

23. Reactions of nitrosoarenes with SH groups |
1029 |
detrimental. The reported beneficial effects of added thiols on the testicular toxicity of 3-nitrosonitrobenzene thus remain to be explained. One can speculate that stable protein adducts of a semimercaptal structure may be cleaved by low molecular thiols, followed by restitution of the protein sulfhydryl by another low molecular thiol. Similarly enigmatic is the fact that only 1,3-dinitrobenzene, but not 1,2- or 1,4-dinitrobenzenes, elicit the observed testicular toxicity157.
8. 4-Nitrosophenetol from 4-phenetidine
4-Phenetidine (4-ethoxyaniline) is one of the metabolites of the previously widely used analgesic/antipyretic drug phenacetin which has been accused to cause methemoglobinemia, particularly in infants, and serious kidney lesions following long-term treatment. In addition, phenacetin has been made responsible for an increased rate of cancer in the urinary tract. Hence, phenacetin has been displaced from analgesic formulations in most countries. Neither phenacetin nor 4-phenetidine is toxic per se, but the latter is a suitable substrate for several drug metabolizing enzymes, such as cytochrome P-450s, peroxidases and prostaglandin synthase (for literature see Reference 158), and even for oxyhemoglobin144.
N-Hydroxy-4-phenetidine and its autoxidation product 4-nitrosophenetol formed during cytochrome P-450 oxidation of 4-phenetidine were attributed to the methemoglobinemia and hemolysis observed159 162. The mechanism of ferrihemoglobin formation, however, is much more complicated than considered formerly163 165. Moreover, N-hydroxy-4- phenetidine binds to DNA72 and is directly mutagenic166,167, whereas 4-nitrosophenetol
did not react with DNA72 but binds covalently to proteins, predominantly to sulfhydryl groups40,168.
The reaction of 4-nitrosophenetol with thiols is extremely complex as deduced in Section II. Formation of the semimercaptal39, the sulfinamide36 and the sulfenamide72 have been described. Formation of GSSG was clearly observed (0.44 mM) when 4- nitrosophenetol (1 mM) was allowed to react with GSH (2 mM) under physiological conditions36. On the other hand, GSSG hardly increased when isolated rat hepatocytes were exposed to 0.2 mM 4-nitrosophenetol while GSH had dropped markedly and resulted in cell death158. Similarly, total GSH of isolated perfused rat livers was depleted by 4- nitrosophenetol with a small increase in GSSG and mixed glutathione/protein disulfides. The bile flow of the livers was significantly inhibited and the excretion mechanism for GSSG impaired169. These results suggested that glutathione adducts might be formed that compete with the GSSG excretion mechanism25. Interestingly, transport systems of glutathione conjugates in human red cells were similarly inhibited by metabolites of 4-nitrosophenetol170. Possible candidates for this inhibitory action are two thio ethers, 4-ethoxy-2-(glutathion-S-yl)-aniline (7) and 4-amino-40 -ethoxy-2-(glutathion-S-yl)- diphenylamine (17)68.
When 4-nitrosophenetol reacted with human red cells formation of acid-stable hemoglobin adducts was observed. The amount of these adducts was markedly increased when the reduction of GSSG was inhibited. These findings suggest GSH-mediated formation of the sulfenamide cation that is not consumed by further GSH-mediated reactions but is available for ring addition reactions56.
9. 4-Nitroso-N ,N -dimethylaniline
4-Nitroso-N,N-dimethylaniline has been reported to be bactericidal and mutagenic to Salmonella typhimurium TA 100 tester strains. In addition, this compound has proved to

1030 |
P. Eyer and D. Gallemann |
be carcinogenic to male mice and rats171. 4-Nitrosodimethylaniline was shown to be cytotoxic to isolated rat hepatocytes and to deplete cellular GSH. Pretreatment with diethylmaleate, which reduced hepatocyte GSH by 85%, enhanced 4-nitrosodimethylaniline toxicity172,173.
The reaction of 4-nitrosodimethylaniline with GSH is quite slow and leads to formation of N,N-dimethyl-p-phenylenediamine and 2 molecules GSSG together with hitherto unknown glutathione conjugates36. The disappearance of 4-nitrosodimethylaniline in the presence of GSH was clearly enhanced by glutathione S-transferase. No attempts have been reported on isolation of such a reaction product172. Formation of quite stable glutathione S-conjugates derived from 4-nitrosodimethylaniline have already been suggested to explain the inhibitory effect on biliary GSSG excretion25. It should be noted that the N,N-dimethyl-p-quinone diiminium cation, which is easily formed during autoxidation of N,N-dimethyl-p-phenylenediamine, also reacts rapidly with GSH with formation of a typical Michael addition product174.
These data show that 4-nitrosodimethylaniline can deplete GSH in the liver by multiple reactions that are not exclusively related to the nitroso function, and it appears that the slow reactivity towards thiols correlates with the strongly negative Hammett constant
pC D 1.755 .
C. Polycyclic Nitrosoaromatics
1. 4-Nitrosobiphenyl from 4-aminobiphenyl
4-Aminobiphenyl (25) is a carcinogenic aromatic amine detected in cigarette mainstream and sidestream smoke and found to increase the risk of smokers for bladder cancer. In addition, 4-aminobiphenyl induces methemoglobinemia in various animal species. A prerequisite for these toxic actions appears to be N-hydroxylation175. The extent of covalent binding of radiolabelled 4-aminobiphenyl to rat hemoglobin was extraordinarily high and amounted to 5% of the dose103. This high binding index was correlated with an exceptionally high rate of N-oxygenation of 4-aminobiphenyl by rat liver microsomes176.
