
Учебники / Middle Ear Mechanics in Research and Otology Huber 2006
.pdf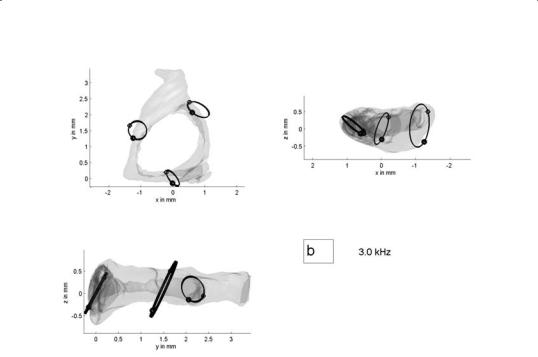
Fig. 3b At 3.0 kHz the displacement path (scaled by 75000) of the 3 points of Y83 is clearly elliptical. Note that the annular ligament is su ciently soft and wide (~ 14 μm) to allow in-plane footplate displacements (of the order of 10–8 m/Pa).
Displacementpathsfor3kHz(magnification75000,Fig.3b)arecompletely di erent and di er also from point to point. Vibration is no longer dominated by piston motion. If we measured the motion with a unidirectional interferometer and used di erent observation directions in the x,y-plane we would see no di erence in amplitude for the point on the anterior crus (circular path!), while for the 2 other points the amplitude would be large for observation in a direction along the long axis and small along the short axis of the elliptical path.
For elliptical motion paths the phase of the displacement component
98measured with a uniaxial interferometer will also depend on the observationangle(letusconsideranextremecase,andobserveapointthatfollows a circular path in the y-z plane; when we observe along the y direction we will get a motion component that is 90° out of phase with the component observed along the z direction).
3.2Change in gerbil stapes velocity component with observation angle
Using the experimentally determined rigid body velocities (rel. to ear canal pressure)foragerbilstapeswecalculatedwithEq.(1)the3-Ddisplacement components of a point at the middle of the stapes head (x=0, y=1.0 mm z=0 in Fig. 2). Using Eq. (2) we predicted the velocity amplitude and phase
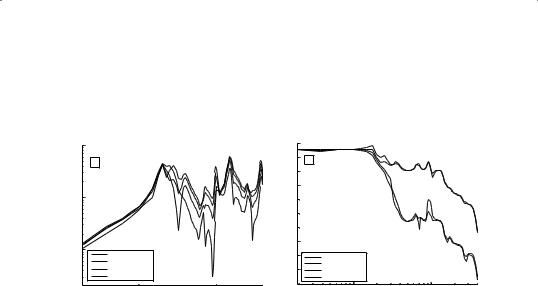
when this point is observed from di erent viewing angles (values inspired by study [11]: α =10° and β = 0, 20, 40 and 60°).
|
-3 |
|
300 |
|
10 |
|
|
|
a |
|
200 |
|
|
|
|
|
|
|
100 |
Pa |
|
veloc phase in deg. |
0 |
velocity amp. in m/s per |
10-4 |
-100 |
|
|
|||
|
-200 |
||
|
-300 |
||
|
-400 |
||
10-5 |
|
||
|
alfa=10; beta=0 |
|
-500 |
|
alfa=10; beta=20 |
|
|
|
alfa=10; beta=40 |
|
-600 |
|
alfa=10; beta=60 |
|
|
|
|
|
-700 |
|
103 |
104 |
|
frequency in Hz
b |
|
alfa=10; beta=0 |
|
alfa=10; beta=20 |
|
alfa=10; beta=40 |
|
alfa=10; beta=60 |
|
103 |
104 |
frequency in Hz
Fig. 4 Predicted amplitude (4a, left panel) and phase (4b, right panel) for the velocity component (in the direction of observation) of a point at the middle front of the gerbil stapes head, when 4 di erent observation angles, α =10° and β = 0, 20, 40 and 60°, are assumed. Amplitude and phase are relative to ear canal pressure.
