
Solid-Phase Synthesis and Combinatorial Technologies
.pdf8.3 PURIFICATION OF SOLUTION-PHASE LIBRARY INTERMEDIATES |
363 |
8.18); in this case, the basic tag allows the extraction of the product into aqueous acid and the same double-extraction procedure for the above example (steps a and b, Fig. 8.18) can be applied. Finally, the neutral product is detagged (step f, Fig. 8.18) and obtained as a pure sample, providing that detagging is performed without the generation of impurities. An example of a basic phase tag for liquid–liquid extraction has been recently reported (90).
Liquid–liquid two-phase extractions are sometimes suitable for parallel synthesis. Manual multichannel pipettes can handle a few tens of solutions and recover the desired liquid phase after the extraction. If numbers of individuals increase, partial or total automation is necessary and monoor multichannel robotic pipettes deliver solutions and withdraw either the top or the bottom phase of a liquid–liquid system with extreme accuracy, transferring it to other reaction vessels. They allow the sequential preparation of medium–large libraries but are very time consuming when large libraries of > 1000 members are involved.
8.3.3 An Example: Synthesis and Purification of an Iminodiacetic-Based Triamide Library
Cheng et al. (91) have reported the manual synthesis of a 960-member, flexible triamide library L6 made by decoration of an iminodiacetic acid–based scaffold. The structure of L6 and the synthetic scheme leading to the library are reported in Fig. 8.19. Sequential amide coupling using well-known peptide coupling procedures involving primary amines M1 (six representatives) and M2 (eight representatives) and carboxylic acids M3 (20 representatives) was considered trivial enough to avoid monomer rehearsal.
Treatment of the protected iminodiacetic acid 8.32 with EDC in DMF produced the anhydride 8.10, which was treated in situ with M1 to give intermediates 8.33 (steps a and b, Fig. 8.19). These were purified by washing with acid to remove the unreacted amines and any aqueous by-products (step c). Coupling with M2 using phosphorus (1-hydroxy-1H-benzotriazolo)tri-1-pyrrolidinylhexafluoro phosphate (PyBOP) and DIPEA in DMF produced 48 intermediates 8.34 (step d) that were purified by sequential acid and basic washings to remove unreacted amines (step c) and unreacted 8.33 (step e), respectively. Deprotection of the Boc carbamate (step f) and coupling with M3 produced 960 triamides (L6, step g) that were purified in the same manner as 8.34 (steps c and e, Fig. 8.19). The final compounds were obtained with overall yields varying from 10 to 71% in quantities of 30–150 mg with purity generally >95%.
Other methods are more suitable for the purification of medium–large solutionphase libraries (vide infra), but if small arrays are prepared and partition between aqueous and organic phases is applicable, this method rapidly produces good-quality library compounds, either alone or in conjunction with other separation techniques.
8.3.4 Liquid–Liquid Extractions: Multiphase Systems
Recently, Horvath, and Rabai (89) have reported the use of fluorous biphasic systems as an alternative to aqueous biphasic systems for phase transfer catalytic processes in
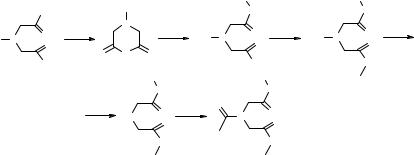
364 SYNTHETIC ORGANIC LIBRARIES: SOLUTION-PHASE LIBRARIES
|
|
Boc |
|
|
R1 |
|
|
R1 |
|
OH |
|
|
|
NH |
|
NH |
|
|
a |
N |
M1 |
|
|
|
M2 |
|
|
|
|
|
O |
O |
|||
Boc N |
O |
|
|
Boc |
N |
Boc N |
||
|
O |
O |
b,c |
|
|
O |
d,c,e |
O |
|
O |
|
|
|
|
|||
8.32 |
O |
|
8.33 |
OH |
8.34 |
NH |
||
OH |
8.16 |
|
|
|||||
|
|
|
R1 |
|
|
R1 |
NH |
R2 |
|
|
|
NH |
|
|
|
|
|
|
f |
|
O |
M3 |
O |
|
O |
|
|
|
HN |
|
N |
|
|
||
|
|
O |
g,c,e |
|
O |
|
||
|
|
|
R3 |
|
|
|||
|
|
8.35 |
NH |
|
|
NH |
|
|
|
|
|
|
|
|
|||
|
|
|
R2 |
|
L6 |
R2 |
|
|
|
|
|
|
|
|
|
|
960 discretes triamide library
a: EDC, DMF, rt, 1 hr; b: DMF, rt, 24 hrs; c: acidic extraction of impurities; d: PyBOP, DIPEA, DMF, rt, 25 hrs; e: basic extraction of impurities; f: 4N HCl, dioxane, rt, 45'; g: PyBOP, DIPEA, DMF, rt, 20 hrs.
