

Supplement F2: The Chemistry of Amino, Nitroso, Nitro and Related Groups.
Edited by Saul Patai Copyright 1996 John Wiley & Sons, Ltd.
ISBN: 0-471-95171-4
CHAPTER 9
Acidity and basicity
SILVIA BRADAMANTE
CNR, Centro di studio sulla Sintesi e Stereochimica di Speciali Sistemi Organici, c/o Dipartimento di Chimica Organica e Industriale, Universita` di Milano, via Golgi 19, 20133 Milano, Italia
Fax: 39 2 236.43.69; e-mail: BRADA@ICIL64.CILEA.IT
I. ABBREVIATIONS . . . . . . . . . . . . . . . . . . . . . . . . . . . . . . . . . . . . |
380 |
|
II. INTRODUCTION . . . . . . . . . . . . . . . . . . . . . . . . . . . . . . . . . . . . . |
380 |
|
III. GAS-PHASE SOLUTION CORRELATION . . . . . . . . . . . . . . . . . . . . |
381 |
|
A. Acidity and Basicity Scales in Solution . . . . . . . . . . . . . . . . . . . . . |
381 |
|
1. |
Proton transfer in water and DMSO . . . . . . . . . . . . . . . . . . . . . |
381 |
2. |
Complex formation with Lewis acids . . . . . . . . . . . . . . . . . . . . |
381 |
3. |
Hydrogen bonding . . . . . . . . . . . . . . . . . . . . . . . . . . . . . . . . |
381 |
B. Acidity and Basicity Scales in the Gas Phase . . . . . . . . . . . . . . . . . |
382 |
|
1. |
Scales relative to proton . . . . . . . . . . . . . . . . . . . . . . . . . . . . . |
382 |
2. |
Metal cation binding . . . . . . . . . . . . . . . . . . . . . . . . . . . . . . . |
384 |
3. |
Organometallic and organic cation binding . . . . . . . . . . . . . . . . |
385 |
4. |
Anion binding and electron affinities . . . . . . . . . . . . . . . . . . . . |
385 |
5. |
Cationic hydrogen bonds . . . . . . . . . . . . . . . . . . . . . . . . . . . . |
385 |
C. Gas Phase Solution Relationships . . . . . . . . . . . . . . . . . . . . . . . . |
385 |
|
IV. NITROGEN BASES . . . . . . . . . . . . . . . . . . . . . . . . . . . . . . . . . . . |
388 |
|
A. Amine Derivatives . . . . . . . . . . . . . . . . . . . . . . . . . . . . . . . . . . |
388 |
|
B. Amidines and Guanidines . . . . . . . . . . . . . . . . . . . . . . . . . . . . . . |
392 |
|
C. Amidates and Amides . . . . . . . . . . . . . . . . . . . . . . . . . . . . . . . . |
395 |
|
V. NITROGEN ACIDS . . . . . . . . . . . . . . . . . . . . . . . . . . . . . . . . . . . |
395 |
|
A. Structural Characterization of Metal Amides in the Solid State |
|
|
and in Solution . . . . . . . . . . . . . . . . . . . . . . . . . . . . . . . . . . . . . |
396 |
|
B. Amine Derivatives . . . . . . . . . . . . . . . . . . . . . . . . . . . . . . . . . . |
398 |
|
C. NH Bond Dissociation Energy . . . . . . . . . . . . . . . . . . . . . . . . . . . |
400 |
|
D. Carboxamides and Related Compounds . . . . . . . . . . . . . . . . . . . . . |
403 |
|
VI. HYDROXAMIC ACID AS AMPHOTERIC SYSTEMS . . . . . . . . . . . . |
405 |
|
VII. HETEROCYCLES . . . . . . . . . . . . . . . . . . . . . . . . . . . . . . . . . . . . |
407 |
|
VIII. NITRO DERIVATIVES . . . . . . . . . . . . . . . . . . . . . . . . . . . . . . . . . |
409 |
379
380 |
Silvia Bradamante |
|
A. Nitromethane Derivatives . . . . . . . . . . . . . . . . . . . . . . . . . . . . . . |
409 |
|
B. Structure of Nitroalkanes and their Conjugated Bases . . . . . . . . . . . |
411 |
|
C. Nitroaryl-substituted Methanes . . . . . . . . . . . . . . . . . . . . . . . . . . |
414 |
|
