

Supplement F2: The Chemistry of Amino, Nitroso, Nitro and Related Groups.
Edited by Saul Patai Copyright 1996 John Wiley & Sons, Ltd.
ISBN: 0-471-95171-4
CHAPTER 23
Reactions of nitrosoarenes with SH groups
P. EYER and D. GALLEMANN
Walther-Straub-Institut fur¨ Pharmakologie und Toxikologie, Ludwig-Maximilians- Universitat¨ Munchen,¨ Nuˇbaumstr. 26, D-80336 Munchen,¨ Germany
Fax: (089)-51452-224
I. INTRODUCTION AND HISTORICAL REMARKS . . . . . . . . . . . . . . . . 1000 II. REACTION PRODUCTS AND PATHWAYS . . . . . . . . . . . . . . . . . . . . . 1001 A. Overview . . . . . . . . . . . . . . . . . . . . . . . . . . . . . . . . . . . . . . . . . . 1001 B. Formation of the Semimercaptal Intermediate . . . . . . . . . . . . . . . . . . 1001 1. Structural elucidation . . . . . . . . . . . . . . . . . . . . . . . . . . . . . . . . 1001 2. Kinetic aspects of semimercaptal formation . . . . . . . . . . . . . . . . . . 1003
C. Formation of the N-Hydroxyarylamine and its Secondary Products . . . . 1007 1. N-Hydroxyarylamine . . . . . . . . . . . . . . . . . . . . . . . . . . . . . . . . 1007 2. The bifurcation at the semimercaptal stage . . . . . . . . . . . . . . . . . . 1007 3. Secondary products . . . . . . . . . . . . . . . . . . . . . . . . . . . . . . . . . 1009
D. The Sulfenamide Cation Descendants . . . . . . . . . . . . . . . . . . . . . . . . 1009 1. The sulfinamide . . . . . . . . . . . . . . . . . . . . . . . . . . . . . . . . . . . . 1009 a. Identification . . . . . . . . . . . . . . . . . . . . . . . . . . . . . . . . . . . . 1009 b. Formation mechanism and kinetics . . . . . . . . . . . . . . . . . . . . . 1010
2. Sulfenamide and arylamine . . . . . . . . . . . . . . . . . . . . . . . . . . . . 1011 a. Formation and identification . . . . . . . . . . . . . . . . . . . . . . . . . . 1011 b. Remarks on the formation mechanism . . . . . . . . . . . . . . . . . . . 1012
3. Thio ether . . . . . . . . . . . . . . . . . . . . . . . . . . . . . . . . . . . . . . . 1014 4. N-Sulfenylquinonimines and resultant products . . . . . . . . . . . . . . . 1015 a. Monocyclic products . . . . . . . . . . . . . . . . . . . . . . . . . . . . . . 1015 b. Bicyclic products . . . . . . . . . . . . . . . . . . . . . . . . . . . . . . . . . 1016
c. N-Sulfenylquinonimine formation from nitrosophenols
and nitrosoanilines . . . . . . . . . . . . . . . . . . . . . . . . . . . . . . . . 1018 E. Formation of N-Hydroxysulfonamide . . . . . . . . . . . . . . . . . . . . . . . . 1019 F. Reaction Pathways Involving Radical Intermediates . . . . . . . . . . . . . . 1019
III. BIOLOGICAL SIGNIFICANCE . . . . . . . . . . . . . . . . . . . . . . . . . . . . . 1020 A. Introduction . . . . . . . . . . . . . . . . . . . . . . . . . . . . . . . . . . . . . . . . 1020 B. Monocyclic Nitrosoaromatics . . . . . . . . . . . . . . . . . . . . . . . . . . . . . 1022
999
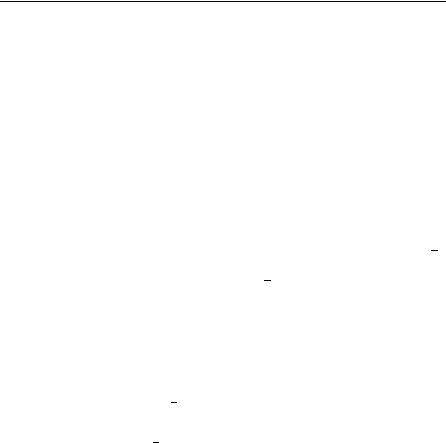
1000 |
P. Eyer and D. Gallemann |
1. Nitrosobenzene generated from nitrobenzene and aniline . . . . . . . . . 1022 2. Nitroso-procainamide from procainamide . . . . . . . . . . . . . . . . . . . 1023 3. 3-Nitrosobenzamide . . . . . . . . . . . . . . . . . . . . . . . . . . . . . . . . . 1023 4. Nitroso derivatives of chloramphenicol . . . . . . . . . . . . . . . . . . . . . 1024 5. Nitroso derivatives of chloroanilines . . . . . . . . . . . . . . . . . . . . . . 1026 6. Nitroso derivatives of sulfonamide drugs . . . . . . . . . . . . . . . . . . . 1026 7. Nitroso derivatives of dinitrobenzenes . . . . . . . . . . . . . . . . . . . . . 1028 8. 4-Nitrosophenetol from 4-phenetidine . . . . . . . . . . . . . . . . . . . . . 1029 9. 4-Nitroso-N,N-dimethylaniline . . . . . . . . . . . . . . . . . . . . . . . . . . 1029
C. Polycyclic Nitrosoaromatics . . . . . . . . . . . . . . . . . . . . . . . . . . . . . . 1030 1. 4-Nitrosobiphenyl from 4-aminobiphenyl . . . . . . . . . . . . . . . . . . . 1030 2. 2-Nitrosofluorene from 2-acetamidofluorene . . . . . . . . . . . . . . . . . 1031
D. Heterocyclic Nitrosoaromatics . . . . . . . . . . . . . . . . . . . . . . . . . . . . 1031 1. Nitrosoimidazoles from nitroimidazoles . . . . . . . . . . . . . . . . . . . . 1031 2. Heterocyclic N-oxygenated compounds derived from food 1033
mutagens . . . . . . . . . . . . . . . . . . . . . . . . . . . . . . . . . . . . . . . .