M1 : primary amines and anilines (6)
M2 : primary and secondary amines, anilines (8)
M3 : carboxylic acids (20)
Figure 8.19 Synthesis and purification of the solution-phase, discrete triamide library L6 using liquid–liquid extraction.
which the aqueous phase interferes with water-sensitive reactions; Barthel-Rosa and Gladysz (92) have subsequently reviewd the use of both fluorous catalysts and reagents in fluorous media. Fluorous-tagged reagents/catalysts were prepared and optimized to become miscible with the fluorous phase and immiscible with the organic phase. Ogawa and Curran (93) extended the application of this method through the use of benzotrifluoride (BTF) as a mixed solvent that dissolves organic and fluorous reagents giving a homogeneous reaction mixture, followed by a two-phase, organic–fluorous liquid extraction to isolate the desired products. The same group (97–103) and others (94–96, 106–109) have also reported the synthesis of tagged catalysts (94–97), Sn-based (98–101), Si-based (102–103), and P-based (104–107) reagents and/or protecting groups for a variety of chemical transformations and of tagged ligands for organic reactions under fluorous experimental conditions (108, 109).
The organic–fluorous system is orthogonal to the aqueous and organic phases, so that a triphase liquid–liquid–liquid system is realizable (Fig. 8.20) in which the partition between three different layers can give very pure compounds if the affinity of reagents, catalysts, and products for each phase is clearly determined. Curran (110) was the first to foresee combinatorial applications for this extraction method, which relies on fluorous tags to label reagents, monomers, and/or reaction products and the partitioning of intermediates and final discretes into a specific layer; he has also summarized the current possibilities of fluorous reagents and techniques for solutionphase parallel synthesis in a recent review (111).
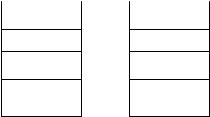
8.3 PURIFICATION OF SOLUTION-PHASE LIBRARY INTERMEDIATES |
365 |
|
organic |
aqueous |
|
aqueous |
organic |
|
fluorous |
fluorous |
|
light organic solvents |
halogenated |
|
(non-halogenated) |
organic solvents |
|
Figure 8.20 Liquid–liquid triphase extractions: aqueous/organic/fluorous mixtures.
Bior triphasic fluorous extraction protocols have been applied to the synthesis and purification of small arrays of isoxazolines and isoxazoles from tagged allyl alcohols and nitrile oxides (102), biaryls, and alkenylaryls from the Stille coupling of tagged tin reagents with organic halides or triflates (112, 113), using also microwave irradiation (114) and homoallylic alcohols from tagged stannanes and aldehydes (115). Recently a “fluorous switch” amine has been reacted with excess of isocyanates in an organic medium during the synthesis of an array of ureas (116); the fluorous by-prod- uct partitioned into the fluorous phase in a liquid–liquid biphasic extraction and was thus removed from the crude library discretes.
Unfortunately, this triphasic system is limited by the technical feasibility of handling single layers from a triphasic system, which is more complex than for the corresponding biphasic system, and the application of triphase extraction protocols to medium–large libraries is not practical. Curran and Luo have recently reported the so-called Light Fluorous Synthesis (LFS) (117): the lower fluorine content of fluorous tags used in LFS imparts better physico-chemical properties to the tagged compounds and makes more flexible both the reaction conditions and the purification-work up procedures. Alternative multiphase systems are also under investigation (118) and are likely to produce novel extraction phase systems. It can be expected that technological improvements in liquid handling/dispensing/separation protocols in the future will lead to wider use of aqueous/organic/fluorous extractions as a clean and effective purification method for combinatorial libraries in solution.