IX. REFERENCES . . . . . . . . . . . . . . . . . . . . . . . . . . . . . . . . . . . . . . . |
416 |
|
|
|
|
|
I. ABBREVIATIONS |
|
AN |
acetonitrile |
|
BDE |
bond dissociation energy |
|
CHA |
cyclohexylamine |
|
D(A H) |
bond dissociation energy |
|
DME |
dimethoxyethane |
|
DN |
donor number |
|
DSP |
dual substituent parameter |
|
EA(A ) |
electron affinity |
|
EG |
ethylene glycol |
|
EPA |
electron pair acceptor |
|
EW |
electron withdrawing |
|
EWG |
electron-withdrawing group |
|
FT-ICR |
Fourier transform ion cyclotron resonance |
|
GAPT |
generalized atomic polar tensors |
|
GB |
gas-phase basicity |
|
HBA |
hydrogen-bond acceptor |
|
HBD |
hydrogen-bond donor |
|
HPMS |
high-pressure mass spectrometry |
|
IBE |
intrinsic bond energy |
|
ICR |
ion cyclotron resonance |
|
IE(H) |
ionization energy |
|
ISM |
intersecting state model |
|
MCA |
methyl cation affinity |
|
NB |
nitrobenzene |
|
NMP |
N-methylpyrrolidine-2-one |
|
PA |
proton affinity |
|
PNS |
principle of nonperfect synchronization |
|
RSE |
radical stabilization energy |
|
TEP |
triethylphosphate |
|
II. INTRODUCTION
This review is an update of the contribution on analogous topics that appeared in previous volumes of this series1,2. It is not intended to be comprehensive, but rather a critical survey of the emerging research lines, views and re-interpretation of old facts under the stimulus of new experimental results. The last decade has seen an explosion of gas-phase chemistry with wide spread investigations of proton transfer reactions of various acids and bases. We felt it to be logical and convenient to treat simultaneously, in each specific sub-chapter, gas-phase and solution data to offer a wide aspect of the topic and facilitate connections between the two approaches. Therefore, the content of the review is organized in such a way that specific data on nitrogen bases and acids is preceded by preliminaries on the acidity and basicity scales, both in solution and in the gas phase. On the same grounds, we postponed the treatment of nitrogen acids to a discussion on structural aspects of
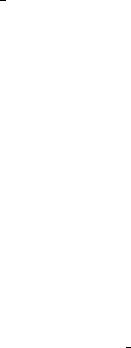
9. Acidity and basicity |
381 |
nitranions, both in the solid and in solution. Amphoteric and heterocyclic systems are treated on their own because they present both basic and acidic properties. The final sub-chapter is dedicated to recent advances in nitroalkanes.
III.GAS-PHASE SOLUTION CORRELATION
A.Acidity and Basicity Scales in Solution
1. Proton transfer in water and DMSO
Precise pKa values in water and mixed aqueous solutions, based on well-known experimental methods of measurement3, are available in the literature4. The problem of measuring pKa values for weak bases and acids has also been overcome5. Acting alternatively as a hydrogen-bond acceptor (HBA) or a hydrogen-bond donor (HBD), water strongly solvates both cations and anions.
In the case of non-HBD solvents, such as DMSO, the measured pKa values are absolute (that is, free from ion pairing) and can be directly compared with gas-phase acidities6; in addition, knowledge of the heats of ionization in DMSO7 allows the evaluation of a possible entropy effect when the two phases are compared. The mechanism of proton transfer between oxygen and nitrogen acids and bases in aqueous solution has been reviewed8.