IV. REFERENCES . . . . . . . . . . . . . . . . . . . . . . . . . . . . . . . . . . . . . . . . . 1035
I. INTRODUCTION AND HISTORICAL REMARKS
The reaction of the aromatic nitroso group with thiols was unknown in organic chemistry until the sixties of this century. Thus, in 1969 Furuya and coworkers attempted to investigate the mechanism of thio etherification of 4-nitrosophenol in more detail when they reacted it with ethylmercaptan1. The products they observed, however, most probably resulted from the interaction of the nitroso group with the thiol. This gap in organochemical knowledge is quite amazing since reactions of nitrosoarenes with thiols are exothermic and kinetically uninhibited at ambient temperature. Furthermore, these reactions obviously parallel the semiacetal formation of carbonyls reacting with alcohols. Moreover, similar reactions of nitrosoaromatics with active hydrogen compounds are well known2,3. Thus, a semiacetal-like product was identified in 1901 by Bamberger and Rising4 while reacting nitrosobenzene with 4-toluenesulfinic acid. However, until the late seventies there are only a few papers superficially dealing with the interaction of nitrosoarenes with thiols.
To our knowledge, this reaction was first mentioned by Smentowski in 19635. He investigated the reaction of nitrosobenzene with 2-naphthylthiol and reported the thiol disulfide and the corresponding azo(xy)aromatics to be the main products. A variety of products have been observed by several groups: thiol disulfide5,6, azo(xy)aromatics5 7, arylamines6,8, an adduct of nitrosoarene with thiol which could be hydrolyzed to the corresponding arylamine by acid or alkali treatment9 11 and a thio ether8,12. Despite this mysterious medley of different products emerging from the reaction of nitrosoaromatics with thiols, no detailed investigation was undertaken to illuminate the obviously complex reactions until toxicologists took an interest in that topic. This interest arose when it became clear that nitrosoarenes and the N-hydroxyarylamines are biological reactive intermediates that are involved in toxic, allergic, mutagenic and carcinogenic effects. Hence, interactions with cellular thiols, predominantly with glutathione (GSH) and protein SH groups, were considered to be important for the detoxication of nitrosoarenes. In 1977, Neumann and coworkers11 were the first to present a hypothetical scheme illustrating how a labile nitrosoarene/thiol adduct9 11 may liberate the arylamine.
In 1958, Kiese and coworkers for the first time detected nitrosobenzene in the blood of dogs after aniline administration, and it was his group who discovered N-oxygenation and the site of action in vivo13 16. Finally, Kiese initiated17 and extended the research in the metabolic fate of nitrosobenzene in erythrocytes10 and encouraged one of the authors
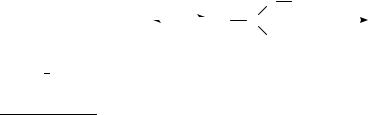
23. Reactions of nitrosoarenes with SH groups |
1001 |
to participate in this field18,19. Hence, we greatfully took the opportunity to maintain this tradition by collecting the pertinent data and presenting a comprehensive review on chemical reactions of the aromatic nitroso group with thiols. Detailed investigations in well defined chemical systems revealed these reactions to be very complex, and a lot of items remain in the dark until today. Since scientists of chemical toxicology are expected to be among the most interested readers of this chapter, the biological significance of these reactions is presented in more detail, too.
II. REACTION PRODUCTS AND PATHWAYS
A. Overview
Scheme 1 summarizes the main reaction pathways proposed for the interaction of nitrosoarenes with thiols in aqueous, neutral solvents as deduced from product patterns and kinetic data. Accordingly, the first reaction step is the formation of an addition product (1), termed ‘semimercaptal’. This labile intermediate is cleaved by a second thiol molecule yielding the corresponding N-hydroxyarylamine (2). Alternatively, the semimercaptal N O bond may be broken with formation of an electrophilic sulfenamide cation
(3). Both reaction pathways compete for each other, depending on aryl substituent(s), pH of the reaction medium, and concentration and pKa of the thiol. The ramification of the reaction pathway at the sulfenamide cation stage is even more complex since the positive charge is delocalized through the aromatic system, depending on the aryl substituent(s). Generally, three electrophilic centers (the sulfur atom and the o- and p-position of the aromatic ring) can react with various nucleophiles (excess thiol, solvent H2O and the reaction product arylamine 8), giving rise to a variety of intermediates and end products such as sulfinamides (4), sulfenamides (5 and 6), thio ethers (7), arylamines (8) and N- sulfenylquinonimines (9 and 10). Again, the preference of a distinct pathway depends on the aryl substituent(s) and the thiol concentration. Beside the pathways summarized in Scheme 1, a few additional reaction possibilities are reviewed in Sections II.E and II.F.
