
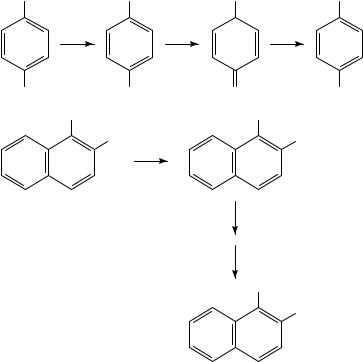
18. The electrochemistry of nitro, nitroso, and related compounds |
847 |
the steric bulk of the t-butyl group in 18 forces the hydroxyl group of the hydroxylamino group out of the aromatic plane, thus reducing the degree of resonance interaction of the nitrogen with the benzene ring and making it easier to cleave the N O bond.
NO2 |
NHOH |
NH |
NH2 |
(4)
OH |
OH |
O |
OH |
|
NO |
|
NHOH |
|
OH |
|
OH |
(5)
NH2
OH
IV. AROMATIC DINITRO COMPOUNDS
The behavior of dinitro compounds is of both synthetic and mechanistic interest. There is obvious synthetic value to selective reduction of a dinitro compound since the product has two readily differentiated groups for subsequent elaboration. One is also interested in the effect which one of the groups has on the other, and how this may change as one group is altered electrochemically. The observed effects depend on the nature of the structural relation between the two groups.
It is necessary at this point to review the behavior of analogous benzenoid species. As mentioned previously nitrobenzene (1) undergoes one-electron reduction to a stable radical anion in aprotic media39. The first polarographic reduction wave of meta-dinitrobenzene (19) is 0.25 V positive of that of 1. This substantial shift (1 V D 23.06 kcal mol 1) is due to the effect of the second inductively electron-withdrawing nitro group on the reduction potential of the first. The second reduction potential of 19, on the other hand, is negative of the reduction potential of 1. This is presumably because in the radical anion formed at the first step the first nitro group now bears a negative charge40 and hence is inductively electron-supplying. Para- and ortho-dinitro benzenes (20 and 21), however, exhibit markedly different behavior. The first reduction potential of 20 is even more positive than that of 19, even though the second nitro group in 20 is further away
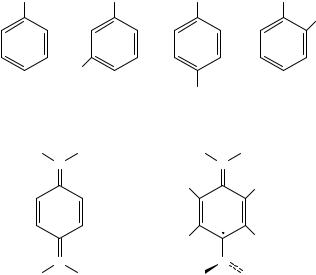
848 |
Albert J. Fry |
from the first than in 19 and should exert less of an inductive effect. Furthermore, the second reduction potential of 20 is positive of that of the reduction potential of neutral nitrobenzene, even though in the case of 20 one is reducing a species already carrying a negative charge. ortho-Dinitrobenzene (21) behaves similarly, although the effects are less dramatic. The anomalous voltammetric behavior of 20 and 21 (and other aromatic compounds bearing unsaturated groups para or ortho to each other)41 has been ascribed7,9 to quinoidal contributions such as 22 to the structure of the dianions and corresponding monoanion radicals, thus providing a means of charge localization and stabilization in such species (presumably the effect is not as great in 21 because the two nitro groups are twisted somewhat out of planarity). The unusual ESR spectrum of the radical anion of 20 was also ascribed to a quinoidal contribution to the structure7. Parker has obtained further evidence for the formation of quinoidal dianions from 20 and 2142 and quantum mechanical calculations (MP2, SCF) show that related monoanions also prefer a quinoidtype structure43. On the other hand, it has been found that the radical anion of 1,4- dinitrodurene prefers a structure in which one nitro is perpendicular and the other is parallel to the plane of the ring44. The resonance stabilization of the quinoidal form is outweighed by the steric repulsions which arise if the nitro groups are both in the plane of the ring. The molecule apparently adopts a compromise in which only one nitro group is in the plane of the ring, thus preserving some stabilization in the semiquinoidal structure 23.
