
Учебники / Genetic Hearing Loss Willems 2004
.pdf204 |
Willems |
III.GENES FOR NONSYNDROMIC HEARING LOSS
The first nuclear gene involved in nonsyndromic HL was identified in 1995 as POU3F4. Two more genes, myosin 7A and connexin 26, were isolated in 1997. In the last 5 years an impressive list of 25 additional genes have been identified, bringing the total of nonsyndromic HL genes to 28 in June 2002.
The spectrum of genes (Table 4) includes cytoskeletal proteins, such as diaphanous and the myosins 3A, 6, 7A, 15 and myosin heavy chain 9; structural proteins of the organ of Corti, such as alpha tectorin, stereocilin, COL11A2 en otoancorin; transcription factors, such as POU3F4, POU4F3, and EYA4; ion transport and channel proteins such as connexins 26, 30, 31, and 43, pendrin, KCNQ4, TMC1, and claudin 14; and proteins of unknown function such as icere, cochlin, otoferlin, wolframin, CDH23, USH1C, and TMPRSS3. The largest groups of homologous genes are the myosins and the connexins (each four genes). Apart from the nuclear genes, mutations in mitochondrial genes (12S rRNA and tRNA-ser) have also been found to be associated with nonsyndromic HL.
Some of the HL genes, including MYO7A, MYH9, COL11A2, GJB2, GJB3, SLC26A4, WFS1, CDH23, and tRNA-ser, are responsible for both nonsyndromic and syndromic HL. Most of these genes are involved in only a limited number of pedigrees up to now, with the exception of GJA2
(connexin 26), KCNQ4, SLC26A4, COCH, POU3F4, and 12S rRNA. Connexin 26 mutations are among the most frequent mutations in the human genome, being present in 1–4% of most Caucasian individuals, and representing almost half of all autosomal recessive deafness.
REFERENCES
1.Leon PE, Raventos H, Lynch E, Morrow J, King MC. The gene for an inherited form of deafness maps to chromosome 5q31. Proc Natl Acad Sci USA 1992; 89:5181–5184.
2.Tekin M, Arnos KS, Pandya A. Advances in hereditary deafness [Review]. Lancet 2001; 358:1082–1090.
3.Resendes BL, Williamson RE, Morton CC. At the speed of sound: gene discovery in the auditory system [Review]. Am J Hum Genet 2001; 69:923–935.
4.Steel KP, Kros CJ. A genetic approach to understanding auditory function [Review]. Nat Genet 2001; 27:143–149.
5.Willems PJ. Genetic causes of hearing loss. N Engl J Med 2000; 342:1101–1109.
6.Van Camp G, Willems PJ, Smith RJ. Nonsyndromic hearing impairment: unparalleled heterogeneity [Review]. Am J Hum Genet 1997; 60:758–764.
7.Kalatzis V, Petit C. The fundamental and medical impacts of recent progress in research on hereditary hearing loss [Review]. Hum Mol Genet 1998; 7:1589–1597.
Gene Localization and Isolation |
205 |
8.Morton CC. Genetics, genomics and gene discovery in the auditory system. Hum Mol Genet 2002; 11:1229–1240.
9.www.uia.ac.be/dnalab/hhh/
10.Skvorak AB, Weng Z, Yee AJ, Robertson NG, Morton CC. Human cochlear expressed sequence tags provide insight into cochlear gene expression and identify candidate genes for deafness. Hum Mol Genet 1999; 8:439–452.
11.Steel KP. Inherited hearing defects in mice [Review]. Annu Rev Genet 1995; 29:675–701.