B. Formation of the Semimercaptal Intermediate
1. Structural elucidation
According to the different electronegativity of nitrogen and oxygen, the nitroso
group is polarized in analogy to the homologous carbonyl group†. Similar to alcohols reacting with carbonyls, the addition of thiols to nitrosoarenes yields a semiacetal-like N-hydroxysulfenamide which was first postulated by Youssefyeh21.
|
|
|
|
|
k1 (fast) |
S |
R |
(slow) |
|
||
|
|
|
|
O + RSH |
|
|
k2 |
products (1) |
|||
Ar |
|
N |
|
|
Ar |
N |
|
||||
|
|
|
|
|
|
||||||
|
|
|
|
|
k −1 |
OH |
|
|
|
|
|
|
|
|
|
|
|
|
|
|
|
|
|
The family of |
the N-hydroxysulfenamides |
has been |
commonly termed ‘semimer- |
captal’22 31, despite the potential confusion with carbonyl/thiol adducts (see, for example, Reference 32). With few exceptions, semimercaptals are very unstable and give various
† Molecular orbital calculations on the electrostatic potential of the aromatic nitroso group revealed an uneven charge distribution at the nitroso-nitrogen, enabling both electrophilic and nucleophilic attack20. Accordingly, the major negative region is located at the outside of the C Ar NDO angle within the molecular plane, reflecting the nitrogen lone electron pair. A significant build-up of a positive potential was calculated to be inside the C Ar NDO angle below and above the molecular plane.

RA / D
RA
N |
O + RSH |
|
|
|
|
RA / D |
|
|
OH |
−OH− |
|
RA / D |
N S R |
|
|
RA / D |
|
||
|
(1) |
|
|
|
+ RSH |
|
+ |
|
− RSSR |
RD |
|
|
|
|
|
OH |
|
|
|
N |
|
|
|
H |
|
|
|
(2) |
|
|
+ |
|
|
RD |
|
|
|
|
(3) |
|
|
+ H2 O |
|
|
|
− RD H / H+ |
|
|
O |
N S R |
RD |
|
|
(9) |
|
|
|
RSH |
|
+ |
|
+H2 O |
|
N S |
R |
−H+ |
RA / D |
+
N S R
|
+ RS− |
|
N S R |
|
RD |
|
N S R
+RS−, RSH
−RSSR
RD
− RDH / H+
(6)
N
(10)
O
N S R
H
(4)
|
|
S |
R |
|
|
|
|
|
N S |
R |
|
|
|
|
H |
|
|
|
(5) |
|
− RSSR |
+ RSH |
|
|
|
|
|
|
|
|
|
|
|
S |
R |
|
|
RD |
|
|
NH2 |
|
|
|
(7) |
|
|
N |
S |
R |
+ RSH |
RD |
NH2 |
− RSSR |
|||||
H |
|
|
|
|
|
|
|
|
|
|
(8) |
N |
S |
R |
|
|
|
RSH
SCHEME 1. Main reaction pathways during interaction of nitrosoarenes with thiols (RA denotes electron acceptor substituent and RD electron donor substituent)
1002
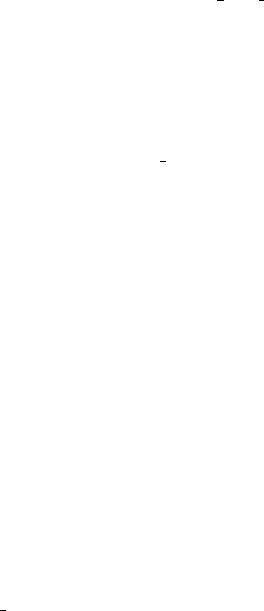
23. Reactions of nitrosoarenes with SH groups |
1003 |
products depending on the nature of their aryl substituent(s). Therefore, this family has only been hypothesized for about ten years11,18,21 23,25,33 37 before it could be isolated in substance. In our laboratory, Klehr reacted nitrosobenzene with 1-thioglycerol in methanol at 40 °C and confirmed the proposed structure by 13C-NMR, FAB-MS and
UV spectroscopy24,38. In the meantime some other semimercaptals have been proved structurally29,38,39.
Semimercaptals (1) exhibit a variety of spectroscopic characteristics. The most typical property is a strong FAB-MS signal at m/z D [MCH 18]C (positive ion mode), thereby enabling a clear distinction from the isomeric sulfinamide (4)24,29,38,39. Obviously, the N-hydroxy group is particularly prone to proton impact and subsequent loss of water. Further discrimination from the sulfinamide is given by the absence of an IR absorption atD 1060 cm 1 which is typical for the SDO double bond29. The semimercaptal exhibits maximum UV absorption in the region of 255 270 nm18,24,30,38,40. The 13C-NMR chemical shift of the ipso carbon atom of N-hydroxy-N-(1-thioglycerol-S-yl)-aniline was found to be similar to that of N-hydroxyaniline (152 ppm). The signal of the aliphatic carbon atom vicinal to the sulfur atom (37 ppm) appeared between the corresponding signals of the parent thiol and the sulfenamide, indicating a relatively weak electron withdrawal at the sulfur atom24,38 (Table 1). Characteristic 1H-NMR signals29 of semimercaptals, containing N S conjugated cysteinyl residues, are summarized in Table 1. On separation by reversed-phase HPLC, semimercaptals are eluted subsequent to the respective sulfinand sulfenamides24,38.