The amplitude curves (Fig. 4a) run almost parallel up to 1.5 kHz; at higher frequencies this is no longer true. Top to bottom, curves at most frequencies are in decreasing order of β, but in some frequency bands this order is not respected. Phases (Fig. 4b) are the same for all β for frequencies below 1.0 kHz, at higher frequencies phase di erences of 20 to 30° and an extra unwrapping step is seen with increasing β.
In the low frequency region changing β from 0° to 60° the amplitude is reduced less than predicted by the cosine factor (cos60°=0.5). Changing the observation angle introduces contributions from the x and z translation components compensating for the reduction in the y-component.
At high frequencies we found vibration amplitude measured at observation angle β=60° that was even smaller than the 0.1 of the actual piston 99 component and cosine correction will not work.
For the human ear the piston-like motion is quite evident at low frequencies (Fig. 3) and a cosine correction works well below 1.5 kHz.
In general, if stapes motion is measured at an angle β to the piston axis over a wide frequency range and piston motion is estimated using a cosinecorrection,largeerrorsareintroducedduetothepresenceofmultiple modes of vibration.

Acknowledgments
We thank S. Merchant and J.J. Rosowski of Mass. Eye and Ear Infirmary (Boston, USA) for helping with the human temporal bones and M. Ravicz (same institute) for communicating typical α and β angles. Supported by NIH/NIDCD, Capita Fund, FSR (Flanders, Belgium) and RAFO (Univ. Antwerp).
References
1.W. Decraemer, S. Khanna and W. Funnell, “Measurement and modeling of the three-dimensional vibration of the stapes in cat,” Proc. Rec. Developments in Auditory Mechanics, World Scientific, 36–43 (1999)
2.W. Decraemer, S. Khanna, O. de la Rochefoucauld, W. Dong and E. Olson, “Is the scala vestibuli pressure influenced by non-piston like stapes components? An experimental approach,” Proc. Auditory Mechanisms: Processes and Models, Portland
491–494 (2005)
3.E. Overstreet, C. Richter, A. Temchin, M. Cheatham and M. Ruggero, “Highfrequency sensitivity of the mature gerbil cochlea and its development,” Audiology and Neuro-Otology, 8, 19–27 (2003)
4.J. Rosowski, M. Ravicz, S. Teoh and D. Flandermeyer, “Measurement of middleear function in the Mongolian gerbil, a specialized mammalian ear,” Audiology and Neuro-Otology, Vol. 4, No. 3–4, 129–136 (1999)
5.T. Ren, “Reverse propagation of sound in the gerbil cochlea,” Nature Neuroscience, 7 (4), 333–334 (2004) and private communication.
6.K. Heiland, R. Goode, M. Asai and A. Huber, “Human temporal bone study of stapes footplate movement,” Am. Journ. Otology 20, 81–86, (1999)
7.N. Hato, S. Stenfelt and R. Goode, “Three-dimensional stapes footplate motion in human temporal bone,” Audiology and Neuro-Otology, 8, 140–152 (2003)
8.H.KurokawaandR.Goode,“Soundpressuregainproducedbythehumanmiddle ear,” Otolaryngology – Head and Neck Surgery, 349–365 (1995)
1009. A. Huber, T. Linder, M. Ferrazzini, S. Schmid, N. Dillier, S. Stoeckli and U. Fish, “Intraoperative assessment of stapes motion,” Ann. Otol. Rhinol’ Laryngol. 110, 31–35, (2001)
10. W. Chien, M. Ravicz, S. Merchant and J. Rosowski, “The e ect of methodological di erences in the measurement of stapes motion of live and cadaver ears,”
Abstract #925 of the 25th Midwinter meeting of the Assoc. Res. in Otolaryngology (ARO), New Orleans, USA (2005)
11. W. Decraemer, S. Khanna and J. Dirckx, “The integration of detailed 3-dimensi- onal anatomical data for the quantitative description of 3-dimensional vibration of biological structures. An illustration from the middle ear,” Proc. 5th Internat. Conf. on Vibration Measurements by Laser Techniques: Advances and Applications, SPIE Proc. Series, Vol. 4827, 148–158 (2002)