14
Connexins
Paolo Gasparini
Second University of Naples and Telethon Institute of Genetics and
Medicine, Naples, Italy
I.INTRODUCTION
Connexins are a large family of proteins involved in formation of gap junctions that allow the direct transfer of small molecules and ions between neighboring cells (1). Transmission at gap junction synapses is very fast allowing the production of almost instantaneously acting potentials. Gap junctions are rare between mammalian neurons, but are common in nonneural cells, such as glia, epithelial cells, and smooth and cardiac muscle cells. Three groups of connexins exist, named alpha, beta, and non-alpha–non- beta, as shown in Figure 1. Each connexin is identified by a number directly related to its molecular weight. Connexins form hexameric hemichannels (termed ‘‘connexons’’) in the endoplasmic reticulum, which are then translocated into the plasma membrane. The connexon then ‘‘docks’’ with a connexon of an adjacent cell to form a functional channel termed a ‘‘gap junction.’’ Connexons can form either homotypic, heterotypic, or heteromeric channels. Connexins are expressed in many di erent tissues, including skin and inner ear. In the epidermis, gap junctions appear to play a role in the coordination of keratinocyte growth and di erentiation (2), whereas several arguments support the idea that gap junctions have an important role in auditory transduction. The auditory organ has gap junctions between the outer hair cells and supporting cells (including melanocytes), providing a morphological basis for the occurrence of intracellular responses to sound in supporting cells and for electric coupling of receptor cells. The endothelium of the scala media of the cochlea is involved in the production of a receptor response to the auditory stimulus and is separated
207
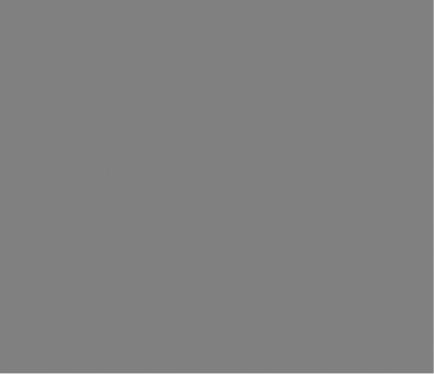
208 |
Gasparini |
Figure 1 Connexin family tree (courtesy of D. Condorelli). Schematic representation of connexin family tree with division into alpha, beta, and non-alpha–non-beta groups.
from the endolymphatic space by tight junctions in the marginal cell layer, which is coupled to gap junctions. Immunohistochemical and ultrastructural analysis of some members of this protein family (connexin 26) in the rat cochlea showed that gap junctions in both epithelial and connective tissue cells are involved in recycling endolymphatic potassium ions (3).
The identification of mutations in several connexin genes in patients with sensorineural hearing loss definitively confirmed the involvement of gap junctions in the endocochlear potential of audition. Mutations in the gap junction genes encoding the connexins have also been shown to cause epidermal disease and peripheral neuropathy (4, 5; Connexin-Deafness Homepage at http://www.iro.es/deafness/).
Connexins |
209 |
II.CONNEXINS AND NONSYNDROMIC AUTOSOMAL RECESSIVE DEAFNESS (NSRD)
Approximately 80% of cases of congenital deafness are inherited in an autosomal recessive fashion or are apparently sporadic (2). Despite the fact that more than 30 loci have been described for nonsyndromic autosomal recessive deafness (DFNB) (see Hereditary Hearing Loss Homepage at http://dnalab-www.uia.ac.be/dnalab/hhh/), a single locus, DFNB1 (MIM 220290), accounts for a high proportion of the cases, with variability depending on the population. The gene involved in this type of deafness is GJB2, which encodes the gap junction protein connexin 26 (Cx26).
DFNB1 was mapped to 13q12 in two consanguineous Tunisian families (6) and further confirmed by linkage in large series of patients (7,8). In 1997, two independent studies demonstrated the role of the GJB2 gene in this type of deafness. In the first study, two nonsense mutations were found in three families from Pakistan linked to DFNB1 (9). In the second study, 35 Mediterranean families with autosomal recessive NSHL were used to refine the DFNB1 interval to 5 cM between markers D13S175 and D13S232, which contains the GJB2 gene. The following mutational search of the GJB2 coding region in these families showed that 19 of 35 patients were homozygous for a specific deletion, named 35delG (10). Hearing-impaired individuals from another family were found to be compound heterozygotes for 35delG and another deletion named 167delT. Both sequence variants produce a frameshift in the GJB2 mRNA, leading to a premature truncated protein and loss of function. The above-mentioned deletions show a worldwide distribution that can be dependent on the ethnic background.
