
Solid-Phase Synthesis and Combinatorial Technologies
.pdf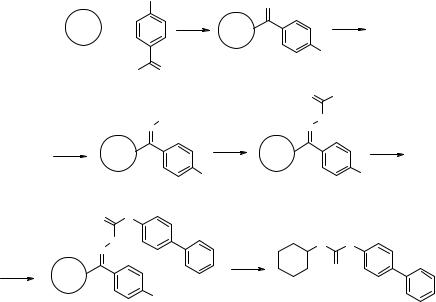
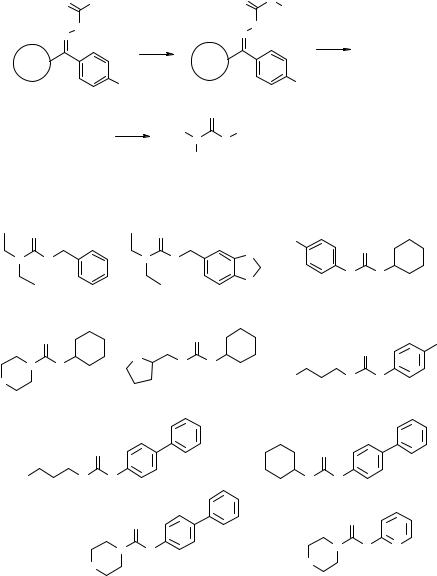
244 SYNTHETIC ORGANIC LIBRARIES: SOLID-PHASE DISCRETE LIBRARIES
|
|
|
|
|
H |
|
|
|
O |
Cl |
|
O |
N |
|
|
|
|
|
|
|
R1 |
|
|
|
O |
|
|
O |
|
|
|
|
N |
|
a |
N |
|
|
|
|
|
|
|
|
|
|
|
|
|
NO2 |
|
|
NO2 |
|
|
|
PhoximeTM |
|
|
|
|
|
|
|
|
b |
|
O |
|
|
|
|
|
R2 |
N N R1 |
|
|
|
|
|
|
|
a: R1NH2, dry DCM, rt, 16 hrs; |
||||
|
|
|
|
H |
b: R2R3NH, dry toluene, 80°C. |
||
|
|
|
|
R3 |
|
|
|
|
|
|
|
L6 |
|
|
|
|
|
Prepared Compounds (yield, purity): |
|
|
|||
O |
|
O |
|
OMe |
|
|
|
|
|
|
|
|
|
O |
|
N |
N |
|
N N |
O |
|
|
|
|
|
|
|
||||
|
|
|
|
|
|||
|
H |
|
H |
|
|
N |
N |
|
|
|
|
O |
|
||
|
|
|
|
|
H |
H |
|
|
98%, 93% |
|
98%, 76% |
|
88%, 87% |
||
|
O |
|
|
O |
|
|
I |
|
|
|
|
|
|
|
|
|
|
O |
N |
N |
|
|
O |
N |
N |
|
|
|
|||
|
|
|
|
||||
O |
H |
|
H |
H |
OMe |
N |
N |
|
|
95%, >90% |
|
H |
H |
||
86%, >90% |
|
|
|||||
|
|
89%, 96% |
|||||
|
|
|
|
||||
|
|
O |
|
|
|
O |
|
OMe |
N |
N |
|
|
N |
N |
|
|
H |
H |
|
|
H |
H |
|
|
90%, 92% |
|
|
91%, 98% |
|
||
|
|
O |
|
|
|
O |
|
|
|
|
|
|
|
|
|
|
|
N |
N |
|
|
N |
N N |
|
|
|
|
|
H |
||
|
|
|
H |
|
O |
|
|
|
|
O |
99%, 97% |
|
77%, 92% |
||
|
|
|
|
Figure 6.23 General structure of the SP urea library L6 and selected library individuals.