8.3.5 An Example: Synthesis and Purification of Amides and Dihydropyrimidines from Multicomponent Reactions
The synthesis of small arrays of organic compounds derived from multicomponent condensations was recently reported by Studer et al. (119). A 10-member amino acid amide library L7 (Fig. 8.21) was prepared using the fluorous Ugi (“Flugi”) condensation, and another 10-member dihydropyrimidine library L8 (Fig. 8.21) was prepared using the Biginelli (“Fluginelli”) condensation adapted to the fluorous phase. The key intermediates for library preparation were the silyl bromide 8.36, prepared from a fluorous iodide (120), and the acyl bromide 8.37 and the acid 8.38, prepared from an orthothiobenzoate (121), as shown in Fig 8.21. The structure of the fluorous tag was

366 SYNTHETIC ORGANIC LIBRARIES: SOLUTION-PHASE LIBRARIES
|
O R2 |
H |
|
|
|
|
|
|
|
|
|
|
O |
|
|
|
|
|
|
|
Ph |
O |
|
|
|
||||
|
|
N |
|
|
|
|
|
N |
NH |
|||||
|
N |
|
R3 |
|
|
|
|
|
|
|
|
|
||
|
R1 |
O |
|
|
|
|
|
|
|
O |
|
R2 |
|
R3 |
|
L7 |
|
|
|
|
|
|
|
|
|
|
|
||
|
|
|
|
|
|
|
|
|
L8 |
|
R1 |
O |
O |
|
|
10 discretes |
|
|
|
|
|
10 discretes |
|
|
|
||||
|
amide library |
|
|
|
|
|
|
|
|
|||||
|
|
|
|
dihydropyrimidine library |
|
|||||||||
|
|
|
|
|
|
|
||||||||
|
|
|
|
|
|
|
|
|
|
|
|
|
O |
|
|
|
COOH |
|
|
|
|
|
|
|
|
|
|
Br |
|
|
|
|
|
|
|
|
|
|
Rfh |
|
|
|
||
Rfh |
|
|
|
|
|
|
R |
|
|
|
|
|||
|
|
|
|
|
|
fh |
|
|
|
|
|
|||
Rfh |
Si |
|
|
|
|
|
|
|
Si |
|
|
|
|
|
|
|
|
|
|
|
|
|
|
|
|
|
|||
|
8.38 |
|
|
|
|
|
|
|
Rfh |
|
8.37 |
|
||
|
Rfh |
|
|
|
|
|
|
|
|
|
|
|
|
|
|
|
|
|
|
R |
|
|
|
|
|
Rfh |
|
|
|
|
|
|
a,b |
|
fh |
|
|
c |
|
|
Si |
|
|
|
|
Rfh |
I |
R |
Si |
R |
|
|
Rfh |
Rfh |
|
|
|||
|
|
|
|
fh |
|
|
|
|
|
|||||
|
|
|
|
fh |
H |
|
|
|
|
Br |
|
|
||
|
|
|
|
|
|
|
|
|
|
|
|
|
|
|
|
|
|
|
|
|
|
|
|
|
|
8.36 |
|
|
|
|
SPr |
|
|
|
|
|
|
SPr |
|
|
|
|
O |
|
|
|
|
|
|
|
|
|
|
|
|
|
|
|
|
|
|
|
Rfh |
|
|
|
|
SPr |
|
|
|
SPr |
||
|
SPr |
d,e |
|
|
|
SPr |
|
f |
|
R |
fh |
|||
|
SPr |
|
Rfh |
|
|
|
|
|
|
|
|
Rfh |
||
|
|
Si |
|
|
|
|
|
|
|
Si |
||||
Br |
|
|
|
|
|
|
|
|
|
|
|
|
||
|
|
|
Rfh |
|
|
|
|
|
|
|
|
Rfh |
||
|
|
|
|
|
|
|
|
|
|
|
|
|||
|
|
|
|
|
O |
|
|
|
|
|
|
|
O |
|
|
g |
Rfh |
|
|
|
Br |
h |
|
|
Rfh |
|
OH |
||
|
|
|
|
|
|
|
|
|
||||||
|
|
Rfh |
Si |
|
|
|
|
|
|
|
Rfh |
Si |
|
|
|
|
|
8.37 |
|
|
|
|
|
|
|
8.38 |
|||
|
|
|
Rfh |
|
|
|
|
|
|
Rfh |
||||
|
|
|
|
|
|
|
|
|
|
|||||
|
|
|
|
|
|
|
|
|
|
|
|
Rfh = C10F21CH2CH2-
a:Mg, Et2O, reflux, 2 hrs; b: HSiCl3, reflux, 16 hrs; c: Br2, FC-72, rt, 12 hrs; d: t-BuLi, Et2O, -78°C, 45';
e:8.36, BTF/FC-72, rt, 30'; f: AgNO3, BTF/Me2CO/THF/H2O, rt, 12 hrs; g: Br2, FC-72, rt, 3 hrs;
h:THF/BTF/H2O, rt, 12 hrs.