NH2
(25)
The fate of N-hydroxy-4-aminobiphenyl in rat erythrocytes in vitro was studied in more detail177. It was found that both N-hydroxy-4-aminobiphenyl and 4-nitrosobiphenyl had rapidly disappeared while ferrihemoglobin formation still proceeded. Acid treatment of the hemolysate liberated only one third of the expected 4-aminobiphenyl, indicating a binding type different from a sulfinamide linkage. 3-Hydroxy-4-aminobiphenyl was considered a probable ferrihemoglobin-forming candidate which, after oxidation to an o-quinonimine, may form an acid-stable conjugate with hemoglobin146.
Upon reaction of 4-nitrosobiphenyl with thiols, its negative Hammett constant pC D0.1855 is expected to facilitate formation of a sulfenamide cation that may delocalize its positive charge partly to C 3 , C 20 and C 40 . Hence formation of aminophenols and acid-stable hemoglobin adducts would be conceivable.
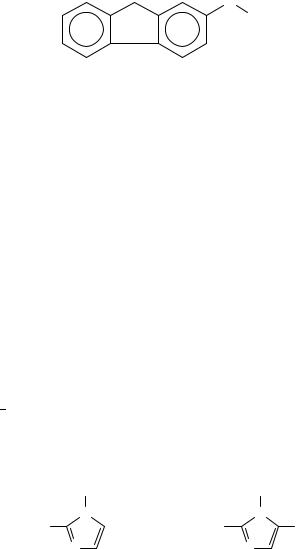
23. Reactions of nitrosoarenes with SH groups |
1031 |
2. 2-Nitrosofluorene from 2-acetamidofluorene
2-Acetamidofluorene (26), which was initially intended to be used as an insecticide178, is one of the most extensively studied chemical carcinogens. This aromatic amide as well as its amine and nitro derivatives induce tumors in a wide variety of sites, including liver, urinary bladder, mammary gland, intestine and forestomach. The initial activation involves the formation of N-hydroxy intermediates179,180.
H
N
COMe
(26)
The observation that 2-nitrosofluorene, under physiological conditions, readily reacts with GSH to yield a water-soluble product that liberated 2-aminofluorene upon mild acid treatment is probably the first report in the literature pointing to sulfinamide formation. At that time, however, no further attempts were made to identify this compound9. Formation of two glutathione conjugates from 2-nitrosofluorene was reported later on35. These authors succeeded in isolation and identification of N-(glutathion-S-yl)-2-aminofluorene- S-oxide (sulfinamide) and N-(glutathion-S-yl)-2-aminofluorene (sulfenamide). These conjugates made up >90% of the 2-nitrosofluorene applied (10 mM GSH, pH 7), indicating that hydroxylamine formation is a minor pathway if at all. This behavior
can be expected, considering the strong -donor character of the fluorenyl moiety30pC D 0.4855 .
D. Heterocyclic Nitrosoaromatics
1. Nitrosoimidazoles from nitroimidazoles
Misonidazole [27; 1-methoxy-3-(2-nitroimidazol-1-yl)-2-propanol] and the model compound 1-methyl-2-nitroimidazole have been used as radiosensitizers in the treatment of certain types of human tumors. One important property of these compounds is that they are more toxic to hypoxic cells than to aerobic cells, indicating that reductive metabolism of the drug is involved in the toxicity. Results of a number of studies suggest that intracellular thiols play a significant role in the hypoxic cell toxicity, and it was found that reduction products formed stable thio ethers with GSH (for literature see References 181 183). The reaction mechanism of thio ether formation has not been fully established. It has been suggested that the 4-electron reduction product was involved in thio ether formation181,184,185, and that the hydroxylamine rather than the nitroso derivative was the reactant. On the other hand, an intermediate nitroso derivative is expected to give a sulfenamide cation (see Scheme 1) which easily allows thio ether formation.
CH2 CHOHCH2 OMe |
|
CH2 CH2 OH |
N |
|
N |
O2 N |
Me |
NO2 |
N |
|
N |
(27) |
|
(28) |

1032 P. Eyer and D. Gallemann
An extensive proposal of the underlying reaction mechanisms has been presented by McClelland’s group57,186,187.
Unlike phenylhydroxylamine, these hydroxylamines do not exhibit an acid-catalyzed N O cleavage as in the Bamberger rearrangement. In contrast, N O cleavage becomes predominant at neutral pH when the imidazole nitrogen is no longer protonated. The resulting nitrenium ion is considerably stabilized since the positive charge is highly delocalized into the imidazole system under predominant formation of an imminium ion. Nucleophilic attack of GSH to the resonance contributing carbenium ion at the C 5 atom easily explains formation of a stable thio ether30,187. Noyce188 has determined C constants defining the accelerating effect of heterocyclic ring systems in reactions that result in the formation of a carbenium ion center adjacent to the ring. The C values so obtained were0.82 for the 1-methyl-2-imidazole and 1.02 for the 1-methyl-5-imidazole group188. It has been predicted that N-hydroxy-4-anisole C D 0.7855 should have a reactivity similar to that of 2-(hydroxylamino)-1-methylimidazole, thereby undergoing a neutral Bamberger rearrangement187. In fact, the structural analogue N-hydroxy-4-phenetidine quickly yielded high amounts of 4-aminophenol when kept in phosphate buffer at pH 7.4 under argon189.