the corresponding oxime resin was prepared in a 100-g scale according to a published procedure (steps a and b) (173). This resin was converted to Phoxime resin (step c) (174), which was then to be reacted with primary amines to provide the first class of resin-bound monomer. In this scheme, the formation of the urea with simulta-
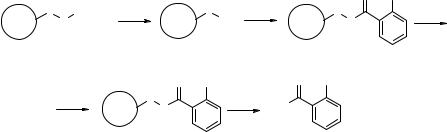
6.3 EXAMPLES OF SOLID-PHASE DISCRETE LIBRARY SYNTHESIS 245
neous cleavage from the support was the final step of the synthesis, which required optimization of the reaction temperature among other parameters. Eight 200-mg aliquots of Phoxime resin were placed in eight of the 24 independent reactors of the synthesizer and then coupled for 2 h at room temperature with 4-bipheny- lamine as a single standard (steps d and e, Fig. 6.22). The intermediate resin-bound carbamate 6.54 was successfully characterized. After the usual washing cycles, cyclohexylamine was added and the eight reaction mixtures were heated at different temperatures ranging from 50 to 120 °C (steps f1–f8). This experiment gave higher yields of recovered urea 6.55 with a temperature of 80 °C or above (Fig. 6.22). A small 10-member array L6 was prepared at 80 °C, and the optimized conditions proved to be quite general for the production of ureas 6.55; the structure and the quality of each library individual are reported in Fig. 6.23.
In the second example, optimization of a Suzuki coupling on SP was carried out. Two boronic acids were coupled with resin-bound iodide 6.56 (Fig. 6.24) at 90 °C for increasing reaction times of 1, 3, 6, and 10 h and the yields of the products 6.58 and 6.59 were calculated after cleavage of the product from the resin. The results are shown in Table 6.3. The less hindered thiophene boronic acid reacted completely after 1 h, while the more hindered tolyl derivative required 3 h to drive the reaction to completion. In this case it was found that while 3 h was an optimal length of time for both substrates, longer reaction times tended to decrease the reaction yields.
This type of SP synthesizer is ideal for more complex optimization studies in which multivariate optimizations, where more than one variable is changed at a time, could rapidly lead to optimized general SP reaction conditions. The high cost of this and other fully automated SP synthesizers may well be balanced by their regular application to library production and multivariate optimization in a dedicated combinatorial
O I
L |
Fmoc |
a |
L |
NH2 |
b |
L |
|
N |
|
|
|
N |
|
|
H |
|
|
|
|
H |
|
|
|
|
|
|
6.56 |
|
|
O |
Ar |
|
O |
Ar |
|
c1-c4 |
L |
|
d |
H2N |
|
|
|
N |
|
|
|
|
|
|
H |
|
|
|
|
|
|
6.57 |
|
|
|
|
|
|
|
|
|
6.58 |
Ar = 2-thienyl |
|
|
|
|
|
6.59 |
Ar = o-tolyl |
L = linker
a: 20% piperidine in DMF, rt; b: 2-IBzCOOH, DIC, HOBt, rt; c1-c4: ArB(OH)2, PdCl2(PPh3)2, Na2CO3, DME, NMP, 90°C, 1 hr (c1), 3 hrs (c2), 6 hrs (c3), 10 hrs (c4); d: TFA/H2O 95/5, rt, 30'.
Figure 6.24 SP automated chemistry assessment for the Suzuki coupling of supported aryl iodine 6.56 with boronic acids.
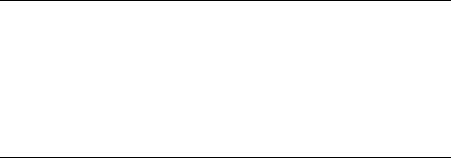
246 SYNTHETIC ORGANIC LIBRARIES: SOLID-PHASE DISCRETE LIBRARIES
TABLE 6.3 Time Dependence of SP Suzuki Coupling to Give Biaryl Amides 6.58 and 6.59
Final Compound |
Reaction Time (hr) |
Yielda |
Purityb |
|
|
|
|
6.58 |
1 |
90 |
100 |
6.59 |
1 |
99 |
90 |
6.58 |
3 |
95 |
100 |
6.59 |
3 |
100 |
98 |
6.58 |
6 |
91 |
99 |
6.59 |
6 |
96 |
98 |
6.58 |
10 |
67 |
100 |
6.59 |
10 |
62 |
99 |
aYield from 6.56. bCalculated by HPLC.
synthesis laboratory. A recent report (175) has presented the SP chemical assessment and the synthesis of a small array of 1,2-diamines in large quantities (>100 mg) using thesamesynthesizer.
6.4 NEW TRENDS IN SOLID-PHASE DISCRETE LIBRARY SYNTHESIS
6.4.1 Solid Supports for High-Throughput Organic Synthesis: Two-Dimensional SP Synthesis
Recently, the need for high-quality medium–large SP libraries of discretes has significantly increased, mostly because of problems encountered when trying to derive useful and reliable information from large SP libraries of pools, thus giving rise to general skepticism in many chemists. While we will address the issue of preparing quality pool libraries on SP in the next chapter, two emerging approaches for the synthesis of discrete libraries on dimensional supports will be presented here and in the next section. Resin beads, in fact, correspond to a zero-dimensional support in that the reactor is completely symmetrical and its portions undistinguishable; planar solid supports, covered in the section, have a bi-dimensional architecture which confers specific features to the SP as do mono-dimensional, thread-like supports covered in the next section.