Figure 8.21 Fluorous-tagged Flugi (L7) and Fluginelli (L8) libraries : synthesis of tagged intermediates 8.36–8.38.
optimized to have a high content of fluorine atoms, which caused the complete partition of 8.37 and 8.38 into fluorous solvents but also an ethylene spacer between the organic moiety and the fluorous tag in order to avoid changes in substrate reactivity due to the electronic effect of the fluorocarbon chain.
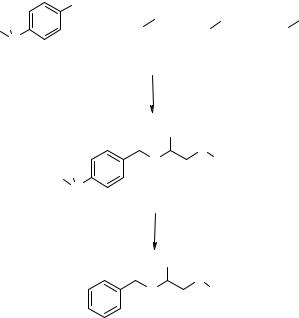
8.3 PURIFICATION OF SOLUTION-PHASE LIBRARY INTERMEDIATES |
367 |
The synthesis of array L7 is reported in Fig. 8.22. Compound 8.38 was reacted simultaneously with amines (M1, two representatives), aldehydes (M2, five representatives), and isonitriles (M3, two representatives) to give 10 compounds (not all the combinations were reacted). The reaction was performed in trifluoroethanol (TFE), another hybrid fluorous–organic solvent (step a, Fig. 8.22), and after evaporation of the TFE, the crude product 8.39 was purified by two-phase extraction between fluorous solvents and benzene (step b). After evaporation of the solvent, the fluorous tag was cleaved with TBAF (step c) and a triphasic extraction (step d, Fig. 8.22) was performed to remove the fluorosilane tag and acid 8.38–related impurities extracted into the fluorous layer. Excess TBAF and TBAF-related impurities partitioned into the acidic aqueous layer. Yields and purities of the synthetic protocol are reported together with the structures of the library members L7a–j in Table 8.2.
Synthesis of the array L8 is reported in Fig. 8.23. Bromide 8.37 was esterified with a urea alcohol (step a, Fig. 8.23) and purified by triphasic extraction (step b), giving pure 8.40 in the fluorous phase. Compound 8.40 was reacted with M1 (β-keto esters, four examples) and with M2 (aldehydes, three examples), as shown in step c, and then intermediates 8.41 were purified by two-phase fluorous/organic extraction (step d).
|
|
|
COOH |
|
|
|
|
|
|
|
|
|
|
|
|
|
|
|
|
|
R Rfh |
|
|
+ R1 |
|
|
NH2 + R2 |
CHO + R3 |
NC |
||||||||||||
fh |
Si |
|
|
|
|
|
|
|
|
|
|
|
|
|
|
|
M |
|
M3 |
|
|
|
|
|
|
|
|
M |
|
|
|
|
|
|
|
||||||
|
|
|
|
|
|
|
|
|
|
|
|
|
|
|
|
|||||
|
8.38 |
|
|
|
|
|
1 |
|
|
|
|
|
|
2 |
|
|
||||
|
Rfh |
|
|
|
|
|
|
|
|
|
|
|
|
|
|
|
|
|
|
|
|
|
|
|
|
|
|
|
|
|
|
a,b |
|
|
|
|
|
|
|
||
|
|
|
|
|
|
O |
|
|
|
R2 |
|
|
|
H |
|
|
|
|||
|
|
|
|
|
|
|
|
|
|
|
|
|
|
|
|
|
|
|
|
|
|
|
Rfh |
|
|
|
|
N |
|
|
|
|
|
N |
R3 |
organic phase |
|||||
|
|
|
|
|
|
|
|
|
|
|||||||||||
|
|
|
|
|
|
|
|
|
|
|
|
|||||||||
|
|
|
|
|
|
|
|
|
|
|
|
|
|
|
|
discarded |
||||
|
|
|
|
|
|
R1 |
|
|
|
O |
|
|||||||||
|
|
Rfh |
Si |
|
|
|
|
|
|
|
|
|
|
|||||||
|
|
|
8.39 |
|
|
|
|
|
|
|
|
|||||||||
|
|
|
|
|
|
|
|
|
|
|
|
|
||||||||
|
|
|
Rfh |
|
|
|
|
|
|
|
|
|
|
|
|
|
|
|
|
|
a: TFE, 90°C, 48 hrs; b: fluorous/ |
|
|
|
|
|
|
c,d |
|
|
|
|
|
|
|
||||||
|
|
|
|
|
|
|
|
|
|
|
|
|
|
|
|
|||||
organic extraction; c: TBAF, THF, rt, 30'; |
|
|
|
|
|
|
|
|
|
|
|
|
|
|
|
|
||||
d: fluorous/aqueous/organic extraction. |
O |
|
|
|
R2 |
|
|
|
|
|
|
|
||||||||
|
|
|
|
|
|
|
|
|
|
|
H |
|
|
|
||||||
|
|
|
|
|
|
|
|
|