2. Complex formation with Lewis acids
The Lewis acid base reaction leading to complex formation9,10 has been recently11 considered in relation to the role of solvation effects. Many scales of thermodynamic parameters have been suggested. The concept of donor number (DN) was proposed by Gutmann12, and defined as the H (kcal mol 1) for the interaction of a basic solvent with SbCl5 in 1,2-dichloromethane at room temperature:
B:(soln) |
C |
SbCl5(soln) |
! |
B: SbCl5(soln) |
1 |
|
|
|
|
|
Subsequently, DN has been defined according to the reaction
B:(soln) |
C |
BF3(g) |
! |
B:BF3(soln) |
2 |
|
|
|
|
|
as the enthalpy change at 25 °C under 1 atm pressure in dichloromethane13. The two approaches are complementary. These scales, which cover a wide range of reactivity (ca 150 kJ mol 1), can be considered ‘hard basicity’ scales. Conversely, those using I2, I X (X D electron withdrawing group, EWG) or HgBr2 as reference acids are called ‘soft basicity’ scales.
3. Hydrogen bonding
Few attempts14 have yet been made to establish general scales for hydrogen-bond acidity and basicity. In his book11, Reichardt reviewed the hydrogen-bond subject when he analysed solvent effects. Kamlet and Taft defined a ˇ scale of solvent HBD basicities, as well as an ˛ scale of solvent HBA acidities15. Although a large number of properties have been interpreted in terms of hydrogen-bond acidity (˛) and/or hydrogen-bond basicityˇ 16, it is only recently that an effort has been made for building general HBD and HBA scales based on thermodynamic measures17 20.
A scale was developed of relative hydrogen-bond basicity for a wide variety of compounds by means of their retention in gas chromatography21. Results indicate that the

382 |
Silvia Bradamante |
use of hydrogen-bond basicity scales based on the free energy of formation of hydrogenbond complexes to the rationalization of solvation-related phenomena must be used with discretion.
B. Acidity and Basicity Scales in the Gas Phase
1. Scales relative to proton
The acidity of a substrate AH in the gas phase is measured using the standard enthalpy change H°acid for the heterolytic bond dissociation:
|
|
AH ! A C HC |
3 |
|||||||
As the process cannot be directly studied, H° |
can be calculated as follows22 |
with |
||||||||
good approximation ( 2 kJ mol 1): |
|
|
|
acid |
|
|
|
|
||
|
š |
|
|
|
|
|
|
|
|
|
AH |
! Až C Hž |
DA H |
bond dissociation energy |
|
||||||
Až C e ! A |
EAA |
electron affinity |
|
|||||||
Hž |
! HC C e |
IEHž |
|
ionization energy |
|
|||||
|
H° |
DA |
|
H |
|
EAA |
C |
IEHž |
(4) |
|
|
acid D |
|
|
|
|
|
|
The proton affinity PA of a substrate B, defined as fH° B , can be similarly
calculated22: |
|
BHC ! B C HC |
5 |
Inspection of equations 3 and 5 leads to the following: Hacid° |
of substrate AH is |
equal to PA of its anion A , and PA of substrate B is equal to |
Hacid° of its cation |
BHC . Nevertheless, in accordance with common scientific use, the acidity scale of AH is presented separately from the basicity scale of B. Analogously, gas-phase acidity
( G°acid) and gas-phase basicity (GB) are used for the Gibbs free-energy scales. The values have been obtained using various experimental methods. A critical review of three
different experimental approaches for measuring bond energies has recently been published and considered radical kinetics, gas-phase acidity cycles and photoionization mass spectroscopy23. None of these methods appears to be completely satisfactory, but all of the measurements made using one method lead to bond energies within the error bars of the others (with the exception of the ethylene case). The gas-phase basicity of most organic compounds is within the 700 1000 kJ mol 1 range, whereas the gas-phase acidities are within the 450 kJ mol 1 range. Tables 1 and 2 show some significant values of