2. Kinetic aspects of semimercaptal formation
The formation of the semimercaptal has been shown to be reversible18,22,30,36,38,40 . First indications came from a simple observation: nitrosobenzene18,38 and nitrosochloramphenicol22, respectively, having reacted with GSH to complete disappearance of their characteristic UV absorption, could be recovered from the reaction mixture by extraction with ether. During reaction of various nitrosoarenes with thiols, no isosbestic points were detected between the UV spectra of the nitrosoarenes and their end products, indicating formation of a labile intermediate18,22,38,40. Correspondingly, absorbance of most nitrosoarenes decreased biphasically when reacted with excess thiol18,22,30,36,40. A
rapid initial fall in optical density |
|
reflecting the establishment of the semimercaptal |
||
|
||||
equilibrium |
|
was followed by a |
slower decrease due to consecutive reactions of |
|
|
the semimercaptal with concomitant readjustment of the preceding equilibrium (see equation 1). Similarly, the rapid build-up and the slower decrease of the semimercaptal can be observed in the region around 260 nm18,30 (the maximum absorbance of the semimercaptals).
Forward and reverse reaction rates have been shown to increase with rise in pH18,30,38, but no indications for general acid or base catalysis were found30. Therefore, the thiolate anion is assumed to be the nucleophile ultimately reacting during the forward reaction (Scheme 2). This conclusion is corroborated by the rate constants of semimercaptal formation with various thiols (Table 2). The marked differences in reactivities at pH 7.4 are obviously largely due to the different degree of dissociation, except for t-butylthiol, hemoglobin cysteine (ˇ 93) SH groups (Hb SH) and thiophenol. (With t-butylthiolate
and Hb S 46 48 , steric hindrance may cause the low reaction rates. The low reactivity of phenylthiolate is attributed to resonance stabilization of the negative charge, as indicated by the Hammett reaction constant for dissociation of substituted thiophenols ³ 249).
In |
the |
back |
reaction, the |
N |
|
S |
cleavage of the semimercaptal anion (pK |
a |
estimated |
||
|
30 |
) |
is suggested |
|
be |
|
|
||||
as |
³ 10 |
|
to |
the rate-determining step (Scheme 2). Forward and |
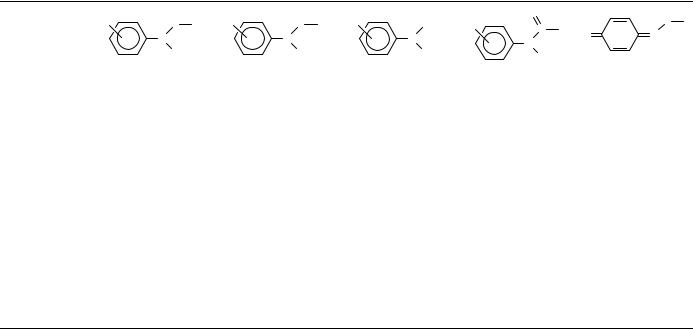
TABLE 1. Comparison of characteristic properties of N S conjugates emerging during the reaction of nitrosoarenes with alkanethiolsa
|
|
R1 |
|
|
|
|
|
|
|
R1 |
|
|
|
|
|
|
R1 |
|
|
|
|
|
|
|
|
|
|
|
|
O |
|
|
|
S R |
|||||||
|
|
|
|
S R |
|
|
|
|
|
|
|
|
S R |
|
|
|
SO2 R |
|
R1 |
|
|
|
|
|
|
|
|
S * R |
O |
|
|
||||||||||
|
|
|
|
|
|
N |
|
|
|
|
|
|
|
|
|
|
|
N |
|
|
|
|
N |
|
|
|
|
|
|
|
|
|
N |
|
|
N |
|||||
|
|
|
|
|
|
|
|
|
|
|
|
|
|
|
|
|
|
|
|
|
|
|
|
|
|
|
|
|
|
|
|
|
|
||||||||
|
|
|
|
|
|
OH |
|
|
|
|
|
|
|
|
|
|
|
H |
|
|
|
|
OH |
|
|
|
|
|
|
|
|
|
|
|
|
|
|||||
|
|
|
|
|
|
|
|
|
|
|
|
|
|
|
|
|
|
|
|
|
|
|
|
|
|
|
|
|
|
|
|
H |
|
|
|
|
|||||
|
|
|
|
|
|
|
|
|
|
|
|
|
|