12. W. Decraemer and S. Khanna, “Measurement, visualization and quantitative analysis of complete three-dimensional kinematical data sets of human and cat middle ear,” Proc.3rd Symposiumonmiddleearmechanicsinresearchandotology,World Scientific, 3–10, (2004)
13. S. Khanna, C. Koester, JF. Willemin, R. Dändliker and H. Rosskothen, “A noninvasive optical system for the study of the function in the inner ear in living animals”, in Selected papers on Coherence – Domain Methods in Biomedical Optics Valery Tuchin Ed. SPIE Vol. 2732, 66–81, (1996)
101

EXPERIMENTAL INVESTIGATIONS ON THE FUNCTIONAL EFFECT OF OSSICULAR JOINT FIXATION
Ch. Offergeld, M.D.1,3, N. Lazurashvili, M.D.2,3, M. Bornitz, M.Sc.3, Th. Beleites, M.D.2,3, Th. Zahnert, M.D.2,3
1 Dept. of Otorhinolaryngology, Head and Neck Surgery, University of Freiburg, Germany (Chairman: Prof. Dr. Dr. h.c. R. Laszig)
2 Dept. of Otorhinolaryngology, Head and Neck Surgery, University of Dresden, Germany
3 Middle Ear Laboratory, Dept. of ORL, HNS, Dresden Medical School, University of Dresden, Germany (Chairman: PD Dr. Dr. h.c. Th. Zahnert)
Corresponding author: Christian Offergeld, M.D., Dept. of Otorhinolaryngology, Head and Neck Surgery, Freiburg Medical School, University of Freiburg, Killianstrasse 5, 79106 Freiburg, Germany
Phone: 49+761+2704212, Fax: 49+761+2704111 Email: offergeld@hno.ukl.uni-freiburg.de
In the normal middle ear, stapes displacement during atmospheric pressure changes is physiologically reduced by the gliding ossicular joints. In fixed ossicular joints, static pressure is assumingly transfered directly towards the inner ear. Therefore unphysiological movements could eventually initiate exceeding displacements and consecutively lead to a risk for inner ear function.
102 Experimental investigations were performed in order to evaluate the dynamic and quasi-static e ect of ossicular joint fixation on the middle ear. Acoustic transfer characteristics and stapes displacement were determined in 12 fresh temporal bone specimens.StapesvibrationamplitudewasmeasuredusingLaser-Doppler-Vibrometry (LDV) before and after stepwise artificial fixation of the ossicular joints; the same applied to measurements of stapes displacement during standardized pressure changes in the external ear canal.
In comparison to a regular middle ear, fixed ossicular joints demonstrated a minimal decrease of footplate vibration amplitude in the low frequency range whereas an improvement of the transfer characteristics of less than 10 dB was demonstrable in the middle and high frequency region. It furthermore shows that atmospheric pressure changes in the external ear canal lead to an unphysiological footplate displacement.

1. Introduction
In the normal middle ear, a mechanical protection system against exceeding quasi-static and dynamic pressure variations exists [1]. It is the movements of the incudo-malleal joint (IMJ) and incudo-stapedial joint (ISJ) that lead to a certain decoupling of the ossicular chain for protection purposes. Therefore an unimpeded gliding of the ossicular joints is necessary [2]. In cases of ossicular joint fixation (e.g. in tympanosclerosis/ after tympanoplasty) the ossicular chain allows only rigid movements. Assumingly pressurecoulddirectlybetransferedfromthetympanicmembranetowards theannularligamentcreatingexceedingdisplacementsofthefootplateinto the vestibulum. In consequence, inner ear disorders can be expected.