NO2 |
NO2 |
NO2 |
NO2 |
NO2
O2 N
|
|
|
NO2 |
|
(1) |
|
(19) |
(20) |
(21) |
−Ο |
+ O− |
−Ο + |
O− |
|
|
N |
|
N |
|
|
|
H3 C |
|
CH3 |
|
|
H3 C |
|
CH3 |
|
N |
|
N+ |
|
−O |
+ |
O− |
− |
O |
|
O |
|||
|
(22) |
|
(23) |
|
These concepts were recently applied to an understanding of the electrochemical reduction of the mono and dinitro derivatives of the nonbenzenoid hydrocarbon 24a45. Compound 24b exhibits a single one-electron wave at 1.08 V, while dinitro compound 25 exhibits two one-electron waves at 0.88 and 1.05 V46. This behavior is quite similar to that exhibited by ortho-dinitrobenzene (21); it appears therefore that 25 is reduced to a dianion in which the quinoidal structure 26 is an important contributor to the resonance hybrid. The quinoidal structure 11 could be produced from 25 even though the
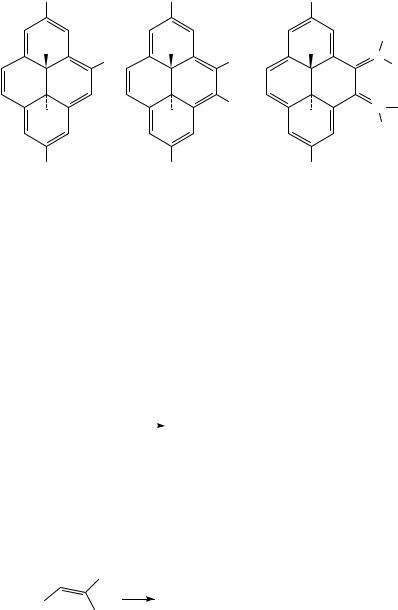
18. The electrochemistry of nitro, nitroso, and related compounds |
|
849 |
||||
t-Bu |
|
t-Bu |
t-Bu |
|
|
|
|
2 |
|
|
|
|
|
1 |
3 |
|
|
O− |
|
|
|
|
|
|
|||
Me |
|
Me |
Me |
+ |
|
|
|
|
X |
NO2 |
N |
− |
|
10 |
|
4 |
|
|
|
O |
|
|
|
|
|
||
9 |
|
5 |
NO2 |
O+ |
|
O− |
Me |
|
|
||||
|
Me |
Me |
|
|
||
8 |
6 |
|
|
O− |
|
|
|
|
|
|
|
||
|
7 |
|
|
|
|
|
t-Bu |
|
t-Bu |
t-Bu |
|
|
|
(24a) |
X = H |
|
(25) |
(26) |
|
|
(24b) |
X = NO2 |
|
|
|
|
|
two nitro groups are twisted out of the plane of the aromatic ring because overlap is still largely preserved between the adjacent ring carbon and nitrogen pi-orbitals in the twisted structure47. Quantum mechanical calculations on 25 and its dianion were used to support these conclusions47.
V. ALIPHATIC NITRO COMPOUNDS
Electrochemical reduction of benzylic nitro compounds (27) in an ethanolic aqueous acetic acid buffer (35:65) affords a mixture of the corresponding oxime and hydroxylamine (equation 6)48. The hydroxylamine can subsequently be oxidized back to the oxime (28) (via the intermediate nitroso compound); conversions as high as 90% can be obtained.
R |
|
R |
|
|
R |
|
|||
|
|
|
|
|
|
NOH + |
|
|
(6) |
ArCHNO2 |
|
ArC |
|
ArCHNHOH |
|||||
|
|
||||||||
|
|||||||||
|
|
(27)(28)
Reduction of ˛,ˇ-unsaturated nitro compounds (29) affords the corresponding oximes (30) in high yields when electrolysis is carried out at 0.4 V (vs SCE) at a mercury or graphite electrode in aqueous isopropanol containing 0.1 M H2SO4 (equation 7)49. Reduction at 1.1 V affords the secondary amine (31) in fair yields. Isolated double bonds elsewhere in the molecule are not affected.
|
NO2 |
R |
R |
|
||||
|
|
|
||||||
Ar |
|
|
|
|
|
|
|
(7) |
R |
ArCH2 C |
|
NOH or |
ArCH2 CHNH2 |
||||
|
||||||||
|
||||||||
|
|
|
|
|
|
|
|
|
(29) |
|
(30) |
|
(31) |
|
Electrochemical reduction of the ˛,ˇ-unsaturated nitro compounds 32 in acetonitrile containing tetraethylammonium tosylate affords the corresponding hydrodimer in 37%
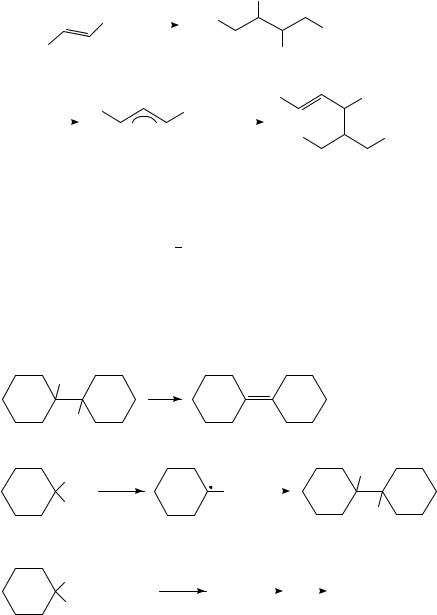
850 |
Albert J. Fry |
yield (equation 8)50. An alternate route more often observed in the electrolysis was depro-
tonation of 32 by an electrogenerated base51 attacks the starting material in a Michael-type
to afford a conjugated carbanion which reaction (equation 9).