|
|
|
|
|
|
|
|
|
|
|
|
|
|
|
|
|
|
|
|
|
|
|
|
|
|||
|
|
|
|
|
|
|
|
|
|
|
|
|
|
|
|
|
|
|
|
|
|
|
|
|
|
|
|
|
|
|
|
|
|
|
|
|
|
|
|
|
|
Reversed-phase |
|
|
|
|
Rt |
> |
|
|
|
|
|
|
Rt |
? |
|
|
Rt |
> |
|
|
|
|
|
Rtc |
> |
|
Rt |
||||||||||||||
|
|
|
|
|
|
|
|
|
|
³ |
|
|
|
|
|
|
|
|
|||||||||||||||||||||||
HPLCb |
|
|
|
|
|
|
ArNH2 C RSSR C RSO2Hd |
ArNO C RSO2H |
|
|
|
|
|
|
|
|
|
|
|
|
|
|
|
|
|
|
|||||||||||||||
Neutral hydrolysis |
Ar-NH-SO-R |
|
|
|
|
|
|
|
|
|
|
|
|
|
|
|
|
|
|
|
|||||||||||||||||||||
|
|
|
|
|
|
|
|
|
|
|
|
|
|
|
|
|
|
|
|||||||||||||||||||||||
Acidic hydrolysis |
ArNH2 C RSO2H |
|
ArNH2 C RSSR C RSO2Hd |
|
|
|
|
|
ArNH2 C RSO2H |
|
|
|
|
||||||||||||||||||||||||||||
|
|
|
|
|
|
|
|
|
|
||||||||||||||||||||||||||||||||
Alkaline hydrolysis |
ArNH224 |
|
|
|
|
|
|
|
|
|
|
|
24 |
ArNO C RSO2H |
ArNH2 C RSO2H |
|
|
|
|
||||||||||||||||||||||
|
|
|
|
|
|
|
|
|
|
|
|
|
|
|
|||||||||||||||||||||||||||
Reaction with RSH |
Ar-NHOH C RSSR |
|
ArNH2 C RSSR |
|
|
|
|
|
|
|
|
|
|
|
|
|
|
|
|
|
|
|
4-aminophenol, |
||||||||||||||||||
|
|
|
|
|
|
|
|
|
|
|
|
|
|
|
|
|
|
|
|
||||||||||||||||||||||
|
|
|
|
|
|
|
|
|
|
|
|
|
|
|
|
|
|
|
|
|
|
|
|
|
|
|
|
|
|
|
|
|
|
|
|
|
|
thio ether |
|||
|
|
|
|
|
|
|
|
|
|
|
|
|
|
|
|
|
|
|
|
|
|
|
|
|
|
|
|
|
|
|
|
|
|
|
|
|
|
|
|
|
|
UV/Vise |
|
|
|
|
|
|
|
|
|
|
|
255f |
|
|
³ 250 |
|
|
|
|
|
|
245f,g |
|
|
|
|
|||||||||||||||
max 1 |
(nm) |
255 |
|
270 |
|
244 |
|
|
|
|
|
230 |
|
412 |
|
|
|
||||||||||||||||||||||||
|
|
|
|
|
|
|
|
|
|
|
|||||||||||||||||||||||||||||||
max 2 |
(nm) |
|
|
|
|
|
|
280 |
|
304f |
|
|
|
|
|
|
|
271 |
|
288f |
|
|
|
|
|||||||||||||||||
|
|
|
|
|
|
|
|
|
|
|
|
|
|
|
|
|
|
|
|||||||||||||||||||||||
ε 1 /ε 2 |
|
D |
|
|
|
|
5 |
|
|
7 |
|
|
|
|
|
|
|
|
|
³ 1165/1360 |
8 |
|
14 |
³ 1060 |
|
|
|
|
|
|
|
|
|
||||||||
|
|
|
|
|
|
|
|
|
|
|
|
|
|
|
|
|
|
|
|
|
|
|
|
|
|
||||||||||||||||
IR: (cm 1) |
|
O |
not existing |
|
|
|
D |
O |
not existing |
|
SO2 |
|
D |
O |
|
|
|
|
|
|
|
|
|
||||||||||||||||||
|
|
S |
|
|
|
S |
|
|
|
|
|
|
|
|
|
|
|
S |
|
|
|
|
|
|
|
|
|
[M C H]C , |
|||||||||||||
FAB-MS |
[M C H]C , |
|
[M C H]C , |
|
|
[M C H]C , |
[M C H]C , |
||||||||||||||||||||||||||||||||||
|
|
[M C H H2O]C |
|
[M C H SR]C |
[M C H O]C |
[M C H ArNH]C |
[M C H SR]C |
||||||||||||||||||||||||||||||||||
13C-NMRh |
CAryl N S: 152 |
|
CAryl N S: 149 |
|
|
|
|
|
CAryl N S: 139 |
|
|
142 |
|
|
|
|
|||||||||||||||||||||||||
|
|
|
|
|
|
|
|
|
|
|
|
||||||||||||||||||||||||||||||
υ (ppm) |
N S CAlkyl: 37 |
|
N S CAlkyl: 42 |
|
|
|
|
|
N S CAlkyl: 56 |
|
58i |
|
|
|
|
||||||||||||||||||||||||||
|
|
|
|
|
|
|
|
|
|
|
|||||||||||||||||||||||||||||||
1H-NMRj |
cys ˇ1: 3.0 |
|
cys |
ˇ1: 2.7 |
|
3.0 |
cys |
ˇ1/2: 4.8 |
cys |
ˇ1: 3.4 |
|
3.6i |
cys ˇ1: 3.64 |
||||||||||||||||||||||||||||
|
|
|
|||||||||||||||||||||||||||||||||||||||
υ (ppm) |
cys |
ˇ2: 3.4 |
|
cys |
ˇ2: 3.1 |
|
3.2 |
|
|
|
|
|
cys |
ˇ2: 3.6 |
|
3.7i |
cys |
ˇ2: 3.87 |
|||||||||||||||||||||||
|
|
|
|
|
|
|
|
a For references compare the respective text parts.
