
Учебники / Genetic Hearing Loss Willems 2004
.pdf324 |
Van Laer et al. |
II.LOCALIZATION AND IDENTIFICATION
To localize the gene responsible for the hearing loss in this family, DNA was collected from 68 members of one familial branch. Initially, linkage to DFNA1 and 2 was ruled out (9). After exclusion of other loci known at that time (DFNA3 and 4; DFNB1–3), a genome search was performed. Fiftyone percent of the genome was excluded before linkage was detected in band 7p15 on the short arm of chromosome 7. With genetic marker D7S673 a maximum lod score of 13.53 at 0% recombination was obtained. Haplotypes were constructed and key recombinational events delineated a candidate interval of 15 cM between the flanking markers D7S493 (telomeric side) and D7S632 (centromeric side). The locus was designated DFNA5, as it was the fifth locus for autosomal dominant hearing impairment (10).
Up to now, the Dutch family remains the only one linked to the DFNA5 locus. Therefore, a refinement of the candidate region could be accomplished only by performing linkage analysis on samples from additional familial branches, and by the analysis of additional polymorphic markers. This resulted in a reduction of the candidate region to less then 2 cM between the flanking markers D7S682 (telomeric side) and D7S1791 (centromeric side) (11). Additionally, a YAC-contig covering the complete candidate region was constructed (11,12). One expressed sequence tag (EST) that was derived from a cochlear cDNA library was positioned in the candidate region using the YAC-contig. The complete cDNA sequence of the corresponding gene was determined and it was designated candidate gene 1 (CG1). Extensive mutation analysis, however, could not reveal any disease-causing mutation (11).
A further reduction of the DFNA5 critical region to 600–850 kb between the flanking markers D7S3076 (telomeric side) and D7S1821 (centromeric side) was accomplished by the analysis of additional family members and by the development of new polymorphic markers from the candidate region (13) (Fig. 3). ESTs previously mapped in the vicinity of the DFNA5 candidate region were fine-mapped using the previously established YACcontig. Only four ESTs mapped in the candidate region, two of them belonging to one gene. Seventy to ninety percent of the candidate region was covered by BACs and PACs, which were completely sequenced as part of the Human Genome Project. By use of a combination of electronic exon trapping (Grail, Mzef, and Genscan), nucleic acid sequence database homology searches (Blast), and 5V-RACE, the full-length cDNA sequences of the genes corresponding to the ESTs were generated. Subsequently, mutation analysis was performed. In one of the genes present in the DFNA5 candidate region, a complex deletion/insertion mutation was identified in all a ected family members: a 1191-bp fragment of intron 7 was deleted and substituted with

DFNA5 |
325 |
Figure 3 The 600–850-kb DFNA5 candidate region and adjacent genetic markers. The genes identified in the candidate region are represented with bold arrows (LOC51678: MAGUK protein p55T—protein associated with Lins 2; DFNA5: autosomal dominant deafness type 5; OSBPL3: oxysterol binding protein—like 3). The genomic length of the genes is indicated underneath the arrows. The positions of the genetic markers are indicated with dashed arrows. D7S1838 and D7S1791, the two polymorphic markers preferred for linkage analysis, are indicated.
a 127-bp fragment derived from intron 8, which was inserted in the opposite direction and followed by a GCCCA stretch of unknown origin (Fig. 4). On the mRNA level, the mutation causes skipping of exon 8 (193 bp), resulting in a frameshift starting at amino acid 330, introducing an aberrant stretch of 41 amino acids followed by a stop codon. This intronic mutation does not a ect intron-exon boundaries or the branchpoint, but deletes five G-triplets at the 3V end of the intron. Evidence is growing that, in addition to the intronexon and the branchpoint consensus sequences, other sequences, in particular G-triplets, are involved in splice site selection. For this reason we assume that the deletion of five G-triplets at the 3V side of intron 7 causes skipping of exon 8. The mutation was never found in any of the 250 controls. The gene was designated DFNA5, as no physiological function could be deduced from extensive computational analysis. At the genomic level DFNA5 measures approximately 60 kb and contains 10 exons. DFNA5 encodes a protein of 496 amino acids with a molecular weight of 54,454 D. DFNA5 expression was demonstrated by RT-PCR both in the cochlear greater epithelial ridges and in the stria vascularis, as well as in every other tissue investigated so far (13).