When 1-methyl-2-nitrosoimidazole became available190 it was found that addition of excess GSH to solutions of 1-methyl-2-nitrosoimidazole led to a rapid loss of the characteristic absorbance at 360 nm within a few seconds. Preliminary experiments suggested that formation of GSSG and the hydroxylamine was followed by formation of stable thio ethers. It should be noted, however, that detection of free hydroxylamine was unsuccessful57. In cell-free systems 1-methyl-2-nitrosoimidazole reacted with excess GSH to form adducts in a 1:3 stoichiometric reaction191.
1-Methyl-2-nitrosoimidazole was by two orders of magnitude more toxic to CHO cells than the nitro and hydroxylamine compound. Circumstantial evidence suggested that GSH might reduce cytotoxicity190. Similar observations were reported with HT-29 human colon cancer cells. Depletion of cellular GSH with buthionine sulfoximine before incubation with the nitrosoimidazole resulted in enhanced susceptibility192. Since the DNA damage by the nitrosoimidazole in HT-29 colon cancer cells was probably not a result of a direct interaction of the nitroso compound, a possible activating effect of GSH to yield the ultimate electrophile was discussed193. Interestingly, mixtures of the nitrosoimidazole with GSH gave rise to DNA strand breaks in the plasmid assay194. These data suggest that 1-methyl-2-nitrosoimidazole is responsible for the cytotoxicity elicited by 1-methyl- nitroimidazole191. Whether the hydroxylamine-derived nitrenium/carbenium ion and/or the nitroso-derived sulfenamide cation are responsible for the DNA effects remains to be elucidated.
Metronidazole [28; 1-(2-hydroxyethyl)-2-methyl-5-nitroimidazole] and other 1-alkyl- 5-nitroimidazoles are important antibiotics for the treatment of anaerobic bacterial and protozoal infections. Evidence suggests that their activity is due to inhibition of the DNA function and that reduction of the nitro group is required for the antiparasitic activity of the drugs195. They are also bacterial mutagens and rodent tumorigens (for literature see Reference 196). The 2-electron reduction model compound 1-methyl-4- phenyl-5-nitrosoimidazole was found to have properties consistent with the biologically active form of a 5-nitroimidazole and to bind to DNA, but at a rate too slow to account for its bactericidal effect. In the presence of physiological concentrations of thiols such as cysteine and GSH, however, binding to lambda-phage DNA and polynucleotides was enhanced by 2 3 orders of magnitude, which was quantitatively sufficient to account for its bactericidal effect197. The authors concluded that a semimercaptal-like intermediate might yield a highly reactive cation that binds to DNA. These data suggest that
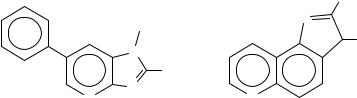
23. Reactions of nitrosoarenes with SH groups |
1033 |
the nitrosoimidazole might still be a penultimate reactive intermediate in the bioactivation of nitroimidazoles which by interaction with thiols would give the ultimate reactive species that binds to DNA. Whether a semimercaptal-type intermediate is formed, which upon loss of the hydroxyl group may produce a sulfenamide cation, or whether a neutral Bamberger rearrangement of the hydroxylamine species occurs, has to await further investigations.
2. Heterocyclic N-oxygenated compounds derived from food mutagens
In the past 15 years, analyses of pyrolyzed amino acids and proteins198 and of cooked protein-containing foods199 have led to the discovery of several classes of highly mutagenic heterocyclic aromatic amines. The most common class of mutagens in foods in the Western diet appears to be aminoimidazoazaarenes, characterized by having 1 or 2 heterocyclic rings fused to an aminoimidazo ring. This class of compounds was also obtained in model reactions by heating creatin(in)e (from muscle) together with an amino acid.
2-Amino-1-methyl-6-phenylimidazo[4,5-b]pyridine (29; PhIP), usually the most abundant product of food-derived mutagens, is formed by heating creatine and phenylalanine at 200 °C200. This compound is modestly mutagenic in the Ames test, but is a potent carcinogen in rats and mice, causing breast and colon cancers.
|
NH2 |
|
N |
|
Me |
|
Me |
|
N |
|
NH2 |
N |
N |
N |
|
(29) |
(30) |
PhIP is a hydrophobic procarcinogen that is inactive per se, but is metabolized in vivo to highly reactive electrophiles that bind covalently to DNA (for literature see Reference 201). The initial bioactivation step responsible for PhIP-DNA adduct formation appears to be the N-oxidation of PhIP to form N-hydroxy-PhIP which undergoes further activation to yield N-acetoxy, N-sulfonyloxy and N-glucuronide derivatives. Using isolated rat hepatocytes it was shown that pretreatment of the hepatocytes with 1-bromoheptane and buthionine sulfoximine, depleting GSH and preventing its resynthesis, respectively, resulted in a 15-fold increase in the formation of PhIP-DNA adducts, as well as in a high level of unscheduled DNA synthesis202,203. These data suggest that GSH may either intervene in DNA binding of reactive metabolites, e.g. by reaction with the putative nitrenium ion, or inhibit formation of the activated N-hydroxy conjugate. Interestingly, formation of an unstable N-(glutathion-S-yl)-PhIP (sulfenamide) was observed on reacting N-acetoxy-PhIP with GSH. The conjugate disappeared upon further purification to yield the stable end-product 5-hydroxy-PhIP84. These data suggest that the intermediate sulfenamide may lead to a nitrenium ion which is in resonance with a highly electrophilic carbenium form85. In this pathway GSH provides a metastable conjugate but not a detoxification product. No details on possible reaction pathways have been presented hitherto.