bRetention times (Rt) compared according to24,26,38,43,45.
c With chiral thiols, double peaks may be obtained at high chromatographic resolution. d Originally, RSOH will result which disproportionates according to equation 7.
e In MeOH/H2O.
f Corresponding sulfenand sulfinamides exhibit about 15 nm difference in their absorption maxima; -donor substituted sulfenand sulfinamides reveal a slight bathochromic shift38. gFor the probable glutathione sulfinamide of the polycyclic 3-nitroso-1-methyl-5H-pyrido[4,3-b]indole, the UV maximum was reported at 262 nm41.
h Data of derivatives from nitrosobenzene reacted with 1-thioglycerol, 2-thioethanol and cysteamine (in CD3OD). The corresponding signal of the original thiols are located at ³27 ppm24,42.
iWith chiral thiols, signals are doubly splitted.
j Data of derivatives containing N S conjugated cysteinyl residues (in CD3OD, D2O or mixtures of both). The corresponding signals of GSH, for example, are located at cys ˇ1: 2.88 š 0.04 ppm and cys ˇ2: 2.93 š 0.03 ppm in D2O35,44.
1004

|
|
23. Reactions of nitrosoarenes with SH groups |
|
1005 |
|||||||
RSH |
Ka (RSH) |
RS− |
|
|
|
|
|
|
|
|
|
|
O |
k1 |
S |
R |
|
|
|
S |
R |
||
|
|
|
|
||||||||
|
|
|
|
|
|
||||||
|
|
Ar |
N |
Ar N |
|
|
|
|
Ar N |
|
|
|
|
|
− |
|
|
|
|
||||
|
|
|
|
|
|
|
|||||
|
|
k −1 |
K |
|
(−NOH) |
|
|||||
|
|
|
|
|
a |
OH |
|||||
|
|
|
|
|
O |
|
|
||||
SCHEME 2. Mechanism |
of semimercaptal |
formation |
(from |
Kazanis and |
McClelland30, |
with |
|||||
modification) |
|
|
|
|
|
|
|
|
|
|
TABLE 2. Second-order rate constants of the semimercaptal formation for various thiols reacting with nitrosobenzene (conditions unless otherwise specified: pH 7.4, 37 ° C, nitrogen)
|
k1,obsa |
|
k1,thiolateb |
|
||||
Thiol |
(M 1s 1) |
pKa (SH) |
(M 1s 1) |
Reference |
||||
|
|
3 |
8.649 |
|
|
|
5 |
|
Cysteine |
12 ð 103 |
2.0 |
ð 105 |
36 |
||||
Cysteamine |
10 |
8.3 |
. |
ð 105 |
25 |
|||
Glutathione |
12 ð |
3 |
1 0 |
|||||
5 ð 10 |
3 |
8.849 |
1.2 |
ð 105 |
25 |
|||
1,2-Ethanedithiol |
4.5 ð 103 |
9.149 |
2.2 |
ð 105 |
38 |
|||
1-Thioglycerol |
1.7 ð 103 |
9.549 |
2.1 |
ð 105 |
38 |
|||
Benzylthiol |
1.5 ð 103 |
9.4 |
1.5 |
ð 105 |
36 |
|||
Thioethyleneglycol |
1.3 ð 10 |
9.6 |
2.1 |
ð 10 |
25 |
|||
Thiophenol |
32 |
|
6.5 |
40 |
|
|
|
25 |
Hb SH |
5.5c |
|
>1151 |
> . |
2 ð |
104 |
40 |
|
|
4 |
|
49 |
2 |
|
3 |
25 |
|
t-Butylthiol |
|
11.1 |
2.0 |
ð 10 |
a k1, obs D -d[nitrosobenzene]/dt Ð [nitrosobenzene] 1 Ð [RSH] 1.
bk1, thiolate D -d[nitrosobenzene]/dt Ð [nitrosobenzene] 1 Ð [RS ] 1. c pH 7.4, 25 ° C, carbon monoxide.
reverse reaction are affected by pH in the same manner so that the equilibrium itself is
unimpaired†.
The semimercaptal formation rates of substituted nitrosobenzenes reacting with GSH25,30,36 and Hb-SH27,40, respectively, have been shown to obey a Hammett correlation. As far as -electron donors are not considered, a good fit is achieved using Hammett constants [k1GSH D C1.930]. However, inclusion of definite - electron donor substituents such as o- or p-alkoxy or dialkylamino groups require the Hammett C scale25,27,36,40 [ C k1GSH D C2.1, C k1Hb-SH D C1.727] (Figure 1). These substituents significantly slow down the semimercaptal formation rates, presumably as -electron donation results in a second resonance structure (11) disabling the nitrosothiol interaction:
− |
δ+ |
δ− |
+ |
|
− |
− |
|||
|
− |
|
|||||||
R2 N |
N |
O |
|
R2 N |
N |
O |
|
|
|
|
|||||||||
|
|
||||||||
|
− |
− |
|
|
− |
− |
|
|
|
|
|
|
(11) |
|
|
|
|
|
|
† A similar mechanism of addition was presumed for the reaction of nitrosobenzene with OH . However, the resulting adduct is not protonated at the pH of reaction, but additionally deprotonated to the dianion, giving rise to a completely different product pattern3,50.