The 35delG mutation appears to be more frequent in Caucasians of European origin (10–16), being particularly frequent in South European countries (12). Conversely, 35delG mutation was absent in patients and controls of Japanese origin (17–19). Since 35delG is located within a stretch of G, a mutational hot spot was hypothesized; however, the fluctuation in carrier status of 35delG in normal-hearing populations from di erent ethnic groups (1 in 35 in southern Europe and 1 in 79 in northern Europe) suggests a possible founder e ect and positive selection for 35delG heterozygote status (20,21).
Mutation 167delT, originally reported in 2.3% of GJB2 mutant alleles of Mediterranean origin (10), was then found to be very common in Ashkenazi Jews. The presence of a conserved haplotype suggests a founder event for its origin (22–24). Several additional mutations have been identified so far in the GJB2 gene (25). Figure 2 reports the localization of CX26 missense mutations, which are scattered along the protein apart from the
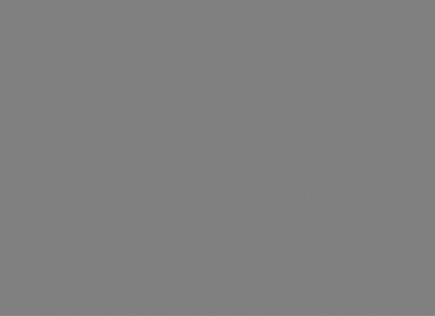
210 |
Gasparini |
Figure 2 Distribution of missense mutations along CX26 gene and protein. Mutations detected in autosomal dominant forms as well as those identified in syndromic cases of hearing loss are clearly identified.
few dominant and syndromic mutations (see below), which are clustered in a specific domain. The density along GJB2 protein of missense mutation (a), of frameshift mutations (b), and of nonsense mutations (c) is graphically shown in Figure 3a, b, and c. While missense mutations are, more or less, distributed with an equal density along the protein, frameshift mutations are clustered in two major areas and nonsense mutations are more frequent in the first half of the gene itself.
Among GJB2 mutations it is worthwhile to annotate mutation 235delC, which has been detected only in individuals of Japanese origin (17–19). An updated list of all mutations detected in GJB2 is also available at the Connexin-Deafness Homepage, while a schematic representation of their localization along GJB2 protein is shown in Figure 2.
The audiological phenotype observed in individuals with GJB2 mutations is variable, depending largely on the type of the mutation present in each a ected patient. Usually, the onset is during early childhood and in most cases hearing loss is present at birth (congenital). In our experience
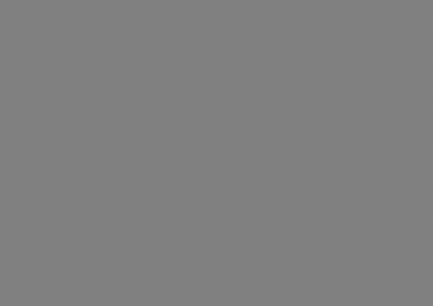
Connexins |
211 |
patients homozygous for the 35delG mutation are characterized by a severe to profound bilateral sensorineural hearing loss (12). This finding has been also reported by other researchers (13,26,27), and in a few cases progression of the sensory deficit in some 35delG homozygotes has also been observed (28). Patients heterozygous compound for 35delG and missense mutation or compound for other mutations present in the mayority of cases with a progressive hearing loss of mild to severe degree. In patients homozygous for 167delT mutation, a trend in the variability of the degree of hearing loss was also evident (23). The variability of the degree of hearing loss can be explained either by a di erent functional role of each mutated allele and its possible residual activity or by the possible presence of environmental and genetic factors.