However, there are only few specific descriptions about the e ect of ossicularjointfixationonmiddleeartransfercharacteristicsduringchanges of atmospheric pressure [3,4]. It was the purpose of our experimental study to demonstrate dynamic and quasi-static consequences arising from fixation of the ossicular joints as it helps to understand the function of the reconstructed middle ear (e.g. prosthesis, glueing of ossicular chain).
2. Materials and Methods
We performed experiments in 12 temporal bone specimens without any middle ear pathology.
Fig. 1 demonstrates the experimental setup. A posterior tympanotomy including removal of the facial nerve was performed in order to gain access towards the oval window niche and the footplate. This access was needed for stapes vibration amplitudeand displacement measurements using LDV (Polytec OFV 3001/ OFV 302; Polytec Co., Waldbronn, Germany). The external ear canal was sealed after positioning of a silicone tube connected to a sound source (Praecitronic Co., Dresden, Germany)
and a pressure pump which was connected to a tympanometer (Phonak 103 Co., Stuttgart, Germany). Calibration of the sound source was defined for sound excitation of 94 dB at 1000 Hz SPL in the region of the ear drum.
The frequency range was defined between 125 Hz and 5 kHz. A probe microphone (Ethymotic Research Inc., Elk Grove Village, IL, USA) was placed through a temporary opening in the external ear canal next to the ear drum for control measurements. Continuous pressure changes were standardized from ± 400 daPa in the external ear canal.
Step 1 included the measurement of the stapes vibration amplitude in regular ossicular joints. Sound excitation was performed using multisinus excitation of 94 dB SPL in the range of 250 Hz until 5000 Hz. LDV-meas- urements of 3 standardized measuring points (P1–P3) on the footplate
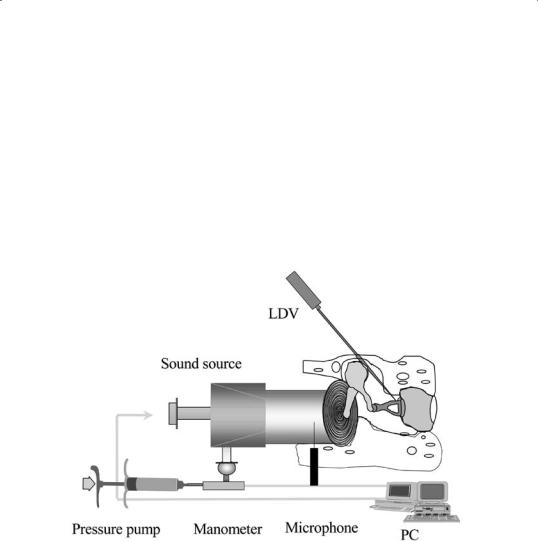
were realized. Afterwards we also performed measurements of the stapes displacement during static pressure variations.
Step 2 included the artificial fixation of the ossicular joints using cyanoacrylate glue (Histoacryl ). In 6 specimens, we started fixing the ISJ, in the other 6 cases we initiated with the IMJ. A droplet of Histoacryl was administered into the joint cleft and distributed properly within.
Step 3 was performed after fixation of the first joint by measurement of the footplate vibration amplitude. Consecutively step 4 included the same procedure after fixation of the second joint. Finally step 5 repeated step 1 after complete fixation of the ossicular chain.
Fig. 1 Schematic view of the experimental setup used for temporal bone investigations.
104
3. Results
Fig. 2 and 3 show exemplary temporal bone measurements in two specimens concerning footplate vibration amplitude during defined sound excitation in the external ear canal. The di erently marked curves indicate measurements of the central footplate region (P2) in normal and prepared temporal bones. Depending on the sequence of fixation, preparation started with either the IMJ or the ISJ before complete fixation of both ossicular joints.
In comparison to normal ossicular joints (Fig. 2, solid curve), fixation of the IMJ leads to an increase of the footplate vibration amplitude in the low and middle frequency region between 1000 Hz and 5 kHz (Fig.

2, dashed curve). This increase, mainly seen in the high frequency range equals less than 10 dB. A comparable improvement was also demonstrable after fixation of both joints including a shift in resonance frequency (Fig. 2, dotted curve). In contrast to the sole fixation of the IMJ it also showed a decrease of transmission of less than 5 dB in the low frequency range.