Several years ago Fodor et al. (176) introduced the so-called VLSIPS (very large scale immobilized peptide synthesis) technique using a combination of α-amino acid–derivatized glass surface bearing amino groups protected with photocleavable protecting groups and photolithographic masks to direct the peptide synthesis. The principle of this technique is depicted in Fig. 6.25, where only the protecting groups X exposed to the light are removed and functionalized with the first α-amino acid. The production of a library using this method is shown in Fig. 6.26, where a spatially encoded library of 16 tetrapeptides is prepared using eight building blocks A–H (two
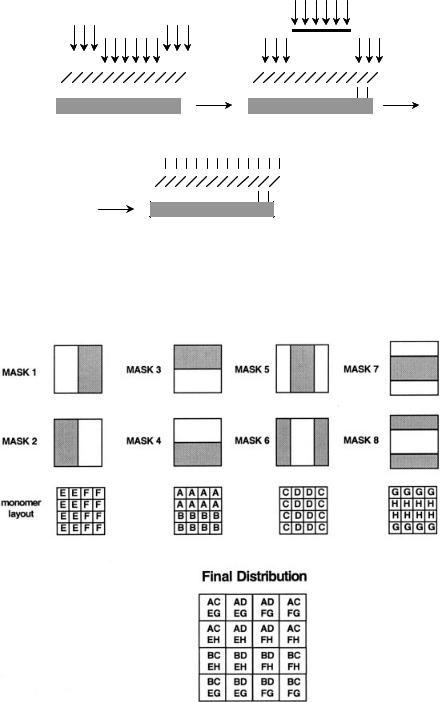
248 SYNTHETIC ORGANIC LIBRARIES: SOLID-PHASE DISCRETE LIBRARIES
for each coupling) and eight masking/coupling steps 1–8. VLSIPS has received a lot of attention for the synthesis of oligomers, especially for nucleotides, and has recently been reviewed (177, 178). Advantages of this method are the high throughput and the miniaturization of the synthetic device [e.g., a recent example reported the preparation of more than 10,000 oligonucleotides on chips just slightly larger than 1 cm2 (179)]; its application to materials science library will be thoroughly illustrated in Chapter 11.
A novel Maskless Array Synthesizer (MAS) based on an individually addressable digital micromirror array used in computer display projection systems has been recently reported for the synthesis of tens of thousands DNA sequences, each segregated on a 16 µm2 area (180). Its usefulness for small organic molecule SPS, though, has largely been prevented by the impossibility of cleaving the final products to test them in solution without the concomitant loss of spatial encoding and by the limited range of chemical conditions that are applicable to this method. Alternative cheaper planar supports such as paper (181, 182) or polyvinylidene fluoride (183) have also been reported for peptide synthesis. The latter showed good stability and greater flexibility under different reaction conditions when compared to glass.
Recently two groups (184–186) have reported a modification to the above methods in which portions of resin beads are sandwiched between two inert woven polypropylene sheets that are then fused together to give a so-called laminar solid phase. The use of this construct as a support for SP chemistry is shown in Fig. 6.27 in a hypothetical example using five sheets. Each sheet is divided into 50 small squares (typically 2 cm2) that are unequivocally marked with an inert ink (a–e for the sheets, 1–50 for the positions, step 1). Each sheet is then reacted with a different monomer of the first set (A1–A5, step 2), the columns of each sheet are cut and collated according to their position on each sheet, and each collated pool of columns is reacted with a different monomer of the second set (B1–B10, step 3). Finally, the columns are cut into squares, and those bearing the same initial numbers are pooled together, threaded, and reacted with the third monomer set (1–10, 11–20, 21–30, 31–40, 41–50, C1–C5, step 4, Fig. 6.27). By increasing the number of sheets and/or the squares in a sheet, it is possible to prepare tens of thousands of discretes using cheap materials. The other main advantage to this technique is the use of resin beads embedded in a fully permeable reaction support, which allows to transfer directly to laminar library production the chemical assessment performed on single resin portions in a conventional protocol.