TABLE 1. Gas-phase basicities: thermodynamic data in kJ mol 1 for BHC ! B C HC
|
PA |
GB |
T S° |
CH3NO2 |
750 |
718 |
32 |
NH3 |
853.5 |
818 |
35.5 |
(CH3)2NCHO |
884 |
852 |
32 |
CH3NH2 |
896 |
861 |
35 |
C5H5N |
924 |
892 |
32 |
(CH3)3N |
942 |
909 |
33 |
(CH3)2N(CH2)3N(CH3)2 |
1021 |
969 |
52 |
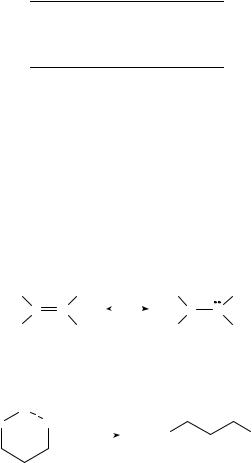
|
9. Acidity and basicity |
383 |
||
TABLE 2. Gas-phase acidities: thermodynamic data in kJ mol 1 |
||||
for AH ! A C HC |
|
|
|
|
|
H° |
G° |
T S° |
|
|
acid |
acid |
|
|
HNO2 |
358 |
1330 |
28 |
|
CH3NO2 |
1491 |
1463 |
28 |
|
HCONH2 |
1506 |
1476 |
30 |
|
(CH3)2NH |
1658 |
1628 |
30 |
|
CH3NH2 |
1687 |
1656 |
31 |
|
NH3 |
1689 |
1657 |
32 |
|
TABLE 3. Effect of hybridization on PA in kJ mol 1
Compound |
PAa |
H3C NH2 |
896 |
H2CDNH |
845 |
HC N |
717 |
aReference 24.
nitrogen-containing compounds. Acidity decreases with an increase in H°acid while the basicity increases with an increase in PA.
Deprotonation processes are exclusively governed by intrinsic structural effects, without any external stabilization. Therefore, the thermodynamic data are affected by the nature of the atom bound to the proton, its hybridization, the adjacent functionality and the transmitted substituent effects.
The physico-chemical aspects of proton transfer reactions involving amines have been interpreted in terms of various intrinsic effects arising from their molecular structures. Although acidities increase by increasing the s character of the reacting atom, no quantitative relation has been found between the s character and the variation in basicity in the case of oxygen and nitrogen bases. In particular the sp2-hybridized nitrogen bases are very close in PA to the corresponding sp3 bases (as shown in Table 3). This has been ascribed to the extra-stabilizing effect of the resonance structures (equation 6).
H |
+ |
H |
|
H |
+ |
|
H |
|
|
|
|
|
|
||
C |
N |
|
|
|
C |
N |
(6) |
|
|
|
|||||
|
|
|
|
|
|
|
|
H |
|
H |
|
H |
|
|
H |
(1)
The loss of symmetry which occurs after deprotonation leads to bigger T S° values and the gain in degrees of freedom in internal rotation leads to bigger entropy values, as shown in reaction 7.
+H
Me2N |
NMe2 |
|
|
||
|
|
|
Me2N |
NMe2 |
(7) |
|
|
|
|||
|
|
|
|
|
(2)
The gas-phase basicity and acidity of amines were in a chapter of the first updated volume of this series2, which provides extensive tables of values of aliphatic and aromatic

384 Silvia Bradamante
amines. However, some of these values have subsequently been revised and reported by Lias and coworkers24, and these are the values which will be used in this review.
The value of the PA of ammonia has been extensively discussed, and values ranging from 202 to 208 kcal mol 1 have been proposed. In particular, since the originally accepted value of 204 kcal mol 1, a different value of 208.3 kcal mol 1 has been suggested on the basis of high-pressure mass spectrometry (HPMS) experiments25. The same authors25b subsequently reversed their arguments, returning to the accepted value of PA NH3 D 204 kcal mol 1.
In most papers the experimental proton affinity difference between water and ammonia is taken as being equal to 37.5 kcal mol 1. Various calculated values of this gap have appeared. The PA values of ammonia and water were 205.6 and 168 or 168.6 kcal mol 1 respectively ( PA D 37 or 37.6 kcal mol 1) according to Dixon and Lias26; the work of Defrees and McLean27 led to similar calculations of PA NH3 D 204.0 and PA H2O D 165.1, with PA D 38.9 kcal mol 1, although Pople and coworkers28 predicted a PA D 39.4 kcal mol 1.
