
920
.pdf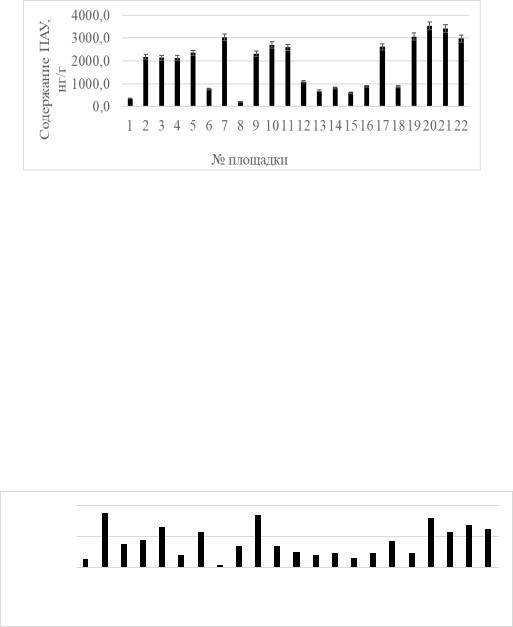
Объект исследования. Мониторинговые площадки заложены по всей площади бывшего шламосборника в количестве 22-х штук. Отбор проб был произведен методом конверта в июне месяце 2018 г. на глубину 0-20 см. Экстракцию ПАУ из почвенных образцов проводили методом омыления. Количественно определяли ПАУ в экстракте методам высоко эфективной жидкостной хроматографии [2]. Повторность трехкратная. В данном исследовании суммарное содержание ПАУ складывалось из отдельных ПАУ таких как: нафталин, бифенил, антрацен, фенантрен, флуорен, бенз(а)антрацен, пирен, флуорантен, БаП, бенз(k)флуорантен, бенз(b)флуорантен, дибенз(a.h)антрацен, бенз(g,h,i)перилен.
Результаты. Суммарное содержание ПАУ в почвах исследуемой территории варьирует в широких пределах: от 207,9 нг/г в почвах площадки №8 до 3536,7 нг/г в почвах площадки № 19 (Рис. 1).
Рисунок 1 Суммарное содержание ПАУ в почвах бывшего шламонакопителя
Следует отметить, что суммарное содержание ПАУ в исследуемой почве не зависит от свойств самой почвы и адекватный эталон сравнения подобрать проблематично. Дело в том, что для Ростовской области фоновым суммарным содержанием ПАУ в почвах принято брать суммарное содержание ПАУ в почве ООПТ «Персиановская степь» - это примерно 300 нг/г, в зоне постоянного техногенного воздействия (в районе НчГРЭС) суммарное содержание в наиболее загрязненной почве доходит до 3500 нг/г [9], однако, во-первых, и в первом и во втором случае речь идет о черноземах, а во-вторых в районе НчГРЭС нагрузка на почву постоянная, а в данном исследовании рассматривается объект, который не подвержен техногенному воздействию уже более 30 лет. Содержание БаП превышено в почвах всех мониторинговых, за исключением почвы площадки № 8. Максимальное превышение ПДК БаП зафиксировано в почвах площадки № 2, где содержание БаП в почве превышено в 8,7 раз (Рис. 2).
ПДК БаП
10,00
5,00
0,00
1 |
2 |
3 |
4 |
5 |
6 |
7 |
8 |
9 |
10 |
11 |
12 |
13 |
14 |
15 |
16 |
17 |
18 |
19 |
20 |
21 |
22 |
№ площадки
Рисуок 2. Уровни ПДК БаП в почвах бывшего шламонакопителя
Согласно СанПиН 2.1.7.1287-03 [3] почва бывшего шламонакопителя характеризуется, как сильнозагрязн нная (Таблица 1).