|
|
|
|
|
|
|
|
|
|||
|
|
|
|
|
|
|
|
|
|
|
|
|
|
|
|
|
N |
|
fluorous phase |
|
|
|
|
|
|
|
|
|
|
|
|
|
|
|
|
||||||
|
|
|
|
|
|
|
|
|
N |
|
|
|
|
|
R3 |
|||||
|
|
|
|
|
|
|
|
|
|
|
|
|
|
discarded |
||||||
|
|
|
|
|
|
|
|
|
|
|
|
|
|
|
|
|||||
|
|
|
|
|
|
|
|
|
R1 |
|
|
O |
|
|
|
L7a-j
10 discretes amide library
Figure 8.22 Synthesis and purification of the solution-phase, discrete amide libraryL7 using fluorous aqueous/organic extraction protocols.

368 SYNTHETIC ORGANIC LIBRARIES: SOLUTION-PHASE LIBRARIES |
|
||||
TABLE 8.2 |
Yields and Purity of the Amide Discrete Library L7 |
|
|
||
|
|
|
|
|
|
Product |
R |
R |
R |
Yield (%) |
Purity (%)a |
|
1 |
2 |
3 |
|
|
L7a |
Benzyl |
Phenyl |
t-Butyl |
83 |
85 |
L7b |
Benzyl |
4-MeOPh |
t-Butyl |
81 |
87 |
L7c |
Benzyl |
c-Hexyl |
t-Butyl |
32 |
89 |
L7d |
n-Propyl |
c-Hexyl |
c-Hexyl |
99 |
>95 |
L7e |
Benzyl |
Phenyl |
c-Hexyl |
92 |
80 |
L7f |
n-Propyl |
i-Propyl |
c-Hexyl |
71 |
>95 |
L7g |
Benzyl |
i-Propyl |
c-Hexyl |
61 |
>95 |
L7h |
Benzyl |
c-Hexyl |
c-Hexyl |
84 |
>95 |
L7i |
Benzyl |
Ethyl |
t-Butyl |
75 |
93 |
L7j |
n-Propyl |
i-Propyl |
t-Butyl |
78 |
81 |
aDetermined by GC.
After removal of the solvent, the silyl tag was cleaved (step e) and the final triphasic extraction (step b, Fig. 8.23) gave the array L8 in the organic phase, removing TBAF-related impurities and the fluorous tag by extraction into the aqueous and fluorous phases, respectively. Yields and purities are reported together with the structures of the library individuals L8a–j in Table 8.3. A few compounds were obtained as mixtures, probably due to lower reactivity of corresponding monomers and occurrence of side reactions.
8.3.6 Solid-Phase Extractions
The partition of compounds between two phases can also happen when one of the phases is solid, that is, using solid-phase extraction (SPE). The rationale of this method is highlighted in Fig. 8.24. Assuming a hypothetical reaction crude where our product P occurs together with an impurity I, the mixture is dissolved in a solvent (phase 1, Fig. 8.24) and eluted through a column filled with a stationary phase (phase 2) that has a high affinity for P and no affinity for I. After elution and repeated washings with fresh phase 1, I is eluted while P remains adsorbed onto phase 2 (Fig. 8.24). The final step is the elution of the column with another liquid phase (phase 3), which disrupts the noncovalent interaction between P and phase 2 and has a strong affinity itself for P, obtaining the elution of P into phase 3 and its quantitative recovery (Fig. 8.24).
Ion-exchange chromatography is based on this principle and has been widely used for analytical purposes, while its application in organic synthesis has been limited to scattered examples, despite its simplicity and applicability to many separation problems. When the SP is an ion-exchange resin and the product has basic or acid functions, SPE should be considered the separation technique of choice. A number of examples from the recent literature serve to illustrate the applicability of the technique to parallel purification of a number of compounds.