|
|
R |
|
NO2 |
2 e− |
O2 N |
|
2 R |
2 H + |
NO2 |
(8) |
|
R |
|
|
(32) |
|
(33) |
|
|
:B − |
R |
NO2 |
1, 32 |
32 |
|
|
− |
|
|
|
2. H + |
||
|
|
|
|
R |
NO2 |
|
(9) |
R |
NO2 |
1,2-Dinitro compounds are reduced to alkenes with elimination of two equivalents of nitrite ion (e.g. 34 to 35 equation 10)52. More surprising, perhaps, is the reductive electrochemical coupling of 1,1-dinitro compounds such as 1,1-dinitrocyclohexane (36) to the corresponding dimeric vicinal dinitro compounds (34, equation 11). The process is initiated by reduction of 36 to a radical anion, which ejects nitrite ion to produce the ˛-nitrocyclohexyl radical (37), coupling of which leads to 348b,52. What happens in the reduction of compounds 38 40, each of which bears an electronegative group bound to the carbon bearing the nitro group, depends upon the nature of the group8b. Compound 38 affords alkene 35; toluenesulfinate ion is ejected from the initial radical anion to produce radical 37, which reacts as shown above to afford 34 and ultimately 35 (equation 12). Reduction of 39 affords a mixture of nitrocyclohexane and cyanocyclohexane (equation 13); nitro ester 40, on the other hand, affords ester 41 (equation 14). Whether it is the nitro group or the hetero atom group which is lost upon reduction apparently depends on both the relative electronegativity of X and the electrolysis potential.
NO2
(10)
O2 N |
|
|
|
|
|
|
|
|
|
|
(34) |
|
|
|
(35) |
|
|
|
|
||
NO2 |
|
|
|
|
|
|
|
|
|
NO2 |
− |
|
|
|
|
|
|
|
|
|
|
e |
|
NO2 |
|
|
|
|
|
|
|
|
−NO2 |
− |
|
|
|
|
|
|
|
||
NO2 |
|
|
|
|
|
|
|
|
|
O2 N |
|
|
|
|
|
|
|
|
|
|
|
(36) |
|
(37) |
|
(34) |
||||||
|
|
|
|
(11) |
||||||
NO2 |
|
e− |
|
|
|
|
|
|
|
|
|
|
(37) |
|
|
|
|
|
|
(35) |
|
|
|
−NO2 − |
|
|
|
|
||||
|
|
|
(12) |
|||||||
SO2 -p-C6 H4 CH3 |
|
|
|
|
|
|
|
|
||
(38) |
|
|
|
|
|
|
|
|
|
|
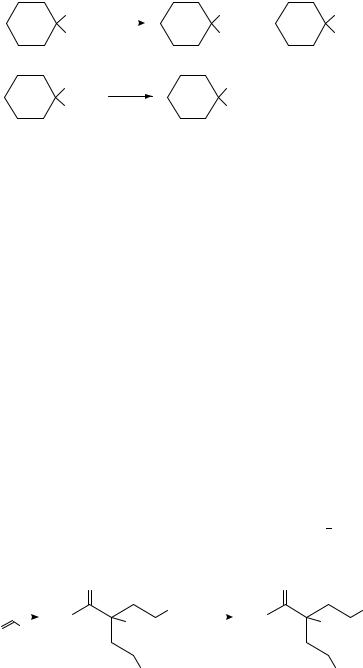
18. The electrochemistry of nitro, nitroso, and related compounds |
851 |
|||||
NO2 |
− |
|
NO2 |
H |
|
|
|
e |
|
+ |
|
|
|
|
−NO2 |
− |
|
(13) |
||
|
H |
CN |
||||
CN |
|
|
|
|||
(39) |
|
|
|
|
|
|
NO2 |
e |
− |
H |
|
|
|
|
|
|
|
|
||
|
−NO2 − |
CO2 Et |
|
(14) |
||
CO2 Et |
|
|
|
|
||
(40) |
|
|
|
(41) |
|
|
Cobalt(III) macrobicyclic polyamine complexes normally exhibit electrochemical behavior analogous to that of nitroarenes, in that electrolysis at pH 0 or 4 affords hydroxylamines, and azoxy compounds at high pH53,54. Their behavior in dry aprotic solvents resembles that of tertiary nitro compounds8 in that reduction affords a radical anion which ejects nitrite ion to afford a tertiary radical. The final product contains a hydrogen atom at the site originally occupied by the nitro group. The same reductive removal of a tertiary nitro group and replacement with hydrogen is observed upon reduction of a nitro derivative of the alkaloid vincristine55.