III.FUNCTIONAL CONSIDERATIONS
DFNA5 most probably is identical to ICERE1 (inversely correlated with estrogen receptor expression gene 1), a gene identified during a di erential display study comparing gene expression in estrogen-receptor-positive and
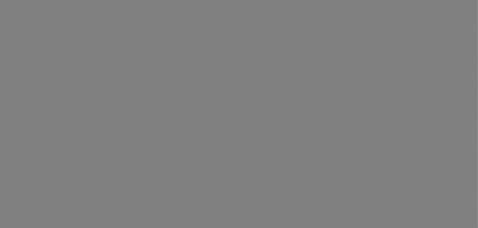
326 |
Van Laer et al. |
Figure 4 The genomic structure of DFNA5. Exons are represented by boxes, and introns by lines. The open reading frame is gray. The position of the exon borders, as derived from the genomic sequence of BAC RG385F02 (GenBank accession number: AC003093), is given above each exon. Exons and introns are drawn at di erent scales. The position of the CpG island is indicated. A detailed representation of the wild-type and the mutant sequence of exon 7, intron 7, and exon 8 is shown below the genomic structure of DFNA5. The putative branchpoint of intron 7 is indicated with an asterisk. In the wild-type chromosome the 5 XGGG-repeats at the 3’-side of intron 7 are represented by vertical lines. The direction of the gene is indicated with arrows. The inserted piece of intron 8 in the mutant chromosome is indicated with a hatched box. It is inserted in the opposite direction and followed by a GCCCA stretch of unknown origin. The borders of the deleted regions are also indicated with their respective nucleotide number. (From Ref. 13. Reproduced with permission.)
estrogen-receptor-negative breast carcinomas (14). This study showed that high expression of estrogen receptors is always accompanied by low levels of ICERE1/DFNA5. However, low expression levels of estrogen receptor are not su cient to obtain high expression of ICERE1/DFNA5. Furthermore, transfection of an estrogen-receptor-negative breast cancer cell line with estrogen receptor does not change the ICERE1/DFNA5 expression level, and transfection of ICERE1/DFNA5 in an estrogen-receptor-positive breast cancer cell line does not alter the estrogen receptor expression level (14). The interactions between ICERE1/DFNA5 and the estrogen receptor are therefore not straightforward. This could suggest the involvement of an intermediate factor (e.g., a transcription factor) that would induce one gene and repress the other. In conclusion, definite proof of the involvement of the ICERE1/DFNA5 gene in breast cancer pathogenesis is lacking and it
DFNA5 |
327 |
might be that the upregulation of ICERE1/DFNA5 in estrogen-receptor- negative breast carcinomas is a side e ect rather than a causative factor of tumor formation.
Another di erential display set up aimed at studying the mechanisms of acquired drug resistance in malignant melanoma by comparing melanoma cell lines that were either resistant or sensitive to di erent types of antineoplastic drugs (etoposide, vindesine, fotemustine, and cisplatin). Interestingly, DFNA5 expression levels are clearly decreased in melanoma cell lines that are highly resistant to etoposide. It was hypothesized that this decrease in DFNA5 expression level contributes to the etoposide resistance phenotype (15). To prove this hypothesis, the etoposide-resistant melanoma cell line was stably tranfected with DFNA5, and it was demonstrated that the transfected cell line was more susceptible to etoposide treatment than the untransfected melanoma cell line. Furthermore, etoposide exposure of DFNA5-transfected cells resulted in an increase of caspase-3-mediated programmed cell death, which might indicate that the resistant melanoma phenotype may be due to a decreased cellular susceptibility to trigger apoptotic events as a result of a decreased level of DFNA5 (16). It is unlikely that similar events take place in cochlear cells from DFNA5-mutated subjects, because apoptosis in terminally di erentiated cells di ers from apoptosis in dividing cells in many aspects. However, the data from this study (16) might indicate that DFNA5 is involved in the receptor/transporter function that is responsible for cellular drug uptake. Association to or regulation of a cellular receptor/transporter might also be a plausible role for DFNA5 in cochlear cells.
REFERENCES
1.Huizing EH, van Bolhuis AH, Odenthal DW. Studies on progressive hereditary perceptive deafness in a family of 335 members. I. Genetical and general audiological results. Acta Otolaryngol (Stockh) 1966; 61:35–41.
2.Huizing EH, van Bolhuis AH, Odenthal DW. Studies on progressive hereditary perceptive deafness in a family of 335 members. II. Characteristic pattern of hearing deterioration. Acta Otolaryngol (Stockh) 1996; 61:161–167.
3.Huizing EH, Odenthal DW, van Bolhuis AH. Results of further studies on progressive hereditary sensorineural hearing loss. Audiology 1972; 12:261–263.