Notwithstanding the large body of PA data, the significant uncertainities of the experimental PA values of water and ammonia (up to 3 4 kcal mol 1) have meant that no absolute affinity scale is yet available.
Using Fourier transform ion cyclotron resonance spectrometry (FT-ICR), Taft29 rechecked the proton transfer equilibria of a large number of compounds with a basicity that is lower than that of H2O. These new data have been compared with those obtained by McMahon’s group using the ICR method30 and found to be very close despite the differences in instrumentation, temperature and experimental techniques. On the contrary, discrepancies have been found with the values determined using HPMS, and when comparing the experimental and calculated basicities of fluorinated ammonia derivatives. The basicity of difluoroammonia F2NH is approximately 43 kcal mol 1 weaker than that of ammonia. Ab initio calculations [Gaussian 92, HF/6-311GŁŁ basis set, fully optimized geometry, zero-point vibrational energy (ZPV) differences included] predict PAcalc NH3 D 208.3 kcal mol 125,26 and PAcalc F2NH D 159.4 kcal mol 1 with PA D 49 kcal mol 1, to be compared with the experimental value PA NH3 D 43 kcal mol 1. The same discrepancy has been found for FNH2.
Ab initio molecular orbital calculations at the G2 level of theory is found31 consistently to reproduce experimental proton affinities to an accuracy of 10 kJ mol 1 for a range of bases with PA spanning ca 500 kJ mol 1.
2. Metal cation binding
By analogy with the basicity towards protons (equation 5) the basicity towards monocharged metal ions (Table 4) is defined by means of the thermodynamics of the reaction:
BMC ! B C MC |
8 |
In practice, thermodynamic data are obtained from the metal-ion transfer reactions:
B1MC |
C |
B2 |
! |
B1 |
C |
B2MC |
9 |
|
|
|
|
|
|
Kebarle and coworkers32 proposed a basicity scale towards KC , in which the potassium affinities are always smaller than the corresponding PA values.
More precise relative Gibbs free-energy scales were established using equation 9. Other metal cations have also been considered, such as Li, Al and Mn: in general, the order of basicity found in the KC scale was not altered33.
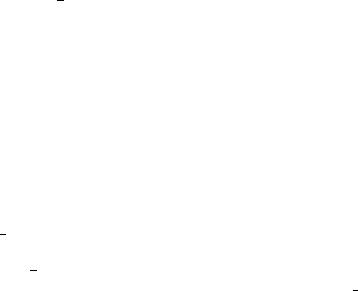
|
9. Acidity and basicity |
385 |
||
TABLE 4. Gas-phase basicities towards KC ; thermodynamic |
||||
data in kJ mol 1 for BKC ! B C KC |
|
|
||
|
KC A |
KC GB |
KC T S° |
|
NH3 |
75 |
50 |
25 |
|
(CH3)3N |
84 |
54 |
30 |
|
C5H5N |
88 |
63 |
25 |
|
(CH3)2NCHO |
130 |
96 |
34 |
|
3. Organometallic and organic cation binding
Knowledge of the gas-phase interactions between cations involving elements of group 14 and electron donor molecules has been used to create various basicity scales. In addition, the absolute methyl cation affinities (MCA) scale has been built by calculating the
enthalpies of reactions 10 and 11 by means of PA and H°34 .
ACH3 |
! |
A C CH3C |
(10) |
BCH3 |
! |
B C CH3C |
(11) |
4. Anion binding and electron affinities
ICR and HPMS have been used to determine the thermochemical data of reactions:
A-HBD |
! |
A C HBD |
(12) |
A-EPA |
! |
A C EPA |
(13) |
where EPA D electron pair acceptor.
Many gas-phase acidity scales have been proposed, according to the nature of the interacting systems35 39.