VI. RELATED PROCESSES
A. Nitro Compounds as Electrogenerated Bases
Reduction of many organic weak acid compounds results in formation of the corresponding carbanions (equation 15). The alkylation of such species represents an attractive synthetic application56. Niyazymbetov and Evans have explored the chemistry of ethyl nitroacetate (42)57. As it happens, reductive cleavage of the ˛-nitro group occurs when 42 is reduced directly, but this problem was circumvented by using electrogenerated superoxide ion to deprotonate 42 (Scheme 6). (This is readily done: one simply exposes the electrolysis solution to the atmosphere during electrolysis; oxygen diffuses into the solvent and is immediately reduced to superoxide). The anion 43 is alkylated in high yield if the electrolysis is carried out in acetonitrile containing a tetraalkylammonium salt as supporting electrolyte; under these conditions ion-pairing is minimized and the carbanion is highly reactive. One can even effect dialkylation of 42 under these conditions. Anion 43 also reacts readily with Michael acceptors to afford the corresponding adducts in good yields. It is also possible to carry out alkylation and Michael addition in a single pot. Double Michael addition can be carried out also to produce adducts of type 44. Since these are tertiary nitro compounds, they are readily reductively denitrated (equation 16)58. The nitro group is used temporarily to effect formation of the two carbon carbon bonds, and is then removed.
R2CHNO2 C e |
! R2C NO2 C 1/2 H2 |
|
|
|
(15) |
|||||
|
|
|
|
O |
|
|
|
O |
||
|
− |
|
X |
2 e |
− |
|
X |
|||
(42) |
e , O2 |
|
EtO |
|
|
|
EtO |
|
||
X |
NO2 |
H+ |
H |
|||||||
|
|
|
||||||||
|
|
|
|
|
|
|
|
|||
|
|
|
|
X |
|
|
|
X |
(44) |
(16) |
|
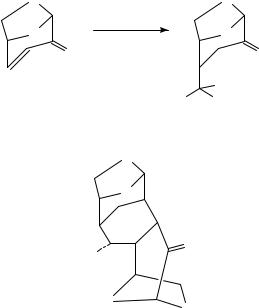
852 |
|
|
|
Albert J. Fry |
O |
C |
e |
! |
O ž |
2 |
|
2 |
||
O2 |
ž C O2NCH2CO2Et ! O2NCH CO2Et |
|||
|
|
|
(42) |
(43) |
43 C ‘E’ (electrophile) ! O2NCH(E)CO2Et SCHEME 6
Secondary nitro compounds can be converted into carbanions in similar fashion. Interesting highly functionalized adducts (46) were prepared by addition to levoglucosene (45) (equation 17)59. Mixtures of diastereomeric adducts were generally formed60. The adduct from nitromethane undergoes double Michael addition followed by aldol condensation to afford the novel adduct 47.
O |
O |
|
e− |
O |
|
O |
|
|
RCH(R′ )NO2 |
O |
|
O |
(17) |
|
|
|
|
|
NO2 |
|
R |
R′ |
|
(45) |
(46) |
|
O
O OH
O
O2 N
O
O
(47)
B. Nitro Compounds as Protecting Groups
The extreme ease of electrochemical reduction of nitro compounds suggests that they might usefully serve as protecting groups. Torii has shown that alcohols can be protected as their p-nitrobenzyl ethers. The group is removed by a two-step sequence involving catalytic reduction to the corresponding amine, followed by electrochemical oxidation of the amine, which is accompanied by hydrolysis of the intermediate Schiff base61.