After GJB3 (see below) had been found to be associated with autosomal dominant deafness, 25 Chinese families with autosomal recessive NSRD were screened for GJB3 mutations. Two unrelated families were identified with
Figure 3 Graphic distribution of the density of GJB2 missense (a), frameshift (b), and nonsense (c) mutations along the protein. The Y scale is 10 . The residue window is 12 amino acids; thus a value of 60 means the presence of six di erent mutations in a portion of 12 residues. (Courtesy of S. Volinia.)
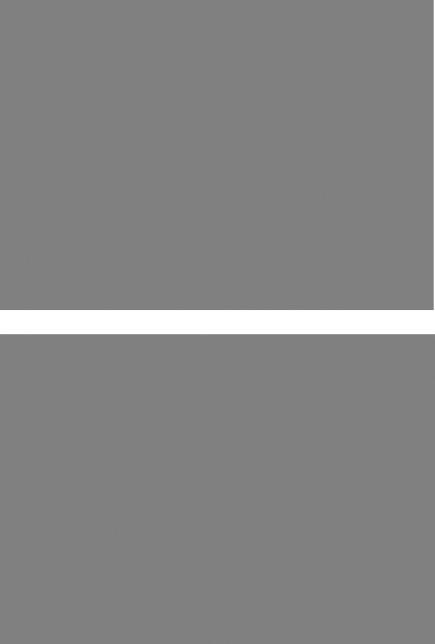
212 |
Gasparini |
Figure 3 Continued.
Connexins |
213 |
compound heterozygous GJB3 mutations, indicating that, like GJB2, recessive GJB3 mutations are also associated with NSRD (29).
Finally, mutations in connexin 43 (GJA1), a member of the connexin family expressed in cochlear tissues, have recently been identified in African American deaf patients. Four patients of 26 were homozygous for a missense mutation, named L11F, while another one was homozygous for a V24A mutation. These data suggest a possible major role of this gene in black people (30).
The recent identification of a recessive mutation in GJB6 and the possible presence of digenic inheritance is described in the following section.
III.CONNEXINS AND NONSYNDROMIC AUTOSOMAL DOMINANT DEAFNESS (NSAD)
About 40 loci have been described for nonsyndromic autosomal dominant deafness (DFNB) (see Hereditary Hearing Loss Homepage at http://dnalabwww.uia.ac.be/dnalab/hhh/), and 16 di erent genes have been identified so far. Three of them belong to the connexin family.
Few GJB2 mutations have been described as causing dominant deafness (9,31; Connexin-Deafness Homepage). M34T has been found in heterozygosity in a family with a few a ected subjects (9), but also in normal subjects (13,15,32) and in a recessive family (13). It is believed that M34T is a dominant mutation with reduced penetrance, and functional studies performed on some mutations by expression in Xenopus laevis oocytes showed that M34T had a dominant negative e ect, whereas the recesive mutation W77R did not (33). W44C was identified as the cause of deafness in several families with nonsyndromic autosomic dominant deafness (31). The localization of dominant mutations within GJB2 is shown in Figure 2. These mutations are clustered in the first extracellular domain of the protein.
Mutation analysis of the GJB3 (CX31) gene, mapping at 1p33–p35, revealed that a missense mutation and a nonsense mutation were associated with high-frequency hearing loss in two families. Moreover, expression of Gjb3 was identified in rat inner ear tissue by RT-PCR, further confirming that mutations in GJB3 may be responsible for bilateral high-frequency hearing impairment (34).
Grifa et al. (35) cloned the human connexin 30 (GJB6) gene, localized it in the same YAC contig of GJB2 on 13q11, and showed expression of GJB6 in the mouse cochlea. Analysis of deaf patients led to the identification of a missense mutation in a family with dominant hearing impairment, although with phenotypic variation. The authors have demonstrated by studies in Xenopus laevis that the GJB6 T5M mutation has a dominant negative e ect