensis and C. demersum. As we can see (Table 2), the toxicities of the water tested, measured by means of photosynthesis and respiration registration, varied considerably. Aquarium water as a control medium was not toxic (T=0). Water from waterconduct compared with the aquarium water affected test organisms, as it apparently contains residual amounts of chlorine. River water sometimes greatly influences the oxygen response of aquatic plants. We can observe that if contamination of water is within the limits of maximum concentrations of toxic substances permissible in fish industry (or water is rich in biogenic elements), the toxicity (T) is negative (water from the river of Dnieper). On the other hand, when the water contains great quantities of toxic components T shows a steady deviation on the positive side (water from the river of Shostka). Industrial wastes may kill the water plant, consequently, such water must be diluted.
430
Table 2. Toxicity of different waters, measured by means of the proposed method with use of N. guadelupensis and C. demersum (n=5, P<0.05)
Medium |
Test organism |
Oxygen (mg/l·hour) |
P |
Toxicity |
|
|
|
||||
samples |
|
|
|
R |
(T) |
|
Photosynthesis |
Respiration |
|||
|
|
|
|
||
|
|
(P) |
(R) |
|
|
|
|
|
|
|
|
Aquarium |
Najas |
4.93±0.08 |
1.45±0.06 |
3.40 |
0 |
water |
Ceratophyllum |
3.79±0.12 |
1.37±0.05 |
2.77 |
0 |
|
|
|
|
|
|
Water-pipe |
Najas |
4.35±0.10 |
1.57±0.08 |
2.78 |
0.18 |
|
Ceratophyllum |
3.41±0.22 |
1.44±0.11 |
2.36 |
0.14 |
|
|
|
|
|
|
Dniepr river |
Najas |
6.20±0.14 |
1.67±0.09 |
3.72 |
-0.10 |
water |
Ceratophyllum |
|
|
|
|
(medium |
4.48±0.26 |
1.52±0.07 |
2.95 |
-0.07 |
|
part) |
|
|
|
|
|
Shostka |
|
|
|
|
|
river water |
Najas |
|
|
|
|
(nearby the |
1.01±0.35 |
1.06±0.14 |
0.95 |
0.72 |
|
city of |
Ceratophyllum |
|
|
|
|
Shostka) |
1.46±0.24 |
1.11±0.11 |
1.32 |
0.52 |
|
Thus, natural and industrial waters can be classified in the following way: |
|
||||
T>0 |
water is toxic |
|
|
|
|
T<0 |
water is eutrophic |
|
|
|
|
T§0 |
water is not toxic. |
|
|
|
|
4. Conclusion
The results of the water toxicity measurements by using the proposed method demonstrate that it can be helpful for both the study of photosynthesis and respiration of aquatic plants in general, and for water toxicity measurements as well.
Further researches must be directed for revealing the most sensitive species of plants to a chemical stress (to use them as test organisms), and for studying effects of individual and simultaneous actions of different chemical substances on physiological functions of hydrophytes.
5. References
1.R.Polystchuk. To the question of choose of tests for estimation of the toxic substances action on algae. Byologya morya, Kiev, 1975, V. 35, pp. 58–65 (in Russian).
2.L.Braginsky, I.Velitchko and E.Stcherban. Freswater plankton in toxic medium. Kiev, Naukova dumka, 1987, 178 p. (in Russian).
3.A.Bozhkov, S.Mogilyanskaya. Relationship between Cu2+ ion concentration in medium, bioaccumulation and toxicity for the microalgae Dunaliella viridis Teod. Algologia, 1994, V.4, N 3, pp. 22–29.
4.A.Mereshko, E.Pasichnaya, A.Pasichnyi. Biotesting the toxicity of aquatic environment by functional characteristics of macrophytes. Hydrobiological j., 1998, V. 34, N 2–3, pp. 103–110.
5.R.Polystchuk. Reaction of overgrowing macrophytes on influence of heavy metals ions. In: The biological bases of fight against overgrowing. Êiev, Naukova dumka, 1973, pp. 155–193 (in Russian).