4.Huizing EH, van den Wijngaart WSIM, Verschuure J. A follow-up study in a family with dominant progressive inner ear deafness. Acta Otolaryngol (Stockh) 1983; 95:620–626.
5.van den Wijngaart WSIM, Verschuure J, Brocaar MP, Huizing EH. Follow-up study in a family with dominant progressive hereditary sensorineural hearing impairment. I. Analysis of hearing deterioration. Audiology 1985; 24:233– 240.
328 |
Van Laer et al. |
6.van den Wijngaart WSIM, Huizing EH, Niermeijer MF, Verschuure J, Brocaar MP, Blom W. Follow-up study in a family with dominant progressive hereditary sensorineural hearing impairment. II. Clinical aspects. Audiology 1985; 24:336– 342.
7.De Leenheer EMR, van Zuijlen DA, Van Laer L, Van Camp G, Huygen PLM, Huizing EH, Cremers CWRJ. Further delineation of the DFNA5 phenotype. Results of speech recognition tests. Ann Otol Rhinol Laryngol 2002; 111:639– 641.
8.De Leenheer EMR, van Zuijlen DA, Van Laer L, Van Camp G, Huygen PLM, Huizing EH, Cremers CWRJ. Clinical features of DFNA5. Adv Oto-Rhino- Laryngol 2002; 61:53–59.
9.Coucke P, Van Camp G, Djoyodiharjo B, Smith SD, Frants RR, Padberg GW, Darby JK, Huizing EH, Cremers CWRJ, Kimberling WJ, Oostra BA, Van de Heyning P, Willems PJ. Linkage of autosomal dominant hearing loss to the short arm of chromosome 1 in two families. N Engl J Med 1994; 331:425–431.
10.Van Camp G, Coucke P, Balemans W, van Velzen D, van de Bilt C, Van Laer L, Smith RJH, Fukushima K, Padberg GW, Frants RR, Van de Heyning P, Smith SD, Huizing EH, Willems PJ. Localization of a gene for non-syndromic hearing loss (DFNA5) to chromosome 7p15. Hum Mol Genet 1995; 4:2159–2163.
11.Van Laer L, Van Camp G, van Zuijlen D, Green ED, Verstreken M, Schatteman I, Van de Heyning P, Balemans W, Coucke P, Greinwald JH, Smith RJH, Huizing EH, Willems PJ. Refined mapping of a gene for autosomal dominant progressive sensorineural hearing loss (DFNA5) to a 2-cM region, and exclusion of a candidate gene that is expressed in the cochlea. Eur J Hum Genet 1997; 5:397–405.
12.Van Laer L, Van Camp G, Green ED, Huizing EH, Willems PJ. Physical mapping of the HOXA1 gene and the hnRPA2B1 gene in a YAC contig from human chromosome 7p14–p15. Hum Genet 1997; 99:831–833.
13.Van Laer L, Huizing EH, Verstreken M, van Zuijlen D, Wauters JG, Bossuyt PJ, Van de Heyning P, McGuirt WT, Smith RJH, Willems PJ, Legan PK, Richardson GP, Van Camp G. Nonsyndromic hearing impairment is associated with a mutation in DFNA5. Nat Genet 1998; 20:194–197.
14.Thompson DA, Weigel RJ. Characterization of a gene that is inversely correlated with estrogen receptor expression (ICERE-1) in breast carcinomas. Eur J Biochem 1998; 252:169–177.
15.Grottke C, Mantwill K, Dietel M, Schadendorf D, Lage H. Identification of di erentially expressed genes in human melanoma cells with acquired resistance to various antineoplastic drugs. Int J Cancer 2000; 88:535–546.
16.Lage H, Helmbach H, Grottke C, Dietel M, Schadendorf D. DFNA5 (ICERE-1) contributes to acquired etoposide resistance in melanoma cells. FEBS Lett 2001; 494:54–59.
22
COCH
Nahid G. Robertson and Cynthia C. Morton
Brigham and Women’s Hospital and Harvard Medical School, Boston, Massachusetts, U.S.A.
I.IDENTIFICATION OF COCH: ORGAN-SPECIFIC APPROACH
COCH (coagulation factor C homology) was identified as a novel sequence isolated initially as a partial transcript from a second-trimester human fetal cochlear (membranous labyrinth) cDNA library (developmental ages 17–19 weeks) (1). Its identification was through an organ-specific approach to gene discovery in the auditory system. Subsequent chromosomal mapping of expressed sequences in the inner ear from this method provides candidate genes for deafness disorders localized in coincidental regions.