290
Таблица 1
Оценка степени загрязнения почвы бывшего шламонакопителя
Содержание в почве |
Категория загрязнения почвы |
|
|
Класс опасности вещества |
1 класс |
2 класс |
3 класс |
|
|
|
|
> 5 ПДК |
Очень сильная |
|
Сильная |
От 2 до 5 ПДК |
Очень сильная |
Сильная |
Средняя |
От 1 до 2 ПДК |
Слабая |
|
|
Таким образом, выявлено, что суммарное содержание ПАУ в почвенном покрове бывшего шламонакопителя не равномерно и варьирует в широких пределах. Несмотря на то, что, в течение уже более 30 лет, территория озера Атаманского не используется, как шламосборник, исследование показало высокий уровень суммарного содержания ПАУ в исследованных почвах, что может свидетельствовать о замедлении биохимических и химических процессов, способствующих деструкции ПАУ. Содержание БаП в исследуемых почвах, как главного маркера загрязнения почв ПАУ, свидетельствует о том, что почвы данной территории можно отнести к сильнозагрязненным.
Исследования выполнены при финансовой поддержке российского научного фонда (РНФ) № 19-74- 10046.
Литература
1.ГН 2.1.7.2041-06. Предельно допустимые концентрации (ПДК) химических веществ в почве. М. 2009. 22 с.
2.ПНД Ф 16.1:2.2:2.3:3.62-09 Количественный химический анализ почв //Методика выполнений измерений массовых долей полициклических ароматических углеводородов в почвах, донных отложениях, осадках сточных вод и отходах производства и потребления методом высокоэффективной жидкостной хроматографии. М. 2009. 22 с.
3.СанПиН 2.1.7.1287-03 Санитарно-эпидемиологические требования к качеству почвы" (с изменениями на 25 апреля 2007 года). М. 2007. 34 с.
4.Чаплыгин В.А., Манджиева С.С., Невидомская Д.Г., Литвинов Ю.А., Минкина Т.М., Черникова Н.П. Содержание тяжелых металлов в тростнике обыкновенном (Phragmites australis) техногенно загрязненного района поймы Северского Донца // Биогеохимия химических элементов и соединений в природных средах: материалы III Международной школы-семинара молодых исследователей. 2018. С. 307-311.
5.Цибарт А.С, Геннадиев А.Н. (2013). Полициклические ароматические углеводороды в почвах: источники, поведение, индикационное значение (обзор) // Почвоведение. 2013. С. 788-788.
6.Lamichhane S., Krishna K.B., Sarukkalige R. Polycyclic aromatic hydrocarbons (PAHs) removal by sorption: a review // Chemosphere. 2016. № 148. С. 336-353.
7.Minkina T., Sushkova S., Yadav B.K., Rajput V., Mandzhieva S., Nazarenko, O. Accumulation and transformation of benzo[a]pyrene in Haplic Chernozem under artificial contamination.// Environmental geochemistry and health. 2019. С. 1-10.
8.Office of the Federal Registration (OFR) Appendix A: priority pollutants. Fed Reg. 1982;47:52309. URL: https://www.epa.gov/sites/production/files/2015-09/documents/priority-pollutant-list-epa.pdf.
9.Sushkova S.N., Minkina T.M., Mandzhieva S.S., Vasilyeva G.K., Borisenko N.I., Turina I.G., Kızılkaya
R. New alternative method of benzo[a]pyrene extractionfrom soils and its approbation in soil under technogenic pressure // Journal of soils and sediments. 2016. №16. С. 1323-1329.
S.N. Sushkova, T.S. Dudnikova, E.M. Antonenko,
A.I. Barbashev, I.P. Lobzenko, T.M. Minkina
Southern Federal University, Rostov-on- Don, Russia
ESTIMATION OF THE SOIL POLLUTION DEGREE IN THE TERRITORY
OF CHEMICAL POLLUTION
BY POLYCYCLIC AROMATIC HYDROCARBONS
Abstract. Soils of the former sludge collector were investigated for the total content of polyarenes in them. The uneven nature of the total distribution of PAHs in the soils of the
291
study area was revealed. The excess of the MPC of benzo(a)pyrene was established, characterizing the soils of the study area as highly contaminated.
Keywords: PAHs, polyarenes, benzo(a)pyrene, sludge collector, industrial waste, technogenic soils.