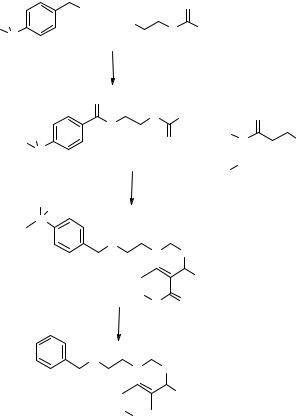
8.3 |
|
PURIFICATION OF SOLUTION-PHASE LIBRARY INTERMEDIATES 369 |
|||||||||||||||||||||||
|
|
|
|
O |
|
|
|
|
|
|
|
|
|
|
|
|
|
|
|
|
|
|
|
||
R Rfh |
|
|
|
|
|
|
|
Br |
|
|
|
|
|
|
|
|
O |
|
|
|
|
|
|||
|
|
|
|
|
|
|
|
|
|
|
|
|
|
|
|
|
|
|
|
||||||
|
|
|
|
|
|
|
|
|
|
|
|
|
|
|
|
|
|
|
|
|
|||||
|
|
|
|
|
|
|
|
+ HO |
|
N NH |
|
|
|
|
|||||||||||
fh |
Si |
|
|
|
|
|
|
|
|
|
|
|
|
|
|
|
|
|
|
|
|||||
|
|
|
|
|
|
|
|
|
|
|
|
|
|
|
|
H |
|
2 |
|
|
|
|
|||
|
|
|
|
|
|
|
|
|
|
|
|
|
|
|
|
|
|
|
|
|
|
|
|
|
|
|
Rfh 8.37 |
|
|
|
|
a,b |
|
|
|
|
|
|
|
|
|
|
|
|
|
|
|||||
|
|
|
|
|
|
|
|
|
|
|
|
|
|
|
|
|
|
|
|
|
|
|
|
|
|
|
|
|
|
|
|
|
|
|
O |
|
|
H |
|
|
|
|
|
|
|
|
|
||||
|
|
|
|
|
|
|
|
|
|
|
|
|
|
|
|
|
NH2 |
|
O |
O |
|||||
|
|
|
|
|
|
|
|
|
|
|
O |
|
|
N |
|
|
|
|
|||||||
|
|
Rfh |
|
|
|
|
|
|
|
|
|
|
|
|
+ |
R1 |
|
|
|
|
|||||
|
|
|
|
|
|
|
|
|
|
|
|
O |
O |
|
|
R2 |
|||||||||
aqueous, organic |
|
Rfh |
Si |
8.40 |
|
|
|
|
|
|
|
|
|
||||||||||||
phase discarded |
|
|
|
|
|
|
|
|
|
|
|
|
CHO M1 |
|
|
|
|||||||||
|
|
|
|
|
|
|
|
|
|
|
|
+ |
|
|
|
|
|||||||||
|
|
Rfh |
|
|
|
|
|
|
|
|
|
|
|
|
|
Ar |
|
|
|
||||||
|
|
|
|
|
|
|
|
|
|
|
|
|
|
|
|
M2 |
|
|
|
||||||
|
|
|
|
|
|
|
|
|
|
|
|
c,d |
|
|
|
|
|
|
|
|
|||||
|
|
|
RfhRfh |
|
|
|
|
|
|
|
|
|
|
|
|
|
|
|
|
|
|
|
|||
|
|
Rfh |
Si |
|
|
|
|
|
|
|
|
|
|
O |
|
|
|
|
|
|
|||||
|
|
|
|
|
|
|
|
|
|
|
|
|
|
|
|
|
|
|
|
|
|
||||
organic phase |
|
|
|
|
|
|
|
|
|
O |
|
|
N |
|
|
|
NH |
|
|
|
|
|
|||
|
|
|
|
|
|
|
|
|
|
|
|
|
|
|
|
|
|
|
|
||||||
|
|
|
|
|
|
|
|
|
|
|
|
|
|
|
|
|
|
|
|
|
|||||
|
|
|
|
|
|
|
|
|
|
|
|
|
|
|
|
|
|
|
|
|
|||||
|
|
|
|
|
|
|
|
|
|
|
|
|
|
|
|
|
|
|
|
|
|
|
|
|
|
discarded |
|
|
|
|
|
|
|
|
O |
|
|
|
|
|
|
|
|
|
|
|
|
|
|
||
|
|
|
|
|
|
|
|
R2 |
|
|
|
R3 |
|
|
|
|
|
||||||||
|
|
|
|
|
|
|
8.41 |
|
|
|
|
|
|
|
|
||||||||||
|
|
|
|
|
|
|
R1 O |
|
|
|
|
|
|
|
|
|
|||||||||
|
|
|
|
|
|
|
|
|
|
|
|
|
|
|
O |
|
|
|
|
|
|||||
|
|
|
|
|
|
|
|
|
|
|
e,b |
|
|
a: TEA, DMAP, dioxane/BTF, 35°C, 22 hrs; |
|||||||||||
|
|
|
|
|
|
|
|
|
|
|
|
|
|
|
|
|
|
|
|
b: aqueous/fluorous/organic extraction; |
|||||
|
|
|
|
|