2-Amino-3-methylimidazo[4,5-f]quinoline (30; IQ) is formed at modest yields by heating creatinine together with an amino acid (glycine, serine, phenylalanine) at 200 °C200.

1034 |
P. Eyer and D. Gallemann |
This compound requires metabolic activation by liver microsomes to yield highly mutagenic derivatives in the Ames test204. In addition, IQ is a multipotent animal carcinogen
that is metabolized by prostaglandin-H synthase205 and the hepatic cytochrome P-450 system204.
Blood protein binding of IQ was found in rats dosed intragastrally with the labelled compound. The same adducts, though in much higher yields, were found when purified rat serum albumin was exposed either to N-hydroxy-IQ or incubated with parent IQ in the presence of a microsomal system. A tripeptide was isolated which contained N-(cystein-S-yl)-IQ-S-oxide (sulfinamide) that easily liberated IQ on acidification. Pretreatment of albumin with p-chloromercuribenzoate reduced covalent binding drastically61. The authors concluded that the reactant most likely to yield this structure is 2-nitroso-3-methylimidazo[4,5-f]quinoline, which is probably formed by autoxidation of N-hydroxy-IQ.
The 3-amino-1-methyl-5H-pyrido[4,3-b]indole derivatives (31; Trp-P-1) and (32; Trp- P-2) were found as tryptophane pyrolysates in broiled fish and meat and in pyrolysates of protein and amino acids by Sugimura and coworkers198. These mutagens are heterocyclic amines and exhibit mutagenicity in the Ames test supplemented with S-9 mix198. The pyridoindole derivatives Trp-P-1 and Trp-P-2 are N-hydroxylated at the exocyclic amino group to form proximate reactive compounds.
|
Me |
|
Me |
|
N |
|
N |
N |
NH2 |
N |
NH2 |
H |
Me |
H |
|
|
|
|
|
(31) |
|
(32) |
|
Thiols, particularly GSH, modify the mutagenic activity and covalent binding to DNA by at least two mechanisms. The first one involves glutathione S-transferase which, in the case of N-hydroxy-Trp-P-2, produced a stable C-conjugate and two labile N- conjugates, one of which decomposed into Trp-P-2 while the other liberated the parent N-hydroxy-Trp-P-2. This latter conjugate was found to be outstandingly mutagenic41,204. The second pathway starts with the nitroso derivative leading to the putative sulfenamide and sulfinamide41. Alternatively, both labile compounds could also be stereoisomeric sulfinamides, particularly since they showed nearly identical UV maxima.
N |
NH2 |
N |
NH2 |
N |
|
N |
|
N |
|
N |
|
Me |
|
|
|
(33) |
|
(34) |
|
The 2-amino-dipyrido[1,2-a:30,20 -d]imidazoles (33; Glu-P-1) and (34; Glu-P-2) were first isolated from glutamic acid pyrolysates. As observed with Trp-P-1 and Trp-P-2,
23. Reactions of nitrosoarenes with SH groups |
1035 |
mutagenic activity was shown after microsomal N-oxygenation at the exocyclic amino group to yield the reactive N-hydroxy and nitroso derivatives204. Most interestingly, upon reaction of nitroso-Glu-P-1 with GSH also an N-hydroxysulfonamide was apparently formed besides sulfinamide, sulfenamide and arylamine37,43 (see Section II.E).
IV. REFERENCES
1.Y. Furuya, I. Urasaki, K. Itoho and A. Takashima, Bull. Chem. Soc. Jpn., 42, 1922 (1969).
2.J. H. Boyer, in The Chemistry of the Nitro and Nitroso Groups (Ed. H. Feuer), Interscience Publishers, New York, 1969, p. 215.
3.P. Zuman and B. Shah, Chem. Rev., 94, 1621 (1994).
4.E. Bamberger and A. Rising, Chem. Ber., 34, 228 (1901).
5.F. J. Smentowski, J. Am. Chem. Soc., 85, 3036 (1963).
6.E. Gulbaran,¨ Finish Chem. J., 37, 229 (1964).
7.E. Y. Belyaev, L. M. Gornostaev and G. A. Suboch, Khim. Tekhnol. Polim., 4, 60 (1975).
8.E. Boyland, P. L. Grover and D. Manson, Ann. Rep. Brit. Emp. Cancer Campaign, 44, 2 (1966).
9.P. D. Lotlikar, E. C. Miller, J. A. Miller and A. Margreth, Cancer Res., 25, 1743 (1965).
10.M. Kiese and K. Taeger, Naunyn-Schmiedeberg’s Arch. Pharmakol., 292, 59 (1976).
11.H. -G. Neumann, M. Metzler and W. Topner,¨ Arch. Toxicol., 39, 21 (1977).
12.D. Manson, J. Chem. Soc., Perkin Trans. 1, 192 (1974).
13.M. Kiese, Angew. Chem., 70, 93 (1958).
14.M. Kiese, Naunyn-Schmiedeberg’s Arch. Exp. Pathol. Pharmacol., 235, 354 (1959).
15.M. Kiese and H. Uehleke, Naunyn-Schmiedeberg’s Arch. Exp. Path. Pharmacol., 242, 117 (1961).
16.M. Kiese, Pharmacol. Rev., 18, 1091 (1966).