References
1.GN 2.1.7.2041-06. Maximum allowable concentration (MPC) of chemicals in the soil. M. 2009.22 p.
2.PND F 16.1: 2.2: 2.3: 3.62-09 Quantitative chemical analysis of soils // Method for measuring mass fractions of polycyclic aromatic hydrocarbons in soils, bottom sediments, sewage sludges and production and consumption wastes by high performance liquid chromatography. M. 2009.22 p.
3.SanPiN 2.1.7.1287-03 Sanitary and epidemiological requirements for soil quality "(as amended on April 25, 2007). M. 2007. 34 p.
4.Chaplygin V.A., Mandzhieva S.S., Nevidomskaya D.G., Litvinov Yu.A., Minkina T.M., Chernikova N.P. The content of heavy metals in common reed (Phragmites australis) of the technogenic contaminated area of the Seversky Donets floodplain // Biogeochemistry of chemical elements and compounds in natural environments: materials of the III International School-Seminar for Young Researchers. 2018. P. 307-311.
5.Tsibart A.S., Gennadiev A.N. (2013). Polycyclic aromatic hydrocarbons in soils: sources, behavior, indicative value (review) // Soil Science. 2013 S. 788-788.Lamichhane S., Krishna K.B., 6. Sarukkalige R. Polycyclic aromatic hydrocarbons (PAHs) removal by sorption: a review // Chemosphere. 2016. № 148.
P. 336-353.
7.Minkina T., Sushkova S., Yadav B.K., Rajput V., Mandzhieva S., Nazarenko, O. Accumulation and transformation of benzo[a]pyrene in Haplic Chernozem under artificial contamination.// Environmental geochemistry and health. 2019. P. 1-10.
8.Office of the Federal Registration (OFR) Appendix A: priority pollutants. Fed Reg. 1982;47:52309. URL: https://www.epa.gov/sites/production/files/2015-09/documents/priority-pollutant-list-epa.pdf.
9.Sushkova S.N., Minkina T.M., Mandzhieva S.S., Vasilyeva G.K., Borisenko N.I., Turina I.G., Kızılkaya
R. New alternative method of benzo[a]pyrene extractionfrom soils and its approbation in soil under technogenic pressure // Journal of soils and sediments. 2016. №16. P. 1323-1329.
UDC 550.4
O.B. Rogova3, P.S. Fedotov1,2, R.Kh. Dzhenloda1,2, V.K. Karandyshev1,4 1National University of Science and Technology “MISiS”, Moscow, Russia;
2V.I. Vernadsky Institute of Geochemistry and Analytical Chemistry, Russian Academy of Sciences, Moscow, Russia;
3 V.V. Dokuchaev Soil Science Institute, Moscow, Russia;
4The Institute of Microelectronics Technology and High-Purity Materials, Russian Academy of Sciences, Chernogolovka, Russia.
Email: olga_rogova@inbox.ru
FORMS OF RARE EARTH METALS IN PERM SOILS
Abstract. The distribution of REEs between environmentally relevant (bioaccessible) fractions has been studied by the example of background, aerially and hydrogenically contaminated soil samples using dynamic extraction in a rotating coiled column. In all samples, extractable REEs are almost completely bound to amorphous metal oxide-or- ganic.
Keywords: soil, rare earth elements, fractionation, dynamic extraction, metal-organic complexes
Rare earth elements (REEs) present a cohesive group that includes lanthanides as well as Y and Sc. In soils, REEs originate mainly from the parent material and the use of organic and inorganic fertilizers. For example, Australian phosphate fertilizers contain an average of 45.2 µg/g La and 61.0 µg/g Ce. The median value of La concentration in European soils was reported to be 14.3 and 13.6 µg/g with maximum values of 109.3 and 229.6 µg/g in agricultural and grazing land soils, respectively. Apart from the agriculture,
292
REEs are also increasingly used in high-tech industry and healthcare technologies. The release of REEs into soils and waters and their transfer into plants may have negative impacts on human health and the environment.