The electrochemical behavior of a series of nitrobenzenesulfonamides (48 and 49) were examined by cyclic voltammetry and other techniques in connection with the use of such arenesulfonyl groups as protecting groups for amines. Interestingly, the behavior of the 2-nitro derivative 48a and the N,N-dialkyl 4-nitro derivative 49 differed from that of the 3-nitro and 4-nitro monosulfonamides 48b and 48c62. Ortho-derivative 48a and 49
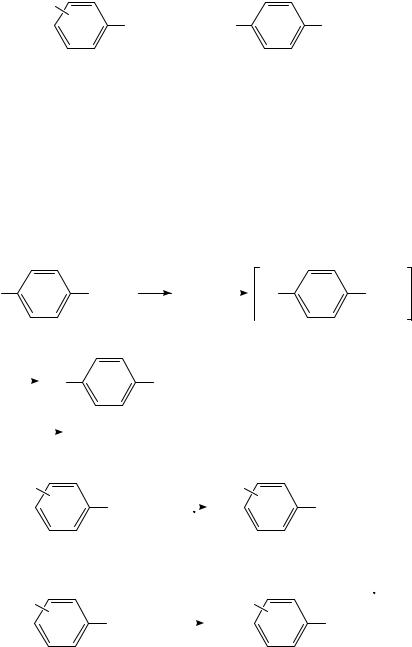
18. The electrochemistry of nitro, nitroso, and related compounds |
853 |
||
O2 N |
|
|
|
SO2 NHBu |
O2 N |
SO2 NBu2 |
|
(48a) X = 2−NO2 |
|
(49) |
|
(48b) X = 3−NO2 |
|
|
|
(48c) X = 4−NO2 |
|
|
|
are reduced to radical anions which are rather stable; they are however reduced at more negative potentials to dianions, which readily fragment with cleavage of the S N bond and ejection of the nitrobenzenesulfinate anion (this is exemplified for 49 in Scheme 7). The free amines are isolated in good yields (>70%). The initial radical anions from 48b and 48c fragment relatively readily with loss of a hydrogen atom to form a species which can be reduced further to a rather stable dianion (Scheme 8). Since the latter species does not undergo spontaneous cleavage of the S N bond at a useful rate, electrochemistry is therefore not useful for removal of the arenesulfonyl group with compounds such as 48b and 48c. The question naturally arises why different behavior should be exhibited by
−2
|
|
|
|
|
|
|
e− |
radical e |
− |
|
|
|
||||||||||
O2 N |
(49) |
SO2 NBu2 |
anion |
|
|
|
|
O2 N |
(50) |
SO2 NBu2 |
||||||||||||
|
|
|
||||||||||||||||||||
|
|
|
|
|
|
|
|
|
|
|
|
|
|
|
|
|
|
|||||
|
|
|
|
|
|
|
|
|
|
|
|
|
|
|
|
|
|
|||||
(50) |
|
|
|
|
O2 N |
SO2 |
− + −NBu2 |
|
|
|
|
|
|
|
|
|||||||
|
|
|
|
|
|
|
|
|
|
|
|
|||||||||||
− |
|
H |
+ |
|
|
|
|
|
|
|
|
|
|
|
|
|
|
|
|
|
||
NBu2 |
|
|
|
|
HNBu2 |
|
|
|
SCHEME 7 |
|
|
|
|
|
|
|
|
|||||
|
|
|
|
|
|
|
|
|
|
|
|
|
|
|
||||||||
|
|
|
|
|
|
|
|
|
|
|
|
|
|
|
|
|
|
|||||
|
|
O2 N |
|
|
|
|
|
|
|
|
|
O2 N |
− |
|||||||||
|
|
|
|
|
|
|
|
|
|
1. e |
− |
|
|
|
|
|
||||||
|
|
|
|
|
|
|
SO2 NHBu |
|
|
|
|
|
|
|
|
|
|
SO2 NBu |
||||
|
|
|
|
|
|
|
|
2. −H |
|
|
|
|
|
|||||||||
|
|
|
|
|
|
|
|
|
|
|
|
|
|
|
|
|
|
|||||
|
|
(48b) X =3−NO2 |
|
|
|
|
|
|
|
|
|
|
|
|
|
|
|
|
||||
|
|
(48c) X =4−NO2 |
|
|
|
|
|
|
|
|
|
|
|
|
|
|
|
|
||||
|
|
O2 N |
|
|
− |
e− |
|
O2 N |
|
|
−2 |
|||||||||||
|
|
|
|
|
|
|
|
|||||||||||||||
|
|
|
|
|
|
|
|
|
|
|
|
|
|
|
||||||||
|
|
|
|
|
|
|
SO2 NBu |
|
|
|
|
|
|
|
|
|
|
|
SO2 NBu |
|
||
|
|
|
|
|
|
|
|
|
|
|
|
|
|
|
|
|
|
|||||
|
|
|
|
|
|
|
|
|
|
|
|
|
|
|
|
|
|
|
|
(51) |
|
|
|
|
|
|
|
|
|
|
|
|
|
|
|
|
|
|
|
|
|
|
|
|
|
|
|
|
|
|
|
|
|
|
|
SCHEME 8 |
|
|
|
|
|
|
|
|

854 |
Albert J. Fry |
compounds as similar as 48a and, say, 48b. In effect, the question is: why does dianion 50 fragment while dianion 51 does not? The difference between the two is quite possibly the fact that dianion 50 has two electrons in an antibonding pi-orbital, whereas 51, which is a dianion radical, has only one electron in an antibonding pi-orbital. Furthermore, 51 can accommodate part of its negative charge on the relatively nonbasic sulfonamide nitrogen atom, while 50 has a doubly negative charge in its pi-system; these effects provide for a powerful driving force for cleavage of 50 which is absent from 51.