17.M. Kiese, D. Reinwein and H. D. Waller, Arch. Exp. Path. Pharmacol., 210, 393 (1950).
18.P. Eyer, Chem.-Biol. Interact., 24, 227 (1979).
19.P. Eyer and E. Lierheimer, Xenobiotica, 10, 517 (1980).
20.P. Politzer and R. Bar-Adon, J. Phys. Chem., 91, 2069 (1987).
21.R. D. Youssefyeh, J. Chem. Soc., Perkin Trans. 1, 1857 (1975).
22.P. Eyer and M. Schneller, Biochem. Pharmacol., 32, 1029 (1983).
23.P. Eyer, E. Lierheimer and M. Schneller, Biochem. Pharmacol., 33, 2299 (1984).
24.H. Klehr, P. Eyer and W. Schafer,¨ Biol. Chem. Hoppe-Seyler, 366, 755 (1985).
25.P. Eyer, in Biological Oxidation of Nitrogen in Organic Molecules: Chemistry, Toxicology and Pharmacology (Eds. J. W. Gorrod and L. A. Damani), Horwood, Chichester, 1985, p. 386.
26.H. Klehr, P. Eyer and W. Schafer,¨ Biol. Chem. Hoppe-Seyler, 368, 895 (1987).
27.P. Eyer, Xenobiotica, 18, 1327 (1988).
28.A. E. Cribb, M. Miller, J. S. Leeder, J. Hill and S. P. Spielberg, Drug Metab. Dispos., 19, 900 (1991).
29.M. K. Ellis, S. Hill, P. Matthew and D. Foster, Chem.-Biol. Interact., 82, 151 (1992).
30.S. Kazanis and R. A. McClelland, J. Am. Chem. Soc., 114, 3052 (1992).
31.P. Eyer, Environ. Health Perspect., 102 (Suppl. 6), 123 (1994).
32.H. P. T. Ammon, C. J. Estler and F. Heim, Biochem. Pharmacol., 16, 769 (1967).
33.B. Dolle,¨ W. Topner¨ and H. -G. Neumann, Xenobiotica, 10, 527 (1980).
34.P. Eyer, H. Kampffmeyer, H. Maister and E. Rosch¨-Oehme, Xenobiotica, 10, 499 (1980).
35.G. J. Mulder, L. E. Unruh, F. E. Evans, B. Ketterer and F. F. Kadlubar, Chem.-Biol. Interact.,
39, 111 (1982). |
|
|
|
|
|
|
|
36. C. Diepold, |
P. Eyer, H. Kampffmeyer |
and |
K. Reinhardt, |
in |
Biological |
Reactive |
|
Intermediates |
|
II. Chemical Mechanisms |
and |
Biological |
Effects |
(Eds. |
R. Snyder, |
|
|||||||
D. V. Parke, |
J. J. Kocsis, D. J. Jollow, C. G. Gibson and C. M. Witmer), Plenum Press, |
New York, 1982, p. 1173.
37.K. Saito and R. Kato, Biochem. Biophys. Res. Commun., 124, 1 (1984).
38.H. Klehr, PhD Thesis, Ludwig-Maximilians-Universitat¨ Munchen,¨ 1988.
39.H. Klehr and P. Eyer, Naunyn-Schmiedeberg’s Arch. Pharmacol., 335 (Suppl.), R 12 (1987).
40.P. Eyer and M. Ascherl, Biol. Chem. Hoppe-Seyler, 368, 285 (1987).
41.K. Saito, Y. Yamazoe, T. Kamataki and R. Kato, Carcinogenesis, 4, 1551 (1983).
42.H. -O. Kalinowski, S. Berger and S. Braun, 13C-NMR-Spektroskopie, Thieme Verlag, Stuttgart, 1984.
1036 |
P. Eyer and D. Gallemann |
43.A. Umemoto, S. Grivas, Z. Yamaizumi, S. Sato and T. Sugimura, Chem.-Biol. Interact., 68, 57 (1988).
44.D. L. Rabenstein and D. A. Keire, in Glutathione. Chemical, Biochemical, and Medical Aspects, Vol. A (Eds. D. Dolphin, O. Avramovic and R. Poulson), Wiley, New York, 1989, p. 67.
45.D. Gallemann, A. Speckmann and P. Eyer, in preparation.
46.R. E. Benesch and R. Benesch, Biochemistry, 1, 735 (1962).
47.G. Geraci and L. J. Parkhurst, Biochemistry, 12, 3414 (1973).
48.Y. M. Torchinsky, Sulfur in Proteins, Pergamon Press, Oxford, 1977, p. 133.
49.M. R. Crampton, in The Chemistry of the Thiol Group, Vol. 2 (Ed. S. Patai), Wiley, London, 1974, p. 379.
50.P. Zuman and Z. Fijalek, J. Org. Chem., 56, 5486 (1991).
51.L. H. Bothelo and F. R. N. Gurd, in Practical Handbook of Biochemistry and Molecular Biology
(Ed. G. D. Fasman), CRC Press, Boca Raton, 1992, p. 359.
52.B. G. Gowenlock and W. Luttke,¨ Quart. Rev., 12, 321 (1958).
53.M. Raban and J. Greenblatt, in The Chemistry of Amino, Nitroso and Nitro Compounds and their Derivatives (Ed. S. Patai), Wiley, Chichester, 1982, p. 53.
54.C. D. Johnson, The Hammett Equation, Cambridge University Press, London, 1973.
55.C. Hansch, A. Leo and R. W. Taft, Chem. Rev., 91, 165 (1991).