Lanthanum toxicity may vary due to changing the environmental conditions. For example, it was demonstrated that deleterious effects of the combined La3+ and acid rain pollution on soybean (Glycine max) were stronger than those of single La3+ or acid rain pollution. Lanthanum toxicity to five different species of soil invertebrates in relation to availability in soil has been also assessed. It is suggested that La may affect soil ecosystems at concentrations slightly above natural background levels (6.6–50 mg La/kg dry soil) in non-polluted soils.
A few attempts have been made to estimate the transfer of REEs from natural soil to plant systems in the basis of the correlation between the total concentrations of REEs in soils and their contents in plant roots. It has been found that for soils from non-polluted natural sites with different geological environments (limestone, granite, and carbonatite), the uptake of REEs by plants is not primarily controlled by the plant itself, but depends on the concentration and speciation of REE in the soil and adsorbed soil water pool. Plants growing on moist grassland contained higher concentrations of REEs as compared to other soils The content of organic matter and low pH may enhance the phytouptake of REEs. REEs uptake by plant roots is considered to be linked with those of Fe, and roots absorb preferentially light REE (La, Ce, Pr, Nd, Pm, Sm, Eu).
For the fractionation of REEs in soils, sequential extraction procedures (SEP) initially developed for the fractionation of heavy metals have been mainly applied. Most studies adopt one of two extensively used SEP, namely, the four-step SEP proposed by Tessier et al. (1979) or the three-step BCR procedure developed in the framework of the Standards, Measurements, and Testing Programme of the European Commission. For example, the BCR procedure has been used to study the fractionation of REEs in the different horizons of two soil profiles (Eutric Fluvisols) at the Wupper River, Germany. Small differences in the total REEs concentrations between the horizons were observed, probably, due to flooding and the linked homogenization processes. REEs were found to dominate in the residual fraction (73.5%), followed by reducible (19.6%), oxidisable (6.6%), and exchangeable (0.4%) fraction. In general, for a large set of soil samples from different regions of Germany, the residual fraction is relatively high. In soils, where water fluctuations are important, the redox potential may be regarded as a key factor controlling the mobilization of REEs also via related changes of pH.
The aim of the present work is to apply the dynamic extraction in RCC to study on the fractionation and mobility of REEs in background floodplain (alluvial) soil as well in soddy-podzolic and floodplain soils aerially and hydrogenically contaminated by heavy metals. Environmentally relevant (bioaccessible) fractions, namely, exchangeable, specifically sorbed, bound to Mn oxides, and bound to metal-organic complexes are separated and analyzed.
All the samples were 5 sub-samples of surface layer (0-10 cm) collected in the city of Perm’ (Russia). The selected soils are characterized by different contents of major components governing the behaviour of micro elements as well as by different levels of anthropogenic impact. Sample 1, alluvial (floodplain) soil taken in the floodplain area of the river Kama opposite to the city of Perm’, was chosen as a sample with background levels of trace elements. Sample 2, soddy-podzolic soil was taken in a small park area at
293
the distance of 0.5 km from an engine building plant. According to the analysis of the snow cover Sample 2 was chosen as a soil subjected to the aerial contamination. Sample 3, alluvial anthropogenic transformed soil, was collected in the floodplain of the river Danilikha. Highly elevated concentrations of Zn, Cu, Cd, Pb, and Ni found in the silt of river Danilikha confirms the hydrogenic contamination of this soil. In all the cases, upper horizons of soils (0–10 cm) were under investigation. The anthropogenic contamination of Samples 2 and 3 has been reported earlier.