On occasion such reductive deprotection processes can be quite selective. Electrochemical reduction of N,N0 -di-p-toluenesulfonyl-N-t-butoxycarbonyl derivatives of aliphatic and aromatic diamines selectively removed the p-toluenesulfonyl group attached to a primary amine site63. Yields on the mono-protected products are fair to high; selective deprotection of the corresponding N,N0 -dibenzoyl derivatives occurred in yields 92%.
VII. NITROSO COMPOUNDS
As can be seen from the preceding discussion, the existence of nitroso compounds as intermediates in the electrochemical reduction of nitro compounds is mostly inferential: nitroso compounds are easier to reduce than nitro compounds. Hence, they should be reduced as quickly as they are formed and would not be expected to be isolable. However, nitroso compounds have occasionally been isolated in unusual structural cases54 and the nitrosobenzene radical anion has been identified by ESR spectroscopy in at least one instance64. It is possible to prepare nitroso compounds by a two-step sequence: one reduces the nitro compound electrochemically to the hydroxylamine, then electrochemically oxidizes the hydroxylamine to the nitroso compound65.
It has been suggested on thermochemical grounds that the radical cations of at least some nitroso compounds should be short-lived species. Such calculations suggest in fact that the C N bond dissociation energy of the radical cation of 2-nitroso-2-methylpropane should be close to zero66. Direct electrochemical oxidation and reduction afford radical cations and radical anions, respectively, the ESR spectra of which have been characterized in a number of cases67. Voltammetric studies have shown that the cationic micelle-forming surfactant cetyltrimethylammonium bromide inhibits the reduction of p- nitroso-N,N-dimethylaniline to the corresponding arylhydroxylamine68.
One possibility, the ECEC path, i.e. alternating electron transfer and protonation steps, for the mechanism of reduction of nitrosobenzene to phenylhydroxylamine was discussed in Section II.A.1. It is pointed out there that this conversion might take place by a number of different paths. Laviron explored this question69. He found that the mechanism is CECE in acidic media and ECEC in basic media and ECCE at intermediate pH.
VIII. ACKNOWLEDGMENTS
Professors Dennis Evans and Petr Zuman kindly provided material in advance of publication. Financial support was provided by the National Science Foundation (Grant #CHE9413128).
IX. REFERENCES
1.A. J. Fry, in The Chemistry of Functional Groups, Supplement F: The Chemistry of Amino, Nitroso and Nitro compounds (Ed. S. Patai), Wiley, Chichester, 1982, pp. 319 337.
2.A. J. Fry, ‘Solvents and Supporting Electrolytes’, in Laboratory Techniques in Electroanalytical Chemistry, 2nd ed. (Eds. P. T. Kissinger and W. R. Heineman), Marcel Dekker, New York, in press.
3.F. Haber, Z. Elektrochem., 4, 506 (1989).

18. The electrochemistry of nitro, nitroso, and related compounds |
855 |
4.(a) P. Zuman, in Electroorganic Synthesis (Eds. R. D. Little and N. L. Weinberg), Marcel Dekker, New York, 1991, p. 137.
(b)P. Zuman, Z. Fijalek, D. Dumanovic and D. Suznjevic, Electroanalysis, 4, 783 (1992).
5.W. M. Smith and A. J. Bard, J. Am. Chem. Soc., 97, 5203 (1975).
6.B. S. Jensen and V. D. Parker, J. Chem. Soc., Chem. Commun., 367 (1974).
7.(a) A. Darchen and C. Moinet, J. Electroanal. Chem., 68, 173 (1976).
(b)F. D. Popp and H. P. Schultz, Chem. Rev., 62, 19 (1962).
8.(a) J. C. Ruhl,¨ D. H. Evans, P. Hapiot and P. Neta, J. Am. Chem. Soc., 113, 5188 (1991).
(b)J. C. Ruhl,¨ D. H. Evans and P. Neta, J. Electroanal. Chem., 340, 257 (1992).
(c)H. Wang and O. Hammerich, Acta Chem. Scand., 46, 563 (1992).
9.C. M. Amatore and J. M. Saveant,´ J. Electroanal. Chem., 86, 227 (1978).
10.(a) B. Kastening, Electrochim. Acta, 9, 241 (1964).
(b)B. Kastening, Coll. Czech. Chem. Commun., 30, 4033 (1965).
(c)B. Kastening, Z. Anal. Chem., 224, 196 (1967).