56.D. Gallemann, PhD Thesis, Ludwig-Maximilians-Universitat¨ Munchen,¨ 1993.
57.S. F. Farah, PhD Thesis, University of Toronto, 1991.
58.J. March, Advanced Organic Chemistry: Reactions, Mechanisms, and Structure, 2nd ed., McGrawHill, Tokyo, 1977.
59.R. A. Yunes, A. J. Terenzani and L. do Amaral, J. Am. Chem. Soc., 97, 368 (1975).
60.J. P. Uetrecht, J. Pharmacol. Exp. Ther., 232, 420 (1985).
61.R. J. Turesky, P. L. Skipper and S. R. Tannenbaum, Carcinogenesis, 8, 1537 (1987).
62.J. G. Tillett, in The Chemistry of Sulphinic Acids, Esters and their Derivatives (Ed. S. Patai), Wiley, Chichester, 1990, p. 603.
63.D. Gallemann, A. Speckmann and P. Eyer, unpublished result.
64.R. M. Moriarty, J. Org. Chem., 30, 600 (1965).
65.R. E. Booms and D. J. Cram, J. Am. Chem. Soc., 94, 5438 (1972).
66.E. L. Eliel and S. H. Wilen, Stereochemistry of Organic Compounds, Wiley, New York, 1994, p. 430.
67.D. Kost and M. Raban, in The Chemistry of Sulphenic Acids and their Derivatives (Ed. S. Patai), Wiley, Chichester, 1990, p. 23.
68.D. Gallemann and P. Eyer, Environ. Health Perspect., 102 (Suppl. 6), 137 (1994).
69.L. Stryer, Biochemie, 4th ed., Spektrum der Wissenschaft, Heidelberg, 1990.
70.R. C. Weast, Handbook of Chemistry and Physics, 54th ed., CRC Press, Cleveland, 1973.
71.S. Darchen and C. Moinet, J. Chem. Soc., Chem. Commun., 820 (1976).
72.G. J. Mulder, F. F. Kadlubar, J. B. Mays and J. A. Hinson, Mol. Pharmacol., 26, 342 (1984).
73.G. Capozzi, G. Modena and L. Pasquato, in The Chemistry of Sulphenic Acids and their Derivatives (Ed. S. Patai), Wiley, Chichester, 1990, p. 403.
74.R. Cecil and J. R. McPhee, Biochem. J., 66, 538 (1957).
75.W. S. Allison, Acc. Chem. Res., 9, 293 (1976).
76.J. R. Prohaska, Biochim. Biophys. Acta, 611, 87 (1980).
77.L.v. den Brock, L. Delbressine and H. Ottenheijm, in The Chemistry of Sulphenic Acids and their Derivatives (Ed. S. Patai), Wiley, Chichester, 1990, p. 701.
78.A. Bagno, S. J. Eustace, L. Johansson and G. Scorrano, J. Org. Chem., 59, 232 (1994).
79.L. Benati, P. C. Montevecchi and P. Spagnolo, J. Chem. Soc., Perkin Trans. 1, 2261 (1985).
80.R. F. Bayfield and E. R. Cole, Phosphorus Sulfur Silicon, 45, 237 (1989).
81.P. de Maria, in The Chemistry of Sulphenic Acids and their Derivatives (Ed. S. Patai), Wiley, Chichester, 1990, p. 293.
82.S. Braverman, in The Chemistry of Sulphenic Acids and their Derivatives (Ed. S. Patai), Wiley, Chichester, 1990, p. 311.
83.L. Craine and M. Raban, Chem. Rev., 89, 689 (1989).
84.R. Reistad, H. Frandsen, S. Grivas and J. Alexander, Carcinogenesis, 15, 2547 (1994).
85.J. H. N. Meerman, F. A. Beland, B. Ketterer, S. K. S. Srai, A. P. Bruins and G. J. Mulder,
Chem.-Biol. Interact., 39, 149 (1982).
86.P. K. Claus, in The Chemistry of Sulphenic Acids and their Derivatives (Ed. S. Patai), Wiley, Chichester, 1990, p. 723.
23. Reactions of nitrosoarenes with SH groups |
1037 |
87.H. Sayo, in The Chemistry of Sulphenic Acids and their Derivatives (Ed. S. Patai), Wiley, Chichester, 1990, p. 571.
88.J. T. Hays, H. L. Young and H. H. Espy, J. Org. Chem., 32, 158 (1967).
89.T. Okuyama, in The Chemistry of Sulphinic Acids, Esters and their Derivatives (Ed. S. Patai), Wiley, Chichester, 1990, p. 639.
90.A. F. Finch, Theilheimer’s Synthetic Methods of Organic Chemistry, Vol. 49, Karger, Basel, 1995, p. 110.
91.N. Takahashi, V. Fischer, J. Schreiber and R. P. Mason, Free Rad. Res. Commun., 4, 351 (1988).
92.K. R. Maples, P. Eyer and R. P. Mason, Mol. Pharmacol., 37, 311 (1990).
93.M. C. R. Symons, W. R. Bowman and P. F. Taylor, Tetrahedron Lett., 31, 3221 (1990).
94.T. P. Bradshaw, D. C. McMillan, R. K. Crouch and D. J. Jollow, Free Rad. Biol. Med., 18, 279 (1995).
95.G. A. Russell, E. J. Geels, F. J. Smentowski, K. Y. Chang, J. Reynolds and G. Kaupp, J. Am. Chem. Soc., 89, 3821 (1967).