|
|
|
|
|
|
|
|
O |
|
|
|
|
c: THF/BTF, 50°C, 72 hrs; |
|||||||
|
|
|
|
|
|
|
|
|
O |
|
|
|
|
|
|
|
|
d: fluorous/organic extraction; |
|||||||
aqueous, fluorous phase |
|
|
|
|
|
|
N |
NH |
|
e: TBAF, THF/TBF, rt, 30'. |
|||||||||||||||
|
|
|
|
|
|
|
|
|
|
|
|
|
|
|
|||||||||||
|
|
|
|
|
|
|
|
|
|
|
|
|
|
|
|
|
|
|
|
|
|
|
|||
|
|
|
|
O |
|
|
|
|
|
|
|
|
|
|
|
|
|
|
|||||||
discarded |
|
|
|
|
|
|
|
|
|
|
|
|
|
|
R3 |
|
|
|
|
|
|||||
|
|
|
|
|
|
|
|
|
|
|
R2 |
|
|
|
|
|
|
|
|
|
|
|
|
|
L8a-j R1 OO
10 discretes dihydropyrimidine library
Figure 8.23 Synthesis and purification of the solution-phase, discrete dihydropyridine library L8 using fluorous aqueous/organic extraction protocols.
Both Siegel et al. (122) and Lawrence et al. (123) have described automated systems for the purification of small arrays of amines and amides. A 48-member array of β-amino alcohols prepared from epoxides and amines was purified using SPE by Shuker et al. (124). Blackburn et al. (125) have described the purification of a 60-member 3-aminoimidazo[1,2-a]pyridine array obtained from a multiple-compo- nent condensation, and Bussolari et al. (126) purified a small array of phenylpropyl amines obtained from dihydrocoumarins and amines. A few applications where ion-exchange resins have been substituted with other solid phases have also recently appeared. For example, the purification of several carbohydrate arrays tagged as hydrophobic O-laurates using C18 silica producing up to 10–30 mg of >90% pure individuals was described by Nilsson et al. (127), and Curran et al. purified fluorous-
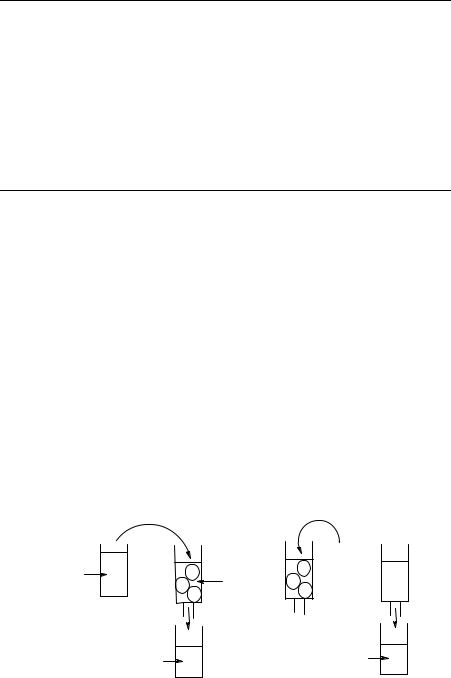
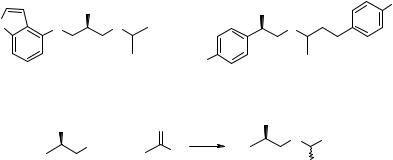
8.3 PURIFICATION OF SOLUTION-PHASE LIBRARY INTERMEDIATES |
371 |
8.3.7 An Example: Synthesis and Purification of a β-Amino Alcohol Library
Siegel et al. (130) reported the synthesis and purification by parallel SPE of a focused 96-member β-amino alcohol library L9 of potential adrenergic agonists or antagonists. The structures of known modulators (8.42, a β1- and β2-antagonist, and 8.43, a β2-agonist) are shown together with the synthetic scheme leading to L9 in Fig. 8.25.