(d)B. Kastening, Ber. Bunsenges. Phys. Chem., 72, 27 (1968).
11.L. Meites, Polarographic Techniques, 2nd ed., Wiley-Interscience, New York, 1965, p. 176.
12.(a) H. Lund, in Organic Electrochemistry (Eds. H. Lund and M. M. Baizer), 3rd ed., Marcel Dekker, New York, 1991, pp. 401 432
(b)W. Kemula and T. M. Krygowski, in Encyclopedia of Electrochemistry of the Elements (Eds. A. J. Bard and H. Lund), Vol. 13; Chap. 2, Marcel Dekker, New York, 1979.
(c)B. Kastening, in Progress in Polarography (Eds. P. Zuman and L. Meites), Vol. 3, WileyInterscience, New York, 1972, pp. 259 267.
13.A. J. Fry, R. D. Little and J. Leonetti, J. Org. Chem., 59, 5017 (1944).
14.E. Laviron, J. Electroanal. Chem., 124, 9 (1981).
15.E. Laviron, J. Electroanal. Chem., 146, 1 (1981)
16.E. Laviron and L. Roullier, J. Electroanal. Chem., 288, 165 (1990).
17.E. Laviron, R. Meunier-Prest and R. Lacasse, J. Electroanal. Chem., 375, 263 (1994).
18.A. Darchen and C. Moinet, J. Electroanal. Chem., 78, 81 (1977).
19.A. Darchen and C. Moinet, J. Chem. Soc., Chem. Commun., 487 (1976).
20.(a) C. D. Gutsche and D. J. Pasto, Fundamentals of Organic Chemistry, Prentice-Hall, Englewood Cliffs, N.J., 1975, p. 714.
(b)G. M. Loudon, Organic Chemistry, Addison-Wesley, Reading, Mass., 1984, p. 1198.
21.T. Ohba, H. Ishida, T. Yamaguchi, T. Horiuchi and K. Ohkubo, J. Chem. Soc., Chem. Commun., 263 (1994).
22.D. H. Geske and A. H. Maki, J. Am. Chem. Soc., 82, 2671 (1960).
23.G. L. McIntire, D. M. Chiappardi, R. L. Casselberry and H. N. Blount, J. Phys. Chem., 86, 2632 (1982).
24.C. -W. Lee and A. J. Fry, unpublished research.
25.(a) J. F. Rusling, Acc. Chem. Res., 24, 75 (1991)
(b)M. Bourrel and R. S. Schecter, Microemulsions and Related Systems, Marcel Dekker, New York, 1988.
(c)I. D. Robb (Ed.), Microemulsions, Plenum Press, New York, 1982.
26.G. Kokkinidis, A. Papoutsis and G. Papanastasiou, J. Electroanal. Chem., 359, 253 (1993).
27.A. Papoutsis and G. Kokkinidis, J. Electroanal. Chem., 371, 231 (1994).
28.S. J. C. Cleghorn and D. Pletcher, Electrochim. Acta, 38, 425 (1993).
29.G. Belot, S. Desjardins and J. Lessard, Tetrahedron Lett., 25, 5347 (1984).
30. P. Delair, A. Cyr and J. Lessard, in Electroorganic Synthesis (Eds: R. D. Little and
N. L. Weinberg), Marcel Dekker, New York, 1991, p. 129.
31.P. N. Pintauro and J. R. Boutha, J. Appl. Electrochem., 21, 799 (1991).
32.(a) C. Ravichandran, M. Noel and P. N. Anantharaman, J. Appl. Electrochem., 24, 965 (1994).
(b) C. Ravichandran, D. Vasudevan and P. N. Anantharaman, J. Appl. Electrochem., 22, 1192 (1992).
33.E. Laviron, R. Meunier-Prest, A. Vallat, L. Roullier and R. Lacasse, J. Electroanal. Chem., 341, 227 (1992).
34.R. Lacasse and R. Meunier-Prest, J. Electroanal. Chem., 359, 223 (1993).
´
35. C. P. Andrieux, A. Batlle, M. Espin, I. Gallardo, Z. Jiang and J. Marquet, Tetrahedron, 50, 6913 (1994).
36. (a) K. J. Stutts, C. L. Scortichini and C. M. Repucci, J. Org. Chem., 54, 3740 (1989).
856 |
Albert J. Fry |
(b)M. Ciureanu, Rev. Roum. Chim., 38, 807 (1993).
(c)M. M. Ellaithy and P. Zuman, J. Pharm. Sci., 81, 191 (1992).
37.D. Vasudevan and P. N. Anantharaman, J. Appl. Electrochem., 24, 559 (1994).