It has been found that the total contents of REEs in alluvial soils (samples 1 and 3) are 2-3 fold higher, than in the soddy-podzolic soil (sample 2). At the same time the contents of REEs in the floodplain of the river Kama (sample 1) is 1.5 fold higher, than in the floodplain of the river Danilikha (sample 3). This may be attributed not only to the difference in the contents of organic carbon in soils but as well as to the accumulation of REEs by Fe-Mn-organic concretions which are abundant in alluvial soils as a result of redox processes. This assumption is proven by the Ce/La ratio, which reflects the role of redox processes and accumulation of organic matter in the formation and development of soil. The value of CCe/CLa equal to 2.7 is maximal for sample 1 (floodplain soil on the river Kama), which also contains the maximal quantities of organic matter and manganese. For samples 2 and 3, CCe/CLa is equal to 2,1 and 2.0, correspondingly, which is apparently due to lower contents of organic matter as compared to sample 1. Hence, the accumulation of REEs is the floodplain soil of the river Danilikha can be mainly governed by the formation of Fe-Mn ortsteins, whereas in the soddy-podzolic soil, the accumulation of REEs might be related to the accumulation of organic matter.
The dynamic fractionation of REEs and other trace elements was performed according to the SEP proposed and tested in our previous works. It should be stressed that we focus on environmentally relevant (bioaccessible) fractions. Other fractions such as elements bound to Al/Fe oxides and refractory organic compounds can be considered as poorly mobilizable/accessible and hence have not been separated. We prefer to use the term “bioaccessibility” in our research since bioavailability can be measured only by biological methods (in vivo). In contrary, bioaccessibility can be assessed by in vitro biomimetric methods such as chemical extraction and fractionation. There are slight differences in the definitions given for bioavailability and bioaccessibility; however, they reflect similar characteristic features of bioavailability as actual availability and bioaccessibility as potential availability.
The extractograms of REEs for studies soil samples show sharp high peaks of all REEs appear at step IV (pyrophosphate extraction) for all samples. In general, the sharper the peak of element on the extractogram, the faster the kinetics of mobilisation of the corresponding fraction and the recovery. Hence, organic complexes of REEs (e.g. humates and fulvates) are kinetically labile. In the background floodplain soil (sample 1), an insignificant portion of REEs are bound to Mn oxides (step III). For the anthropogenic transformed floodplain soil (sample 3) only La is partially recovered in this fraction. For the aerially contaminated soddy-podzolic soil (sample 2), exchangeable and acid soluble fraction are observed as well, nevertheless, the association of REEs with organic complexes is apparently predominant.
294
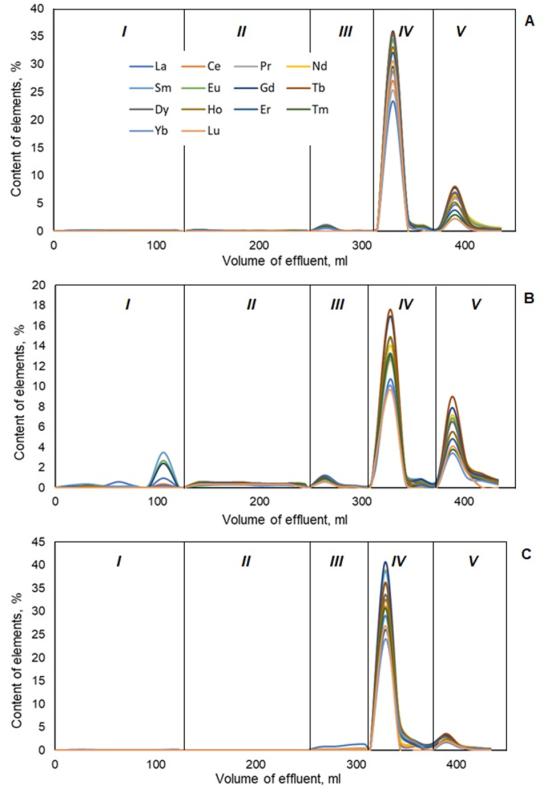
Figure 1. Extractograms of rare earth elements for studied soil samples
(A – sample 1, B – sample 2, C – sample 3). Eluent composition: I – 0.05 М Ca(NO3)2; II - 0.43 М CH3COOH; III – 0.1 M NH2OH•HCl (non acidified); IV – 0.1 M K4P2O7 (pH 11); V - 0.1 M (NH4)2C2O4 (pH 3).
