
Учебники / Genetic Hearing Loss Willems 2004
.pdf164 |
Dixon and Dixon |
21.Dixon J, Gladwin AJ, Loftus SK, Riley J, Perveen R, Wasmuth JJ, Anand R, Dixon MJ. A yeast artificial chromosome contig encompassing the Treacher Collins syndrome critical region at 5q31.3–q32. Am J Hum Genet 1994; 55:372– 378.
22.Li X, Wise CA, Le Paslier D, Hawkins AL, Gri n CA, Pittler SJ, Lovett M, Jabs EW. A YAC contig of approximately 3 Mb from human chromosome 5q31–q33. Genomics 1994; 19:470–477.
23.Loftus SK, Dixon J, Koprivnikar K, Dixon MJ, Wasmuth JJ. Transcriptional map of the Treacher Collins candidate gene region. Genome Res 61996; 26–34.
24.The Treacher Collins Syndrome Collaborative Group. Positional cloning of a gene involved in the pathogenesis of Treacher Collins syndrome. Nat Genet 1996; 12:130–136.
25.Dixon J, Edwards SJ, Anderson I, Brass A, Scambler PJ, Dixon MJ. Identification of the complete coding sequence and genomic organisation of the Treacher Collins syndrome gene. Genome Res 1997; 7:223–234.
26.Wise CA, Chiang LC, Paznekas WA, Sharma M, Musy MM, Ashley JA, Lovett M, Jabs EW. TCOF1 encodes a putative nucleolar phosphoprotein that exhibits mutations in Treacher Collins syndrome throughout its coding region. Proc Natl Acad Sci USA 1997; 94:3110–3115.
27.Chelsky D, Ralph R, Jonak G. Sequence requirements for synthetic peptidemediated translocation to the nucleus. Mol Cell Biol 1989; 9:2487–2492.
28.Marsh KL, Dixon J, Dixon MJ. Mutations in the Treacher Collins syndrome gene lead to mislocalisation of the nucleolar protein treacle. Hum Mol Genet 1998; 11:1795–1800.
29.Winokur ST, Shiang R. The Treacher Collins syndrome (TCOF1) gene product, treacle, is targeted to the nucleolus by signals in its C-terminus. Hum Mol Genet 1998; 7:1947–1952.
30.Isaac C, Marsh KL, Dixon J, Paznekas W, Dixon MJ, Jabs EW, Meier UT. Characterization of the nucleolar gene product of Treacher Collins syndrome in patient and control cells. Mol Biol Cell. 2000; 11:3061–3071.
31.Jones NC, Farlie PG, Minichiello J, Newgreen DF. Detection of an appropriate kinase activity in branchial arches I and II that coincide with peak expression of the Treacher Collins sydrome gene product, treacle. Hum Mol Genet 1999; 8:2239–2245.
32.Emes RD, Ponting CP. A new sequence motif linking lissencephaly, Treacher Collins and oral-facial-digital type 1 syndromes, microtubule dynamics and cell migration. Hum Mol Genet 2001; 10:2813–2820.
33.Lo Nigro C, Chong CS, Smith AC, Dobyns WB, Carrozzo R, Ledbetter DH. Point mutations and an intragenic deletion in LIS1, the lissencephaly causative gene in isolated lissencephaly sequence and Miller-Dieker syndrome. Hum Mol Genet 1997; 6:157–164.
34.Gladwin AJ, Dixon J, Loftus SK, Edwards SJ, Wasmuth JJ, Hennekam RCM, Dixon MJ. Treacher Collins syndrome may result from insertions, deletions or splicing mutations, which introduce a termination codon into the gene. Hum Mol Genet 1996; 5:1533–1538.
Treacher Collins Syndrome |
165 |
35.Edwards SJ, Gladwin AJ, Dixon MJ. The mutational spectrum in Treacher Collins syndrome reveals a predominance of mutations that create a premature termination codon. Am J Hum Genet 1997; 60:515–524.
36.Splendore A, Silva EO, Alonso LG, Richieri-Costa A, Alonso N, Rosa A, Carakushanky G, Cavalcanti DP, Brunoni D, Passos-Bueno MR. High mutation detection rate in TCOF1 among Treacher Collins syndrome patients reveals clustering of mutations and 16 novel pathogenic changes. Hum Mutat 2000; 16:315–322.