96.J. H. Weisburger and E. K. Weisburger, Pharmacol. Rev., 25, 1 (1973).
97.D. E. Rickert, Toxicity of Nitroaromatic Compounds, Hemisphere Publ. Corp., Washington, 1985.
98.D. E. Rickert, Drug Metab. Rev., 18, 23 (1987).
99.J. A. Miller, Drug Metab. Rev., 26, 1 (1994).
100.P. Eyer, H. Hertle, M. Kiese and G. Klein, Mol. Pharmacol., 11, 326 (1975).
101.M. Kiese, Methemoglobinemia: A Comprehensive Treatise, CRC Press, Cleveland, 1974.
102.E. Wieland and H. -G. Neumann, Arch. Toxicol., 40, 17 (1978).
103.L. C. Green, P. L. Skipper, R. J. Turesky, M. S. Bryant and S. R. Tannenbaum, Cancer Res., 44, 4254 (1984).
104.P. B. Farmer, H. -G. Neumann and D. Henschler, Arch. Toxicol., 60, 251 (1987).
105.H. -G. Neumann, IARC Sci. Publ., 89, 157 (1988).
106.G. Sabbioni, Environ. Health Perspect., 102 (Suppl. 6), 61 (1994).
107.G. Sabbioni, Chem. Res. Toxicol., 7, 267 (1994).
108.P. L. Skipper and S. R. Tannenbaum, Environ. Health Perspect., 102 (Suppl. 6), 17 (1994).
109.F. F. Kadlubar, Nature, 360, 189 (1992).
110.H. -G. Neumann, in Metabolic Aspects of Cell Toxicity (Ed. P. Eyer), B. I. Wissenschaftsverlag, Mannheim, 1994, p. 109.
111.H. -G. Neumann, S. Ambs and A. Bitsch, Environ. Health Perspect., 102 (Suppl. 6), 173 (1994).
112.J. H. Harrison and D. J. Jollow, J. Pharm. Exp. Ther., 238, 1045 (1986).
113.P. Eyer, Arch. Toxicol., 6 (Suppl.), 3 (1983).
114.P. Eyer, in Metabolic Aspects of Cell Toxicity (Ed. P. Eyer), B. I. Wissenschaftsverlag, Mannheim, 1994, p. 149.
115.K. Stolze and H. Nohl, Biochem. Pharmacol., 49, 1261 (1995).
116.H. Fujii, B. Zhao, J. Koscielniak and L. J. Berliner, Magn. Reson. Med., 31, 77 (1994).
117.H. Fujii, J. Koscielniak, K. Kakinuma and L. J. Berliner, Free Rad. Res., 21, 235 (1994).
118.E. Beutler, Pharmacol. Rev., 21, 73 (1969).
119.J. Uetrecht and B. Sokoluk, Am. Soc. Pharm. Exp. Ther., 20, 120 (1992).
120.J. P. Uetrecht, Drug Metab. Rev., 24, 299 (1992).
121.J. F. Wheeler, C. E. Lunte, H. Zimmer and W. R. Heineman, J. Pharm. Biomed. Anal., 8, 143 (1990).
122.R. L. Rubin, Clin. Biochem., 25, 223 (1992).
123.W. G. Rice, C. D. Hillyer, B. Harten, C. A. Schaeffer, M. Dorminy, D. A. Lackey, E. Kirsten, J. Mendeleyev, K. G. Buki, A. Hakam and E. Kun, Proc. Natl. Acad. Sci. USA, 89, 7703 (1992).
124.K. G. Buki, P. I. Bauer, J. Mendeleyev, A. Hakam and E. Kun, FEBS Lett., 290, 181 (1991).
125. |
W. G. Rice, |
C. A. Schaeffer, L. Graham, M. Bu, J. S. McDougal, S. L. Orloff, F. Villinger, |
|
M. Young, S. Oroszlan, M. P. Fesen, Y. Pommier, J. Mendeleyev and E. Kun, Proc. Natl. Acad. |
|
|
Sci. USA, 90, 9721 (1993). |
|
126. |
W. G. Rice, |
C. A. Schaeffer, B. Harten, F. Villinger, T. L. South, M. F. Summers, |
|
L. E. Henderson, J. W. Bess, L. O. Arthur, J. S. McDougal, S. L. Orloff, J. Mendeleyev and |
E. Kun, Nature, 361, 473 (1993).
127.X. Yu, Y. Hathout, C. Fenselau, R. C. Sowder, L. E. Hendereson, W. G. Rice, J. Mendeleyev and E. Kun, Chem. Res. Toxicol., 8, 586 (1995).
128.G. Bories and J. -P. Cravedi, Drug Metab. Rev., 26, 767 (1994).
129.A. A. Yunis, A. M. Miller, Z. Salem, M. D. Corbett and G. K. Arimura, J. Lab. Clin. Med., 96, 36 (1980).
1038 |
P. Eyer and D. Gallemann |
130.A. A. Yunis, Ann. Rev. Pharmacol. Toxicol., 28, 83 (1988).
131.M. D. Corbett and B. R. Chipko, Antimicrob. Agents Chemother., 13, 193 (1978).
132.B. L. Gross, R. V. Branchflower, T. R. Burke, D. E. Lees and L. R. Pohl, Toxicol. Appl. Pharmacol., 64, 557 (1982).
133.M. Ascherl, P. Eyer and H. Kampffmeyer, Biochem. Pharmacol., 34, 3755 (1985).