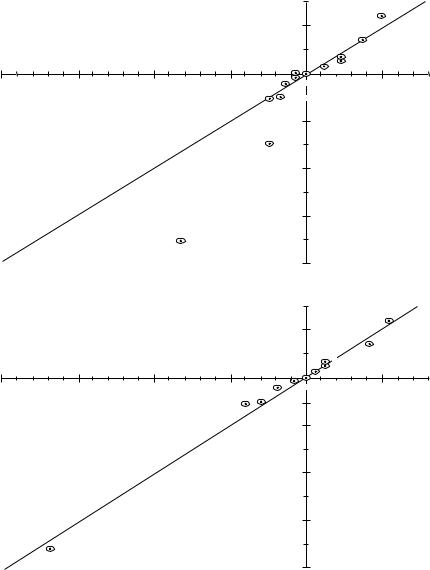
1006 |
P. Eyer and D. Gallemann |
(a)
|
|
σ |
−2 |
−1.5 |
−1 |
ρ = 1.95 (r2 = 0.95)
(b)
|
|
σ + |
−2 |
−1.5 |
−1 |
|
1 |
4-COMe |
|
|
|
|
|
|
|
|
3-Cl |
−0.5 |
|
4-Cl |
0.5 |
|
4-Cl |
||
4-CHOHMe |
|
||
3-OMe |
|
||
|
|
|
|
3.5-Me2 |
3-Me |
|
|
3.4-Me2 |
4-Me |
|
|
|
−1 |
|
|
4-OEt |
|
log |
|
|
|
k |
|
|
|
1 |
|
|
−2 |
k/ |
|
|
0 1 |
|
−3
4-NMe2
|
− 4 |
|
|
1 |
4-COMe |
|
|
|
|
|
3-Cl |
−0.5 |
4-Cl |
0.5 |
4-Cl |
||
|
3-OMe |
|
3.5-Me2 |
3-Me |
|
3.4-Me2 |
4-Me |
|
|
−1 |
|
3-NO2
3-NO2
4-OEt
ρ = 2.01 (r2 = 0.99)
4-NMe2
−2
−3
− 4
0 1 k/1 klog
FIGURE 1. Hammett plots [ (a) and C (b), respectively] for the formation of semimercaptals from GSH and substituted nitrosobenzenes. Log k1/k10 values were calculated from the rate constants reported for pH 7.4, 37 °C18,22,36 and pH 7.49, 25 °C, ionic strength 1 M30. Correction for pH is unnecessary as k1 and k10 are affected in the same manner. Correction for the different temperatures has been revealed to be insignificant. (The Hammett parameter appears to vary with 1/T while seems to be independent of temperature54. Hence, log k1/k10 has been assumed to correlate with 1/T.) For want of
for the complex nitrosochloramphenicol substituent (20), of 4-CHOHMe was used55
23. Reactions of nitrosoarenes with SH groups |
1007 |
This resonance effect is a well-known phenomenon of -donor substituted nitrosoarenes, and the bathoand hyperchromic UV shifts40,52, increased dipole moments2,52 and molecular geometry20,53 have been attributed to it. In addition, molecular orbital calculations have shown a significant decrease of the positive electrostatic potential at the nitrogen atom of the nitroso group in the presence of -donating substituents20 (see footnote in Section II.B.1).
Reverse reaction rates and equilibrium constants of the semimercaptal formation have been shown to fit a Hammett correlation ( -scale), too30. Since an investigation of the latter kinetic parameters has not been possible for nitrosoarenes bearing -donating substituents (because of the kinetic instability of such semimercaptals, see Section II.D), the fit cannot be proved on the C -scale. The Hammett reaction constants were reported to bek 1GSH D 1.4 and K1GSH D C3.230. Thus, electron acceptor groups give rise to rapid establishment of the equilibrium while electron donor groups distinctly slow down this reaction. The relatively high and positive -value of the equilibrium constant (K1) reflects the strong dependence of the semimercaptal equilibrium on the nature of the aryl substituent(s).
C. Formation of the N-Hydroxyarylamine and its Secondary Products
1. N-Hydroxyarylamine
The formation |
of N-hydroxyarylamines (2) has been |
reported for a |
variety |
of nitrosoarenes |
reacting with thiols18,24,33,36,38,41,43. In |
the case of |
electron |
acceptor substituted nitrosoarenes, N-hydroxyarylamines are usually the main end products22,25,28,29,36,41. In contrast, -donor substituted nitrosobenzenes have not been observed to form N-hydroxyarylamines25,36,38,39,56. Similarly, N-hydroxy derivative formation could not be detected during the reaction of the electron-rich 1-methyl- 2-nitrosoimidazole with GSH57. Thus, the electronic effects of the aryl residue play a crucial role not only for the initial reaction rates, but also for the further reaction pathways. In addition, N-hydroxyarylamine formation is substantially affected by the thiol concentration and the pH of the solvent. At an educt stoichiometry of 1:1, N-hydroxyarylamines were hardly detected3,18,22,29,38,43 . From this point of stoichiometry, increasing thiol proportions were found to result in increasing yields of the N-hydroxyarylamines18,22,28,29,36,38,43 . In the case of electron acceptor substituted nitrosoarenes, stoichiometric amounts of thiol disulfide are formed18,22,36. Thus, the N- hydroxyarylamines have been presumed to be formed from the labile semimercaptal by thiolytic cleavage of the N S bond18,22,24,25,28,29,33,36 (see Scheme 1).