37.Dixon J, Hovanes K, Shiang R, Dixon MJ. Sequence analysis, identification of evolutionary conserved motifs and expression analysis of murine TCOF1 provide further evidence for a potential function for the gene and its human homologue, TCOF1. Hum Mol Genet 1997; 6:727–737.
38.Haworth KE, Islam I, Breen M, Putt W, Makrinou E, Binns M, Hopkinson D, Edwards Y. Canine TCOF1: cloning, chromosome assignment and genetic analysis in dogs with di erent head types. Mamm Genome 2001; 12:622–629.
39.Aebi M, Hornig H, Weissmann C. 5V cleavage site in eukaryotic pre-mRNA splicing is determined by the overall 5V splice region, not by the conserved GU. Cell 1987; 50:237–246.
40.Paznekas WA, Zhang N, Gridley T, Jabs EW. Mouse TCOF1 is expressed widely, has motifs conserved in nucleolar phosphoproteins, and maps to chromosome 18. Biochem Biophys Res Commun 1997; 238:1–6.
41.Nicolaides KH, Johansson D, Donnai D, Rodeck CH. Prenatal diagnosis of mandibulofacial dysostosis. Prenat Diagn 1984; 4:201–205.
42.Meizner I, Carmi R, Katz M. Prenatal ultrasonic diagnosis of mandibulofacial dysostosis [Treacher Collins syndrome]. J Clin Ultrasound 1991; 19:124–127.
43.Milligan DA, Harlass FE, Du P, Kopelman JN. Recurrence of Treacher Collins syndrome with sonographic findings. Mil Med 1994; 159:250–252.
44.Cohen J, Ghezzi F, Goncalves L, Fuentes JD, Paulyson KJ, Sherer DM. Prenatal sonographic diagnosis of Treacher Collins syndrome: a case and review of the literature. Am J Perinatol 1995; 12:416–419.
45.Dixon J, Brakebusch C, Fa¨ssler R, Dixon MJ. Increased levels of apoptosis in the prefusion neural folds underlie the craniofacial disorder. Treacher Collins syndrome. Hum Mol Genet 2000; 10:1473–1480.
11
MYH9
Anil K. Lalwani and Anand N. Mhatre
New York University, New York, New York, U.S.A.
I.INTRODUCTION
In 1993, a five-generation family with hereditary hearing impairment associated with cochleosaccular degeneration was identified (Fig. 1) (1). Through linkage analysis, this family was mapped to chromosome 22q12.2–q13.3, spanning a 17–23-cM region, defining a new locus for nonsyndromic hereditary hearing impairment DFNA17 (2). Subsequently, a mutation in MYH9, responsible for the DFNA17 was identified (3). The mutation R705H, a consequence of G–A transition in the nucleotide sequence, is considered critical for the ATPase activity of the myosin motor domain. Simultaneously, mutations in MYH9 have also been identified in hereditary macrothrombocytopenia (4,5). Herein, the DFNA17 phenotype and genotype-phenotype correlations are discussed.
II.AUDIOLOGICAL FINDINGS
Of the 22 member of the family, eight had hearing impairment; six were males and two were females. In the a ected members of the family, hearing loss began as a mild high-frequency deficit apparent by age 10–12 that progressed during adolescence (Fig. 2). By the third decade of life, the hearing loss was moderate to severe. Despite the similarity in the nature and progression of hearing loss, there was variation in the severity of hearing loss among the a ected family members (Fig. 3). Because of the limited
167
168 |
Lalwani and Mhatre |
Figure 1 Pedigree of the DFNA17 family with progressive high-frequency hearing impairment associated with cochleosaccular degeneration.
Figure 2 Pure tone thresholds for the right ear averaged for 500, 1000, 2000, and 3000 Hz. Test results show a mild hearing impairment in all subjects at age 13 with progression of the loss with aging.