134.D. E. Holt, R. Hurley and D. Harvey, J. Antimicrob. Chemother., 35, 115 (1995).
135.W. H. Abou-Khalil, A. A. Yunis and S. Abou-Khalil, Pharmacology, 36, 272 (1988).
136.J. J. Jiminez, G. K. Arimura, W. H. Abou-Khalil, M. Isildar and A. A. Yunis, Blood, 70, 1180 (1987).
137.C. Lafarge-Frayssinet, S. Robbana-Barnat, C. Frayssinet, L. Toucas and F. Decloitre, Mutation Res., 320, 207 (1994).
138.M. Isildar, W. H. Abou-Khalil, J. J. Jimenez, S. Abou-Khalil and A. A. Yunis, Toxicol. Appl. Pharmacol., 94, 305 (1988).
139.A. Anadon, P. Bringas, M. R. Martinez-Larranaga and M. J. Diaz, J. Vet. Pharmacol. Ther., 17, 52 (1994).
140.I. K. Shih, J. Pharm. Sci., 60, 1889 (1971).
141.H. de Vries, P. J. Hemelaar, A. C. M. Gevers and G. M. J. Beyersbergen van Henegouwen, Photochem. Photobiol., 60, 249 (1994).
142.D. C. McMillan, T. P. Bradshaw, J. A. Hinson and D. J. Jollow, Toxicol. Appl. Pharmacol., 110, 70 (1991).
143.D. C. McMillan, T. A. McRae and J. A. Hinson, Toxicol. Appl. Pharmacol., 105, 503 (1990).
144.W. Lenk and H. Sterzl, Xenobiotica, 7, 581 (1984).
145.I. Golly and P. Hlavica, Biochim. Biophys. Acta, 760, 69 (1983).
146.S. Karreth and W. Lenk, Xenobiotica, 21, 971 (1991).
147.G. Birner and H. -G. Neumann, Arch. Toxicol., 62, 110 (1988).
148.M. D. Coleman and M. D. Tingle, Drug Dev. Res., 25, 1 (1992).
149.W. R. Graham, Int. J. Dermatol., 14, 494 (1975).
150.G. L. Scott and M. R. Rasbridge, Br. J. Haematol., 24, 307 (1973).
151.P. A. Kramer, B. E. Glader and T. -K. Li, Biochem. Pharmacol., 21, 1265 (1972).
152.M. D. Coleman and D. P. Jacobus, Biochem. Pharmacol., 45, 1027 (1993).
153.G. L. Mandell and M. A. Sande, in Goodman and Gilman’s The Pharmacological Basis of Therapeutics, 8th ed. (Eds. A. Goodman Gilman, T. W. Rall, A. S. Nies and P. Tayler), Pergamon Press, New York, 1990.
154.M. J. Rieder, R. Krause, I. A. Bird and G. A. Dekaban, J. Aquired Immunol. Defic. Syndr. Hum. Retrovir., 8, 134 (1995).
155.S. P. Spielberg, J. S. Leeder, A. E. Cribb and H. -M. Dosch, Eur. J. Clin. Pharmacol., 36, A 173 (1989).
156.M. Pirmohamed, M. D. Coleman, F. Hussain, A. M. Breckenridge and B. K. Park, Br. J. Clin. Pharmacol., 32, 303 (1991).
157.M. K. Ellis and P. M. D. Foster, Toxicol. Lett., 62, 201 (1992).
158.T. Lindqvist, P. Moldeus´ and B. Lindeke, Pharmacol. Toxicol., 69, 117 (1991).
159.H. Baader, S. Girgis, M. Kiese, H. Menzel and L. Skrobot, Naunyn-Schmiedeberg’s Arch. Exp. Path. Pharmacol., 241, 317 (1961).
160.H. Uehleke, Naunyn-Schmiedeberg’s Arch. Pharmacol., 264, 434 (1969).
161.T. Fischbach and W. Lenk, Xenobiotica, 15, 915 (1985).
162.C. B. Jensen and D. J. Jollow, Toxicol. Appl. Pharmacol., 111, 1 (1991).
163.D. Gallemann and P. Eyer, Biol. Chem. Hoppe-Seyler, 374, 37 (1993).
164.D. Gallemann and P. Eyer, Biol. Chem. Hoppe-Seyler, 374, 752 (1993).
165.D. Gallemann and P. Eyer, ISSX Proceedings, 4, 115 (1993).
166.K. Shudo, T. Ohta, Y. Orihara, T. Okamoto, M. Nagao, Y. Takahashi and T. Sugimura, Mutation Res., 58, 367 (1978).
167.P. J. Wirth, P. Alewood, I. Calder and S. S. Thorgeirsson, Carcinogenesis, 3, 167 (1982).
168.J. A. Hinson and J. B. Mays, J. Pharmacol. Exp. Ther., 238, 106 (1986).
169.P. Eyer and H. Kampffmeyer, Chem.-Biol. Interact., 42, 209 (1982).
170.D. Gallemann and P. Eyer, Biol. Chem. Hoppe-Seyler, 374, 51 (1993).
171.C. M. Goodall, C. M. Moore and O. B. Stephens, Xenobiotica, 16, 587 (1986).
172.J. M. Silva and P. J. O’Brien, Prog. Pharmacol. Clin. Pharmacol., 8, 301 (1991).
173.J. M. Silva, S. D. Jatoe and P. J. O’Brien, Prog. Pharmacol. Clin. Pharmacol., 8, 289 (1991).