2. The bifurcation at the semimercaptal stage
Some detailed work has been done to investigate the dependence of branching ratios at the semimercaptal step on thiol concentration, pH, and the nature of the aryl substituent(s). Thus, Eyer and coworkers18,22,25,36 determined product ratios of the stable end products, N-hydroxyarylamine (2) and sulfinamide (4), while Kazanis and McClelland30 conducted kinetic investigations. Not included in all these studies were-donor substituted nitrosoarenes, as their semimercaptals form a variety of additional products36 (see Scheme 1). Furthermore, as already mentioned in Section II.B.2, the establishment of the semimercaptal equilibrium is distinctly slowed down by these substituents. Besides, consecutive reactions are accelerated, thereby obscuring the biphasic reaction kinetics. Thus, a sufficient kinetic distinction between (fast) semimercaptal formation and (slower) subsequent reactions is not feasible (see equation 1). This effect is
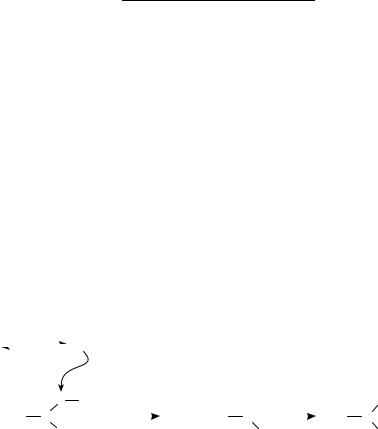
1008 |
P. Eyer and D. Gallemann |
also displayed by sterically hindered thiols as t-butylthiol since kinetics of the initial step are drastically slowed down38 (cf Table 2). [Reaction kinetics of nitrosoarenes with CI- groups can be corrected for incomplete establishment of the semimercaptal equilibrium as shown by Kazanis and McClelland30].
Observation of the slow kinetic component at the maximum absorption of the semimercaptals revealed a pseudo first-order decrease, consisting of a thiol-dependent and a thiol-independent component30:
k2(slow) D k2RSH Ð [RSH] C k2(rearr) |
2 |
The first term obviously reflects the thiol-dependent N-hydroxyarylamine formation while the second term stands for the rearrangement to the sulfinamide (see Scheme 1). Correspondingly, product ratios were found to correlate with thiol concentrations in the following manner22,25:
[N-hydroxyarylamine] |
|
K1 Ð [NOAr] Ð [RSH] Ð k2RSH Ð [RSH] |
|
p |
|
[RSH]; |
|||
[sulfinamide] |
|
D K1 Ð [NOAr] Ð [RSH] Ð k2(rearr) |
D |
Ð |
|||||
|
|
||||||||
p D |
k2RSH |
|
|
|
(3) |
||||
k2(rearr) |
|
|
|
|
At constant pH, the parameter p is dependent on the electronic effects of the nitrosoarene substituent(s). Using only definite acceptor substituted nitrosoarenes, a Hammett correlation on the scale was obtained25. Separate investigation of k2RSH and k2(rearr) for a wider selection of nitrosoarenes reacting with GSH revealed reasonable correlation with Hammett constants only for k2RSH ( D C1.4). However, k2(rearr) was found to fit better on the Hammett C scale ( C D 3.5 30 [for further discussion of k2(rearr) see Section II.D.1.b].
Substantial dependence of the bifurcation ratio p on pH, buffer concentration and tem-
perature was noticed, too18,25,30,33. For the thiol-dependent reaction, a linear correlation of log k2RSH with pH was obtained (slope D 0.9), indicating the thiolate to be the reacting
species30. A similar dependence of k2RSH on the thiol pKa is to be expected, but, except for a few indications33, detailed data are lacking hitherto. General acid-base catalysis could be excluded, and a distinct negative entropy of activation was found. Thus, the detailed mechanism of N-hydroxyarylamine formation seems to proceed via a nucleophilic attack of the thiolate anion at the semimercaptal sulfur atom, forming a properly orientated transition state. The subsequent displacement of the N-hydroxyarylamine anion is probably not assisted by proton transfer, as indicated by the absence of buffer catalysis and the positive Hammett value30 (Scheme 3).
RSH |
Ka(RSH) |
RS− |
|
|
|
|
|
|
|
|
|
|
|
|
− − |
|
|
||
|
S |
R |
k2 |
RSH |
|
H |
|||
|
Ar N |
|
|
|
RSSR + Ar |
N |
|
Ar N |
|
|
|
|
|
|
|||||
|
OH |
|
|
|
|
OH |
|
OH |
SCHEME 3. Mechanism of N-hydroxyarylamine formation (from Kazanis and McClelland30, with modification)