Very similar results were observed after stepwise fixation of the ISJ (Fig. 3, dashed-dottedcurve)andbothossicularjoints.Whilethelossofmiddleear transfer function in the low frequency region was almost the same, the increaseofvibrationamplitudewasmoreemphasizedinthehighfrequencies whenbothjointswerefixed(Fig.3,dottedcurve).Ineithercaseofsingleor combined fixation, a shift of the resonance frequency was demonstrable.
Fig. 2 Diagram demonstrating transfer characteristics of the middle ear in case of intact ossicular chain, fixed IMJ and complete ossicular fixation.
105
Fig.3Diagramevaluatingmiddleeartransfercharacteristicsincaseofintactossicular chain, fixed ISJ and complete ossicular joint fixation.

Fig. 4 demonstrates the static displacement of the stapes footplate under defined pressure changes before and after stepwise fixation of the ossicular joints.Thedashedcurve(IMJ-fixation)showsexceedingfootplatedisplace- ments in comparison to elevated footplate displacements in case of combined joint fixation (dotted curve). The greater displacement for negative pressure can even be more accentuated after fixation of one or both ossicular joints. Fig. 5 demonstrates a dotted curve (both joints fixed) with exceeding footplate displacement, a dashed-dotted curve (ISJ fixed) with decreasing footplate displacement in comparison to the intact ossicular chain (solid curve).
Fig. 4 Stapes footplate displacement after single (IMJ) and combined fixation of ossicular joints.
106
Fig. 5 Footplate displacement after single (ISJ) and combined ossicular joint fixation.

Duetoossicularjointfixation,thefootplatedisplacementisclearlyelevated in the majority of cases during application of pressure (± 400 daPa) in the external ear canal. Exceeding footplate displacements of up to 40 m compared to normal ossicles were observed. Although the increase of the footplatedisplacementisgreaterintotalfornegativepressure,positivepressure application also shows a 3–5fold elevation of the footplate displacement compared to untreated specimen.
4. Discussion
The “bu ering” system of the ossicular joints plays an essential role in the function of the normal middle ear determined by its mechanical components [5]. Thus, acoustic and quasi-static functions of the ossicular joints have to be distinguished explicitly from each other [6]. The ossicular joints perform typical gliding movements during changing static pressure or exceeding acoustical pressure loads while being functionally rigid during physiological acoustic sound transmission. It can be assumed that permanent ossicular joint rigidity could endanger the inner ear.
In our experimental results we demonstrated changes of the middle ear sound transfer function after artificial ossicular joint fixation, depending on localization and order of fixation (IMJ/ ISJ/ combined). This experimental study may also explain a possible pathologic pathway of an impaired regulation mechanism of the middle ear ossicular system. After increasing atmospheric pressure in the external ear canal an unphysiological displacement of the footplate was observed. Descriptions in literature evaluated maximum values of 10–30 m for footplate displacement [4] and served therefore as controls during experimental investigation. Our results definitely exceed the above mentioned values in case of ossicular joint fixation and therefore emphasize descriptions already evaluated by
Hüttenbrink [4] for IMJ-fixation. Circumstances like these have been pre- 107 viously rated as dangerous for the inner ear [7].
Atmosphericpressurechangesoccurverycommonlyandcouldtherefore mean serious pressure loads for the inner ear according to Moser’s description of the crucial role of the malleus-incus articulation [8]. Our results confirm the assumption of an unmitigated transfer of quasi-static pressure loads towards the footplate in case of partially or totally fixed ossicularjoints.Theyfurthermoredemonstratetheindividualsignificanceof the isolated and combined functions of IMJ and ISJ in middle ear transfer function as well as in pressure regulation. The IMJ plays hereby an important role as it seems to be able to partially compensate the quasi-static effects of a fixed ISJ. On the other hand we assume that the gliding motions