To isolate genes expressed preferentially or specifically in the inner ear, the cochlear cDNA library was first subtracted against transcripts of the brain (cortex), which is a highly complex tissue in both number and diversity of mRNAs. Subtractive hybridization reduces the number of housekeeping or other common transcripts and enriches for cochlear-specific messages. For further enrichment, subtraction of the cochlear clones was followed by di erential screening, whereby a number of both known and novel cochlear transcripts were identified (1). One novel cDNA isolated was originally designated as Coch-5B2 (1) and later renamed COCH (GenBank accession number AF006740), based on sequence similarity of the domain of COCH that harbors all of its known disease-causing mutations to a region of Limulus (horseshoe crab) coagulation factor C (2). Subtractive hybridization techniques using a chicken inner ear cDNA library also yielded Coch as one of the di erentially expressed transcripts in the auditory system (3).
329
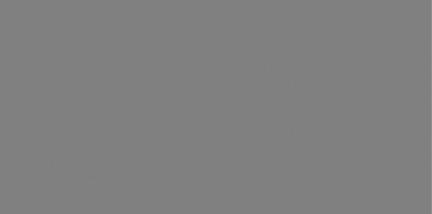
330 |
Robertson and Morton |
II.EXPRESSION OF COCH MRNA AND PROTEIN
Northern blot analysis (Fig. 1) of human COCH on di erent organs (1,4), including fetal cochlea, vestibule (not shown), brain (cerebral cortex), eye, kidney, liver, spleen, skeletal muscle, lung, skin, thymus, adrenal, small intestine, and cartilage, revealed the presence of very high levels of COCH transcripts (f2.0, 2.3, and 2.9 kb) only in the cochlea and vestibule, confirming its di erential expression in the inner ear. Very faint COCH expression is detected in fetal eye and brain, and adult skeletal muscle, with no detectable hybridization in any other organs tested. Northern blots of adult mouse tissues show messages of approximately 2.0 and 2.5 kb, consistent in size with two mouse cDNAs isolated with two polyadenylation sites, and of similar size to two of the three human messages. In the mouse, a somewhat wider range of expression is seen, including the spleen, brain (cerebrum), thymus, cerebellum, and medulla, and very faint levels in eye and lung (4). The significance of these di erences in expression between the two species is not known, but may represent alternative functions of the spleen in hematopoiesis in mice as compared to humans.
Figure 1 Autoradiograph of Northern blot of 10 Ag of total RNAs extracted from human fetal cochlea, brain (cortex), liver, spleen, skeletal muscle, kidney, lung, skin, thymus, adrenal, small intestine, eye, sternal cartilage, and cultured fibroblasts hybridized with COCH. Di erential expression of COCH in the cochlea as compared to other tissues tested is seen, represented by high levels of three transcripts (f2.0, 2.3, and 2.9 kb in size) in the cochlea; very faint hybridization is detected in brain and eye. Transcripts are indicated by arrowheads; the positions of 28S and 18S rRNAs are marked by lines. (From Ref. 1.)
COCH |
331 |
Furthermore, diverse expression patterns of COCH in humans and mice may indicate some di erence in the function of this gene in the two species during the developmental stages examined. Mapping in the mouse (4) shows localization of Coch to mouse chromosome 12 within a region of homologous synteny with the human map assignment on chromosome 14 (see Sec. IV A).
The expression profile of COCH throughout di erent organs of the body indicates that although COCH mRNA is not found exclusively in the inner ear, it is present at much higher levels in the cochlea and vestibule than all other organs examined. A recent proteomic analysis of bovine inner ear
(5) revealed that the COCH protein, cochlin, constitues approximately 70% of proteins isolated from the inner ear and represents the most abundantly detected protein by this analysis. The finding of large amounts of cochlin is in agreement with the high levels of COCH mRNA expression, and may also be a reflection of the relative stability of the protein. This study (5) further revealed the presence of three di erently sized isoforms (f40, 44, and 63 kD) of cochlin, as well as di erently charged isoforms, represented by 16 unique spots on 2D gel electrophoresis. The di erently sized isoforms of cochlin may arise from mRNA splice variants, or could result from posttranslational proteolytic processing. These data also suggest that cochlin may undergo other posttranslational modifications, such as glycosylation, phosphorylation, and/or deamination.