The reductive amination of monomer set M1 (12 N-unsubstituted β-amino alcohols) with M2 (8 ketones) was driven to completion by a large excess of ketone, producing a complex mixture of library individuals, excess ketones, and alcohol by-products (step a, Fig. 8.25). A strong cation exchange resin was used to separate the basic L9 from the neutral impurities and the 96 methanolic solutions were eluted in parallel onto a block of 96 SPE cartridges. The block was then washed with fresh methanol, discarding the neutral impurities (step b). Final elution with methanolic ammonia (step c, Fig. 8.25) detached the desired products, which were characterized by HPLC, MS, and NMR, giving yields in the range of 21–99% and purities of 75–100%.
8.3.8 Chromatographic Methods
The physicochemical properties of the components of a mixture allow its separation using SPE by selective extraction of the target compound on SP, while the other
OH
|
OH |
H |
|
OH |
|
HN |
|
|
H |
|
|
O |
N |
|
N |
|
|
|
|
|
|||
|
8.42 |
|
HO |
8.43 |
|
|
OH |
|
O |
OH |
|
|
|
H |
|
||
|
NH2 |
+ |
a,b,c |
N R2 |
|
|
R |
||||
|
R1 |
|
R2 R3 |
1 |
|
|
|
|
|
|
|
|
M1 |
|
M2 |
L9 |
R3 |
|
|
|
|||
|
|
|
|
96 discretes |
|
β-aminoalcohol library
a: NaCNBH3, 10% AcOH/MeOH, rt, 24 hrs; b: SPE; c: elution with NH4OH.
M1 : aryl-substituted β-aminoalcohols (12)
M2 : acyclic and cyclic ketones (8)
Figure 8.25 Synthesis and purification of the solution-phase, discreteβ-amino alcohol library L9 using SPE.
372 SYNTHETIC ORGANIC LIBRARIES: SOLUTION-PHASE LIBRARIES
components of the mixture are eluted in the liquid phase. If all the mixture components have partial affinities for the SP and are retained with different strengths, the extraction process shifts toward more common chromatographic separations (88). This purification technique is by far the most popular in organic chemistry and direct or reversedphase column, analytical or preparative HPLC and GC are routinely used in most chemistry laboratories.
The well-assessed reputation of chromatographic separations has stimulated their use for combinatorial purposes, and HPLC coupled with MS is the method of choice for automated, high-throughput confirmation and quality control of library individuals. Its use for automated purification of solution-phase library discretes, or cleaved SP library samples, has also been widely investigated. Preparative HPLC can easily provide significant quantities of pure compounds in conjunction with various detection techniques (diode array detection (DAD), MS, ELS, CLND). Typically, this type of system can purify and characterize from 100 to 400 compounds per day, allowing the isolation of up to 75–100 mg of each crude sample (131–134) using multiple automated instruments with high flow rates (up to 70 mL/min) and short gradients (5–10 min; see also Section 6.2).
Despite some obvious limitations as a combinatorial separation method (large volumes of solvents required, cost of multiple automated instruments), the confidence of each synthetic and analytical chemist in LC methods and the extremely high technological level of available instrumentation will keep chromatographic methods among the most popular purification techniques for small–medium solution-phase libraries, especially when large amounts of each crude material are prepared.
8.4 SOLID-PHASE ASSISTED SOLUTION-PHASE LIBRARY SYNTHESIS AND PURIFICATION
8.4.1 General Considerations
The use of supported reagents, catalysts, or ligands for heterogeneous reactions in organic synthesis is becoming increasingly popular and has been reviewed recently (135, 136). The switch from single to multiple reactions for the preparation of combinatorial libraries in the solution phase with the assistance of supported materials has also been studied. Insoluble supported chemicals have a major advantage that mirrors the advantage of SP synthesis; that is, the excess of a supported reagent or of a side product derived from the supported reagent is separated from the product in solution with the same automated filtering/washing procedures described for SPS. This simple concept has driven combinatorial chemists to expand the use of solid-supported reagents for solution-phase library synthesis and purification. Further, some of these reagents may be used to sequester excess soluble reagents or impurities from the reaction mixture (supported scavengers) and others can capture, via covalent bond formation, intermediates or final library compounds prepared in solution (resin capture reagents). The use of these supported compounds in solution-phase combinatorial chemistry is described in what follows.