The distribution of REEs between four extractable fractions is quite unexpected; however, the distribution patterns look similar for all studied samples regardless of the
295
soil characteristics and contamination. In all cases REEs are predominantly associated with organic complexes. For floodplain soils (samples 1 and 3) up to 40-45% of the total contents of REEs are found in the pyrophosphate extractable fraction. The contents of organic carbon (13 and 7.8% for samples 1 and 3, correspondingly) and contamination obviously do not affect the distribution of REEs. Other extractable fractions may be taken into consideration only in the case of La. For the soddy-podzolic soil (sample 2, Corg = 4.7%), the distribution of REEs is somewhat different. The contents of REEs in the pyrophosphate extractable fraction are about 20%. Exchangeable, acid soluble, and easily reducible fractions also may be taken into consideration; their sum being up to 8%. Nevertheless, the main trend is the same, namely, extractable REEs are predominantly associated with organic complexes (e.g. humates and fulvates) recovered by pyrophosphate.
At the first glance, there might be a contradiction between the findings made and the reported results on the fractionation of REEs. According the fractionation using the three-step BCR protocol, REEs were found to dominate in the residual fraction (73.5%), followed by reducible (19.6%), oxidizable (6.6%), and exchangeable (0.4%) fraction. However, there are two distinguishing features in the present and cited works, namely, batchwise and dynamic extraction conditions and quite different SEPs. Hence, the results are hardly comparable. It looks more beneficial to refer to the SEP recently proposed for the fractionation of REEs. This SEP includes four steps: 0.05 M calcium nitrate (easily soluble and ion exchange fraction), 0.1 M citric acid (fraction mobilized by complexation and carbonate bound), 0.05 M hydroxylamine hydrochloride (pH 2) (reducible fraction), 1.4 nitric acid (acid soluble fraction). According to the data obtained for a soil profile, the upper horizon may contain up to 30% of the fraction extractable by citric acid. In turn, this fraction may contain REEs recovered from organic complexes due to the competitive binding with citrate, which is a strong complexing reagent. In future it might be beneficial to apply the SEP proposed by Mittermüller et al (2016) to the dynamic fractionation of
REEs in soils.
In general, the finding made in the present work, namely, the predominant association of REEs and metal oxide-organic complexes in soils, is of great importance since it may indicate the high bioaccessibility of REEs related, in particular, to the digestion of humates and fulvates by rhizosphere microorganisms and subsequent release of REEs.
To the best of our knowledge, the predominant association of REEs and metal ox- ide-organic complexes in soils regardless the sample type and contamination is reported for the first time. This finding may offer a novel insight into the fate and behavior of REEs in soil-plant systems. The results should be extended to a variety of soil samples of different origin. Besides, it may be beneficial to apply another SEP under dynamic conditions and to compare the data obtained.
For the risk assessment, it would extremely important to study processes of adaptation of REEs entering the soil with various carriers such as phosphorus fertilizers, coal and phosphate dust, industrial wastes, etc. There are a lot of related questions. How can REEs fix in soils when entering with easily soluble salts or fertilizers? How does the fractionation and mobility of REEs change at their migration within the soil profile? What is the role of various soil components (for example, organometallic concretions) in the accumulation of REEs in soils? The response to all these questions can be given with the use of the proposed continuous-flow fractionation system, which allows one to improve the selectivity of the separation of the investigated groups of compounds.
The equipment was purchased and maintained with the support of the Ministry of Education and Science of the Russian Federation (Program of Increasing Competitiveness of NUST “MISiS”, projects No К1-2014-026, No К2-2017-088).