38.A. Ion-Carastoian, F. -G. Banica and M. Moraru, Rev. Roum. Chim., 38, 287 (1993).
39.A. H. Maki and D. H. Geske, J. Chem. Phys., 33, 825 (1960).
40.The negative charge in nitroarene radical anions is localized on the nitro group:
(a)W. Kemula and R. Sioda, J. Electroanal. Chem., 7, 233 (1964).
(b)A. H. Maki D. H. Geske, J. Am. Chem. Soc., 83, 1853 (1961).
41.(a) Y. Kargin, O. Manousek and P. Zuman, J. Electroanal. Chem., 12, 443 (1966).
(b)P. Zuman, O. Manousek and S. K. Vig, J. Electroanal. Chem., 19, 147 (1968).
42.H. Wang and V. D. Parker, Acta Chem. Scand., 48, 933 (1994).
43.S. Irle, T. M. Krygowski, J. E. Niu and W. H. E. Schwarz, J. Org. Chem., 60, 6744 (1995).
44.S. Mahmood, B. J. Tabner and V. A. Tabner, J. Chem. Soc., Faraday Trans., 3253 (1990).
45.T. Yamato, K. Fujita, H. Kamimura, M. Tashiro, A. J. Fry, J. Simon and J. Ochterski, Tetrahedron, 51, 9851 (1995).
46.Potentials were measured relative to 0.1 M Ag/AgNO3 and converted to S.C.E.
47.Orbital overlap is proportional to cos2 , where is the dihedral angle between the two orbitals.
Since D 16.1° in 25, cos2 D 0.92, hence little overlap is lost by the twist of the nitro groups out of planarity.
48.F. Miralles-Roch, A. Tallec and R. Tardivel, Electrochim. Acta, 38, 2379 (1993).
49.M. Wessling and H. J. Schafer,¨ Chem. Ber., 124, 2303 (1991).
50. R. D. Little, M. Schwabe and W. Russu, in Novel Trends in Electro-organic Synthesis (Ed.
S. Torii), Kodansha, Tokyo, 1995, p. 123.
51.A. J. Fry, Synthetic Organic Electrochemistry, 2nd ed., Wiley, New York, 1989, p. 308.
52. (a) D. H. Evans and J. C. Ruhl,¨ in Electroorganic Synthesis (Eds. R. D. Little and N. L. Weinberg), Marcel Dekker, New York, 1991, p. 3.
(b) W. J. Bowyer and D. H. Evans, J. Org. Chem., 53, 5234 (1988).
53.A. M. Bond, G. A. Lawrance, P. A. Lay and A. M. Sargeson, Inorg. Chem., 22, 2010 (1983).
54.P. A. Lay and A. M. Sargeson, Inorg. Chem., 29, 2762 (1990).
55.L. Szabo, C. Szantay, E. G. Baitz and M. Mak, Tetrahedron Lett., 36, 5265 (1995).
56.M. E. Niyazymbetov and D. H. Evans, Tetrahedron, 49, 9627 (1993).
57.M. E. Niyazymbetov and D. H. Evans, J. Org. Chem., 58, 779 (1993).
58.M. E. Niyazymbetov and D. H. Evans, Denki Kagaku, 62, 1139 (1994).
59.A. L. Laikhter, M. E. Niyazymbetov, D. H. Evans, A. V. Samet and V. V. Semenov, Tetrahedron Lett., 34, 4465 (1993).
60.Private communication from D. H. Evans and M. E. Niyazymbetov.
61.K. Fukase, H. Tanaka, S. Torii and S. Kusumoto, Tetrahedron Lett., 31, 389 (1990).
62.M. V. B. Zanoni and N. R. Stradiotto, J. Electroanal. Chem., 312, 141 (1991).
63.L. Grehn, L. S. Maia, L. S. Monteiro, M. I. Montenegro and U. Ragnarsson, J. Chem. Res. (S), 144 (1991).
64.C. Nishihara and M. Kaise, J. Electroanal. Chem., 149, 287 (1983).
65.(a) P. Zuman and C. Karakus, Denki Kagaku, 62, 1306 (1994); P. Zuman and B. Shah, Chem. Rev., 94, 1621 (1994).
66.M. L. Greer, H. Sarker, M. E. Mendicino and S. C. Blackstock, J. Am. Chem. Soc., 117, 10460 (1995).
67.G. Gronchi and P. Tordo, Res. Chem. Intermed., 19, 733 (1993).
68.N. C. Sarada, I. A. K. Reddy and K. M. Rao, J. Indian Chem. Soc., 71, 729 (1994).
69.E. Laviron, A. Vallat and R. Meunier-Prest, J. Electroanal. Chem., 379, 427 (1994).