The electron affinity EA is related to the energy of the lowest unoccupied molecular orbital and is defined as the enthalpy at 0 K for22:
M ! e C M |
14 |
Since reaction 14 can be considered similar to reaction 13, approximations between EA and Gibbs free-energy are possible; this subject has been reviewed by Kebarle and Chowdhury40.
5. Cationic hydrogen bonds
In general, the interaction of a protonated base BHC with a neutral HBA is described by the following relationship:
BHC . . .HBA ! BHC C HBA |
15 |
By keeping either BHC or HBA constant, hydrogen-bond basicity or acidity scales can be built41 43.
C. Gas Phase Solution Relationships
Over the last decade, many approaches have been proposed for relating acidity basicity dependent properties in gas phase and solution. To evaluate the solvation effect, the
386 |
Silvia Bradamante |
|
|
|
following thermodynamic cycle is adopted: |
|
|
|
|
|
X°(g) |
|
|
|
A(g) |
! |
B(g) |
|
|
|
° |
|
° |
(16) |
solvXA |
solvXB |
|||
|
|
|
|
|
|
|
|
|
|
A(soln) |
! |
B(soln) |
|
|
|
X° |
|
|
|
|
soln |
|
|
|
where A and B are neutral or ionic reactants and products, X° is the change in thermodynamic function H°, G° or S°, and solv (D solvation) represents the transfer from the gas phase to the solution.
Xsoln° |
D X° g C [ solvXB° solvXA° ] |
17 |
where X°soln is defined as the sum of an intrinsic gas-phase component and a differential solvation term that quantitatively accounts for the variation in basicity or acidity order between the two phases.
The effects of hydration on the basicity of many classes of organic compounds (mainly oxygen and nitrogen bases) have been studied experimentally and/or by using useful approximations for evaluating the change in the thermodynamic functions. In this context, protonated and deprotonated forms of ˛-amino acids have been considered44. However, this approach has generally been found to be accurate only in the case of simple monofunctional molecules.
Within the general framework of relating solution and gas-phase behaviour, many theoretical approaches have been developed to evaluate ion hydration:
(1)the continuum methods45;
(2)the Monte Carlo and molecular dynamic simulations46;
(3)the supermolecule approach47.
Alternatively, a great number of correlations have been made in order to establish empirical relationships between gas-phase and solution acidity and basicity.
In general, there is always an attenuation in effects from gas phase to solution (slope < 1), but this has been found to be particularly large for substituent effects of the basicity of alkylamines48 and pyridines49 in water.
Evaluation of the relative differential solvation term was made possible when a linear relationship with a slope close to 1 was found. The reference plot was obtained correlating G°(aq) versus the GB of large aromatic hydrocarbons (slope ca 1): in fact, these hydrocarbons are not specifically solvated, give highly charge-delocalized cations and have negligible specific interactions. Under these circumstances, the polarizability and resonance effects are similar in the gas phase and in water with the differential solvation of B and BHC remaining almost constant. Thus, any deviation from the reference line is a measure of the relative differential solvation50.