III.SEQUENCE ANALYSIS AND STRUCTURE OF COCH
A.cDNA and Deduced Amino Acid Sequences
Full-length protein-coding cDNAs for COCH (2,4) have been isolated in three species: human, mouse, and chicken (GenBank accession numbers AF006740, AF006741, and AF012252). Sequence comparison (Fig. 2) reveals a high degree of cross-species conservation of COCH in the coding region, showing 94% and 79% amino acid identity in the open reading frame (ORF) of the human to the mouse and chicken sequences, respectively. This homology drops abruptly after the translation stop codon, indicating lack of conservation in the 3V untranslated region of the gene. In all three species, the predicted encoded proteins are almost the same size. The deduced amino acid sequence of human COCH shows an ORF of 550 amino acid residues; the mouse and chicken sequences show an ORF of 552 and 547 amino acids, respectively. The minor variation in size and the lowest degree of amino acid conservation in the three species is in the short N-terminal signal peptide, which would be cleaved o and absent in the mature protein.
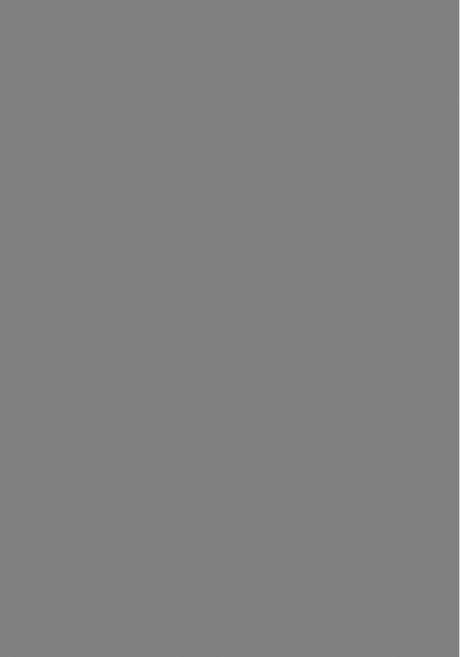
332 |
Robertson and Morton |
Figure 2

COCH |
333 |
B.Domain Structure
The deduced amino acid sequence of COCH (Fig. 2 and 3) shows a mosaic molecule consisting of a secretion signal peptide followed by two di erent types of domains that are also found in combination with other modules in proteins with diverse functions (2,4). Such protein modules may serve similar functions in di erent contexts, but have been shu ed and rearranged in the course of evolution. In juxtaposition with a unique set of domains, such modules may create novel associations. COCH, encoding the protein cochlin, shows a predicted signal peptide (SP), characteristic of secreted proteins, followed by a region homologous to a domain in factor C of Limulus (factor C–homologous domain, FCH), an intervening domain (ivd1), and two von Willebrand factor A–like domains (vWFA1 and vWFA2) separated by an intervening domain (ivd2) (6).
vWFA-like domains are regions approximately 200 amino acid residues in length, often present in multiple copies (and sometimes in tandem) within a single protein. A superfamily of genes with type A domains or ‘‘modules’’ includes proteins, all with ligand-binding properties, involved in various functions such as hemostasis (vWF), the complement (C2, Factor B) and immune systems (integrins such as LFA-1, Mac-1, VLA-1, VLA-2, p150, 95), and the extracellular matrix (cartilage matrix protein, collagens types VI, VII, XII, and XIV) (reviewed in Refs. 7–9). With the exception of integrins, which are transmembrane proteins, the only molecules currently identified to contain type A domains are secreted proteins. The largest number of type A domains known to date are found in the extracellular matrix (ECM), in proteins such as ColVIa3, which has 11 type A–like modules, making up almost all of the protein (10).
Figure 2 Alignment of the complete deduced amino acid sequence of COCH in human, mouse, and chicken, and one domain of factor C in Limulus (horseshoe crab). A high degree of cross-species homology, including conservation of all cysteine residues (dashed boxes), is seen. Dots indicate amino acid residues that are identical to those in human COCH, and dashes indicate gaps introduced to align the sequences. A predicted signal peptide in the beginning of the sequence is indicated by a line above the sequence. Five missense mutations, P51S, V66G, G88E, I109N, and W117R, found in the di erent DFNA9 families, are indicated. Amino acid residues mutated in DFNA9 show cross-species conservation and all reside in the FCH domain, homologous to a domain in Limulus factor C, containing four conserved cysteines. The di erent domains of cochlin are underlined and labeled as FCH (factor C homologous) and vWFA1 and vWFA2 (von Willebrand factor A–like domains 1 and 2). (From Ref. 2.)