A more sophisticated apparatus, called the chemical inkjet printer, has been reported by Lemmo et al. (187) and consists of a sheet of polypropylene (ChemSheet) divided into 2304 (48 × 48) shallow wells the bottoms of which are grafted with chemical functionalities. The principle with which SP parallel synthesis was carried out using this technique is identical to that of the laminar SP shown in Fig. 6.27 except that by using 48 ChemSheets and three sets of 48 monomers, more than 110,000 compounds could be prepared in parallel and then released into the wells. Each well, with a capacity of 8 µL, could produce around 5 nmol of final compound, and if we consider a classical solution dispensing system, with a dispensing time of 2 s per well, around 75 min would be necessary to fill all the wells with a single solution. Moreover,
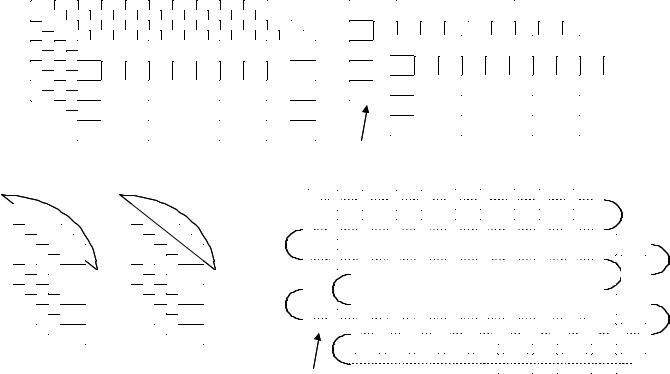
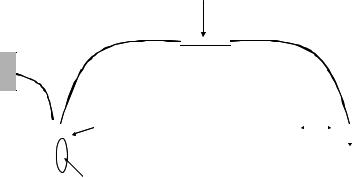
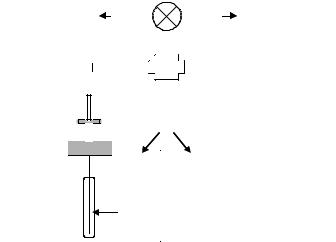
6.4 NEW TRENDS IN SOLID-PHASE DISCRETE LIBRARY SYNTHESIS 251
vacuum: |
gas: |
recovery |
delivery |
reagent chambers (2)
reagent |
reservoir |
(12 per |
chamber) |
Figure 6.29 Details of a reagent delivery manifold in an inkjet microdispenser.
common solvents for organic reactions have been demonstrated. The complete filling of a ChemSheet plate by the microdispenser was successfully performed in less than 10 sec, thus theoretically allowing the processing of hundreds of thousands samples in parallel in very short amounts of time. It is likely that this technique will be more widely used in the future, together with some other miniaturized systems described below, once they have been adapted to accept the normal range of reaction conditions used in parallel organic synthesis. A comprehensive review dealing with microdispensing instrumentation and protocols in drug discovery has recently been published (195).
6.4.2 Planar Solid Supports for High Throughput Organic Synthesis: Mono-Dimensional SP Synthesis
A mono-dimensional support for SPS has been presented recently by Schwabacher et al. (196). A flexible thread grafted with appropriate chemical functionalities is wrapped around a cylinder in a spiral layer (cylinder 1, step 1, Fig. 6.30), placing each thread turn at the same distance. Four parallel lines are drawn lengthwise along the cylinder, and a wax barrier is deposed with a hot glue gun to segregate each of the four cylinder regions. The first monomer set (four representatives) is coupled to each cylinder region, and cross-contamination is prevented by the wax barrier (Fig. 6.30). The thread is then unwrapped and prepared for the second combinatorial step, which his performed on a cylinder with a different diameter (cylinder 2, step 2, Fig. 6.30). The second monomer set (six representatives) is coupled as seen before, and each cylinder region has the same width as before, but the different cylinder dimensions and number of monomers cause the formation of different adducts in the thread regions (Fig. 6.30). The authors used a cotton thread grafted with an amine function, and performed the SP synthesis
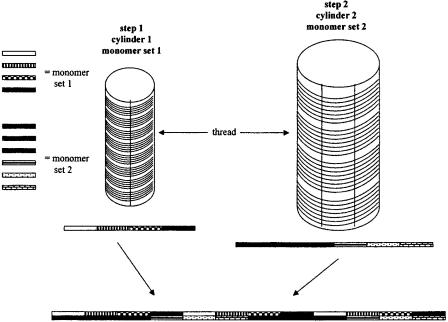