431
6.Physiology of plant organisms and the role of metals. Edited by N.Chernavina. Moskow University Publishing House, 1989, 155 p. (in Russian).
LIST OF PARTICIPANTS (NATO ARW)
“Ecological standardization and equidosimetry for radioecology and environmental ecology”
Kiev, Ukraine, 14-20 April 2002
Key Speakers from NATO Countries:
Henri Maubert |
CEACadarache. Radioprotection Service (France) Henri |
|
Maubert, SPR Bt 355, 13108 Saint Paul lez Durance, |
|
France |
Randall C. Morris |
TREC, Inc., and the Environmental Science and Research |
|
Foundation, USA |
James D. Navratil |
Environmental Engineering and Science |
|
Clemson University, USA |
George Shaw |
Department of Environmental Science & Technology |
|
Faculty of Life Sciences |
|
Imperial College at Silwood Park Ascot, Berkshire, SL5 |
|
7PY, United Kingdom |
Guillaume Echevarria |
Laboratoire Sols et Environnement |
|
UMR 1120 ENSAIA-INPL/INRA, BP 172 |
|
2, avenue de la Forɤt de Haye |
|
54505 Vandoeuvre-les-Nancy, France |
Gerassimos Arapis |
Agricultural University of Athens, Laboratory of Ecology |
|
and Environmental Sciences, Iera Odos,75-botanokos, |
|
Gr-11855, Athens, Greece |
Michael Karandinos |
Agricultural University of Athens, Laboratory of Ecology |
|
and Environmental Sciences, Iera Odos,75-botanokos, |
|
Gr-11855, Athens, Greece |
433
F. Brechignac and G. Desmet (eds.), Equidosimetry, 433–436.
© 2005 Springer. Printed in the Netherlands.
434
Other Participants from NATO Countries:
Mustafa Alparslan |
Canakkale Onsekiz Mart University,Faculty of Fisheries |
|
Terzioglu Campus, 1700 Canakkale/Turkey |
Jiy.K. Kim |
Korea Atomic Energy Research Institute, 150 Dukjin- |
|
dong, Yusong-gu, Taejon, 305-353 Korea |
Marie Svadlenkova |
University of South Bohemia, Dept.of Physics, |
|
Jeronymova10. 371 14 Ceske Budeiovoce, Czech |
|
Republik |
Key Speakers from Partner Countries: |
|
Alexander Dvornik |
Forest Institute of NAS Belarus,BY 246654, Gomel, |
|
Belarus |
Vassili Davydchuk . |
Institute of Geography NASU, UA 252034, Kiev, |
|
Ukraine |
Yuri Kutlakhmedov |
Taras Shevchenko National Kiev Univeristy 03022, Kiev, |
|
Ukraine |
Valeriy Kashparov |
Institute of Agricultural Radiology , UA 255205,Kiev, |
|
Ukraine |
Alexandr Orlov |
Polesskya Agro-forest-ameliorative scientific research |
|
station,UA 262004, Zhitomir, Ukraine |
Georgiy Perepelyatnikov |
Ukraine Radiation Training Centre, RU 252022, Kiev, |
|
Ukraine |
Staniclav Geraskin |
Russian Institute of agricultural radiology and |
|
Agroecology, Ru-249020, Obninsk, Russia |
Vladimir Georgievsy |
Institute of Atomic Energy named Kurchatov, Moscow, |
|
Russia |
Dmitry Grodzinsky |
Institute of Cell Biology and Genetic Engineering NASU, |
|
UA 252022, Kiev, Ukraine |
Vladimir Kovalevsky |
Ministry of Defense of Ukraine, Kiev, Ukraine |
Mikhail Kuzmenko |
Institute of Hydrobiology NASU, Kiev, Ukraine |
Vladislav Petin |
Russian Institute of medical radiology, Ru-249020, |
|
Obninsk, Russia |