Much work has been conducted to find a similar relationship for acidity. The limitation of measuring weak acidities in water has been overcome by the use of acidities in DMSO6, with G° (DMSO) versus G°acid of large unsaturated hydrocarbons being the reference relationship. In this correlation NH3 is a deviant point. This result was attributed to specific differential solvation: on deprotonation, ammonia generates the small and highly charge-
localized NH2 anion. Comparison of the G°(DMSO) and the G°acid of many classes of compounds showed that electron-withdrawing substituted anilines were less acidic in
DMSO, which can be explained by the stronger HBD (greater solvation of the neutral)
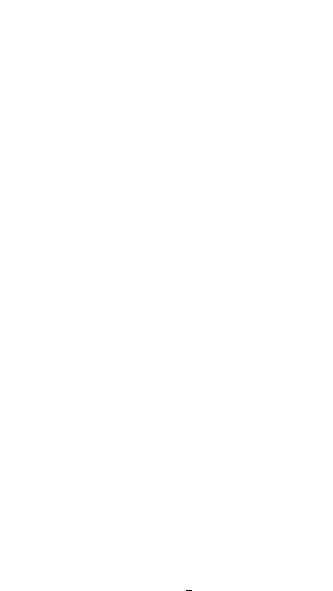
9. Acidity and basicity |
387 |
FIGURE 1. Plot of gas-phase electron affinities from electron-transfer equilibria measurements vs electron affinities deduced from polarographic half-wave potentials and charge-transfer spectra. The observed slope is not equal to 1 but is ca 0.72. This reveals the effect of decreasing solvation exothermicity with increasing charge delocalization of negative ions since high EA molecules lead to ions with more extensive charge delocalization (1 eV D 96.48456 kJ mol 1). Reprinted with permission from Reference 40. Copyright (1987) American Chemical Society
and weaker ion solvation due to considerable charge delocalization in the anions. This effect was also found by plotting the basicities in water against the gas-phase basicities of some enamino ketones51. The greater acidities measured in DMSO and water than in the gas-phase of p-NO2- and p-NO-phenol were attributed to charge localization on the substituent52.
As with proton transfer reactions, the thermodynamic data determined in the two phases can also be compared in the case of electron transfer reactions, as shown in Figure 140.
By correlating the gas-phase and solution data derived from proton transfer reaction, the deviations were accounted for by solvation effects on the reactive centre and/or the substituent.
In the case of solvents with a low solvating power, in which protonated or deprotonated species are not free from counterion effects, spectroscopic and thermodynamic parameters associated with hydrogen bonding or Lewis acid base interactions can be usefully related to PA or GB. Kamlet and coworkers53 reported linear relationships for five series of bases with sp2- and sp3-hybridized oxygen and sp-, sp2- and sp3-hybridized nitrogen. At the same time, Zeegers-Huyskens presented the relationships54 reported in Figure 2.
More details on the possibility of correlating the acidity and basicity values derived in solution and in the gas-phase can be found in the chapter written by Gal and Maria55, where the authors concentrated on the possibility of relating acid/base interactions in various media.

388 |
Silvia Bradamante |
−∆G°HB (kcal mol−1)
3
2
1
0
120 |
130 |
140 |
150 |
160 |
170 |
GA−GB (kcal mol−1)
FIGURE 2. Gibbs free-energy of hydrogen bonding, in tetrachloromethane, between OH HBD and N HBA ( nitriles, ° pyridines, ž anilines). Reproduced with permission from Reference 54
IV. NITROGEN BASES
A. Amine Derivatives
The constants of ion pair formation of 33 amines with 2,4-dinitrophenol in benzeneKB have been compared with the pKa in water56. The effects of structural variations on basicity are larger in water than in benzene for primary and secondary cyclic amines, but similar for tertiary amines. The Taft and Hancock equation [where Ł has the usual meaning and Ecs (Ri) is the steric effect of a component substituent]
log KB D Ł Ł C aiEsc Ri C c |
18 |
i |
|
allows the unified treatment of the determined KB values and indicates that the overall effects of the three substituents at nitrogen can be well accounted for by electronic and steric effects.
In the absence of any chelation effect, the relative base strength of substituted uncon-
jugated amines [Me2NR with R D H, CH3, C2H5, i-C3H7, i-C4H9, sec-C4H9, t-C4H9, c-C6H11, t-C5H11, neo-C5H11, C6H5CH2, H2CDCHCH2, HC CCH2, CF3CH2, NCCH2, (CH3)2NCH2] is governed by the substituent and the solvent. Investigation of their relative
basicity in water and in the gas phase indicates that the importance of polarizability effects over field/inductive effects is greater in the gas phase than in solution57. The hydrogen substituent does not correlate with the other substituents: in fact, in addition to having different hyperconjugation forms, it also offers an additional site for hydrogen-bond formation (Figure 3).
The gas-phase basicity order of alkylamines can be reproduced in terms of softness of the alkyl groups (using the Fukui function fk and local softness parameter sk ), this being far more important than group electronegativity58.