MYH9 |
169 |
Figure 3 Variability in hearing loss. Shown is the hearing impairment observed for di erent a ected individuals between the ages of 18 and 20 years. The proband, III-2, shows the most significant hearing impairment when compared with his three children and his nephew.
number of a ected individuals, it was not possible to determine the rate of progression of hearing impairment. In some members of the family with significant hearing impairment, the word identification scores were good considering the degree of hearing loss. The preservation of clarity with a significant sensorineural hearing impairment is reminiscent of Schucknecht’s strial presbyacusis.
Distortion product otoacoustic emissions (DPOAE) were also performed on the a ected and una ected members of the family. Presence or absence of otoacoustic emissions reflected the level of hearing loss of the individual being tested. OAE were present in individuals with mild hearing loss and absent in members with profound impairment. Therefore, OAE cannot be used for early identification of individuals harboring the mutated gene prior to the development of hearing impairment. Similar to OAE, auditory brainstem responses (ABR) reflected the severity of hearing impairment. ABR were normal in individuals with mild hearing impairment and absent in deaf individuals.
170 |
Lalwani and Mhatre |
III.TEMPORAL BONE FINDINGS
The temporal bone of the proband, a 61-year-old profoundly deaf man, demonstrated classic findings associated with Scheibe, or cochleosaccular, degeneration: degeneration of the stria vascularis, the organ of Corti, and the saccular sensory epithelium. There was collapse of Reissner’s membrane onto the spiral ligament and area of organ of Corti. Stria vascularis was either absent or replaced by basophilic granules. The population of spiral ganglion neurons was reduced to one third of normal levels. Consistent with cochleosaccular degeneration, the utricle and the ampullae of the semicircular canals appeared to be completely normal.
IV. MYH9, A CONVENTIONAL NONMUSCLE MYOSIN
The DFNA17 locus was mapped to a relatively large genetic region of 17–23 cM on chromosome 22q12.2–q13.3, typical for the size of the family studied
(2). The low resolution of the linked region precluded use of the positional cloning approach to identify the disease gene. The candidate gene approach, default alternative to positional cloning, was thus pursued to identify the mutant gene within the DFNA17 locus. Analysis of the chromosome 22gene map identified 163 candidate genes within the region spanning the DFNA17 locus (6). One of the candidate genes encoding the nonmuscle myosin heavy chain A (MYH9), was selected for further analysis. The selection of MYH9, a member of the class II or conventional myosin, was based upon identification of mutations in several other myosin heavy-chain genes that are pathogenically linked to HHI (7).
The class II myosins are broadly expressed in skeletal, cardiac, and smooth muscles as well as nonmuscle tissues and consist of a pair of heavy chains, a pair of light chains, and a pair of regulatory light chains (8). The N-terminal motor domain is the most highly conserved region of the myosin heavy chain and contains the ATPand actin-binding sites. The apparent molecular weight of the class II myosin heavy chain is 200 kDa. The myosin that mediates skeletal muscle contraction, also known as the sarcomeric myosin, represents the most well-characterized representative of the class II myosin family. Cardiac and smooth muscle cells also express isoforms of class II myosin, distinct from the sarcomeric myosin that mediates contraction in these muscle cells.
Characterization of myosins in nonmuscle cells has identified two distinct isoforms of myosin II: nonmuscle myosin-IIA (MYH9) (9,10) and nonmuscle myosin-IIB (MYH10) (10). MYH9 and MYH10 have been mapped to 22q11.2 and 17q13, respectively, and exhibit 85% identity in their motor
MYH9 |
171 |
domains. Several skeletal-muscle heavy-chain genes have also been localized to the region containing MYH10. Most cells express relatively equal amounts of each of these myosins, with a few exceptions; platelets express MYH9 only (11,12) while neuronal tissues predominantly express MYH10. MYH9 and MYH10 demonstrate overlapping but distinct intracellular locations when coexpressed within the same cell type (13,14). In vitro motility studies of these two isoforms has shown that MYH9 is several-fold faster than MYH10 in its rate of ATP hydrolysis and movement of actin filaments (15). The di erences in their localization and their in vitro characteristics suggest di ering in vivo functions.
Sequence analysis of MYH9 in the DFNA17 family identified a G–A transition at nucleotide 2114 that cosegregated with the inherited autosomal dominant hearing impairment (3). This missense mutation changes codon
Figure 4 DFNA17 mutation R705H resides in a highly conserved and functionally important myosin heavy-chain motor domain.
172 |
Lalwani and Mhatre |
705 from an invariant arginine (R) to histidine (H), R705H. The identified mutation R705H within MYH9 occurs in a highly conserved and functionally critical region within the carboxy-terminal half of the myosin heavychain motor domain that forms the globular head in the hexameric myosin molecule (Fig. 4). Within the motor domain, R705 resides in a highly conserved linker region spanning 16 amino acids that contains two free thiol groups, C704 (SH1) and C694 (SH2). Crystal structure of the globular head of the skeletal muscle myosin has shown that the two thiols are part of two short a-helices joined through a kink at the conserved G696 residue (16,17). The SH1 and SH2 helices are believed to play a key role in the conformational changes that occur in the myosin head during force generation coupled to ATP hydrolysis. X-ray crystallographic studies suggest that during the power stroke, the light-chain-binding domain (LCBD) swings relative to the catalytic/ATP-binding domain. The pivot point of this swinging motion is considered to be in the vicinity of the SH1-SH2 helix (18). Not surprisingly, in vitro and in vivo modifications of the SH1 helix including cross-linking of SH1-SH2 groups or alteration/substitution of SH1/SH2 have been shown to disrupt the mechanical function of the myosin motor domain (19,20). Thus, studies investigating myosin structure and function have demonstrated that the functional integrity of myosin is critically dependent upon its flexibility at the SH1–SH2 helix. The strict conservation of this linker region among myosin II subtypes within and between species further underscores its functional importance. The R705H mutation in the DFNA17 family may cause an altered conformation of the SH1 helix that a ects its flexibility and movement, thus disrupting the mechanical function of the motor domain.
V.MYH9 EXPRESSION IN THE MAMMALIAN COCHLEA
Localization of MYH9 expression in situ in the mammalian cochlea has particular importance for understanding and interpreting the histopathology associated with its dysfunction. Within the rat cochlea, MYH9 expression was localized in three distinct tissue types: the organ of Corti, the subcentral region of the spiral ligament, and Reissner’s membrane (Fig. 5) (3). Within the organ of Corti, the MYH9 expression was identified throughout the outer hair cells including the cuticular plate. The CSD histopathology of the a ected DFNA17 proband demonstrated a collapsed Reissner’s membrane, a two-cell-layer-wide membranous barrier that forms the upper boundary of the cochlear duct. MYH9 dysfunction in the Reissner’s membrane may contribute to its compromised cytoarchitecture, thus disturbing cochlear fluid homeostasis and a ecting cochlear function. The cochlear tissue con-
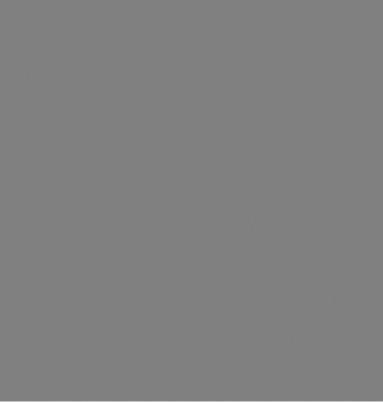
MYH9 |
173 |
Figure 5 (A) Within the rat cochlea, MYH9 expression was localized in three distinct tissue types: the organ of Corti, the subcentral region of the spiral ligament, and Reissner’s membrane. (B) Control cochlear section with competing peptide shows no staining.
spicuous by its absence of MYH9 expression is the stria vascularis, the lateral wall of the cochlear duct, and whose dysfunction is generally considered to be the cause of CSD. The absence of MYH9 expression is the stria vascularis suggests that this tissue type is not directly responsible for the underlying pathophysiology of CSD.
MYH9 is also expressed within the spiral ligament consisting of connective tissue made up of fibrocytes. These fibrocytes of the spiral ligament are classified according to the distinct isoforms of the Na+, K+- ATPase that they express as well as by their cytoskeletal components. The subcentric region of the spiral ligament, immnoreactive to the MYH9