296
О.Б.Рогова3, П.С. Федотов 1,2, Р.Х. Дженлода 1,2, В.К. Карандашев 1,4
1Национальный исследовательский технологический университет «МИСиС»;
2Институт геохимии и аналитической химии имени В. И. Вернадского РАН;
3ФГБНУ Почвенный ин-т им. В.В.Докучаева;
4ФГБУН Институт проблем технологии микроэлектроники и особо чистых материалов РАН
Email: olga_rogova@inbox.ru
ФОРМЫ СОЕДИНЕНИЙ РЕДКОЗЕМЕЛЬНЫХ ЭЛЕМЕНТОВ В ПОЧВАХ ПЕРМИ
Распределение РЗЭ между экологически значимыми (биодоступными) фракциями было изучено на примере образцов фоновых, аэрально и гидрогенно-загрязненных почв с использованием метода динамического фракционирования во вращающейся спиральной колонне. Во всех образцах экстрагируемые РЗЭ практически полностью связаны с металло-органическими соединениями.
Ключевые слова: почва, редкоземельные элементы, фракционирование, динамическое извлечение, металлоорганические комплексы.
УДК 631.41
С.Н. Сушкова, В.А. Чаплыгин, Т.В. Бауэр, Т.М. Минкина, А.Г. Федоренко Южный федеральный университет, Ростов-на-Дону, Россия
e-mail: terra_rossa@mail.ru
ФОНОВОЕ СОДЕРЖАНИЕ И СОСТАВ СОЕДИНЕНИЙ МЕДИ В ЧЕРНОЗЕМЕ ОБЫКНОВЕННОМ РОСТОВСКОЙ ОБЛАСТИ
Аннотация. Установлено, что Cu в черноземе обыкновенном аккумулируется преимущественно в составе глинистых минералов, что обуславливает ее низкую подвижность в почве. Непрочно связанные соединения элемента в основном представлены специфически сорбированными формами. Фракционный состав Cu показал ее тесную связь с органическим веществом почвы.
Ключевые слова: медь, чернозем, почвенные компоненты, состав соединений, подвижность.
Ростовская область играет колоссальную роль в обеспечении продовольственной безопасности России. Здесь расположены и занимают наибольшую территорию самые плодородные почвы мира – черноземы. Их принято считать почвами оптимального микроэлементного состава, своего рода эталонами. Однако изза высокой карбонатности в природных условиях отмечается недостаток многих микроэлементов, в частности меди [10]. Содержание элементов и их подвижность в почве определяют условия питания растений.
Из большого спектра поллютантов медь заслуживает особого внимания. Она относится к группе приоритетных загрязняющих веществ окружающей среды и является элементом 2-го класса опасности. Повышенные концентрации меди в почвах сельскохозяйственных угодий могут образовываться в зонах воздействия промышленных предприятий. В тоже время при низких концентрациях медь играет важную роль в обменных процессах и жизненно необходима для организмов в качестве микроэлемента. Однако при высоких концентрациях она обладает высокой
297
токсичностью, мутагенным и канцерогенным действиями на живые организмы, способна к биоаккумуляции [7].
Определение состава соединений меди в почвах с учетом ее региональных особенностей необходимо для прогнозирования и оценки обеспеченности данным элементом растений, разработки систем применения удобрений с целью оптимизации питания растений, экологического мониторинга.
Целью данной работы является изучение содержания и состава соединенийCuв черноземе обыкновенном естественных ландшафтов Ростовской области.
Для проведения исследований отбирался верхний слой (0-20 см) почвы целинного участка, представленный черноземом обыкновенным тяжелосуглинистым на лессовидных суглинках учебно-опытного хозяйства «Донское» ДонГАУ (Ростовская обл., Октябрьский р-н). Исследуемая почва характеризуется следующими физическими и химическими свойствами: Cорг –3,7%; pH – 7,3; ЕКО – 37,1 смоль (экв)/кг; обменные катионы (смоль (экв)/кг): Са2+ –31,0, Mg2+ – 4,5; СаСО3 – 0,1%; физическая глина – 53,1%, ил – 32,4%.
Физико-химический анализ состава почвы проводили общепринятыми методами: рН водной вытяжки – потенциометрическим в суспензиях при соотношении почва : вода = 1 : 2,5; содержание органического вещества титриметрическим методом (бихроматное окисление по И.В. Тюрину) [4]; емкость катионного обмена (ЕКО) и обменные катионы Са2+ и Mg2+ – по методу Шаймухаметова [8]; содержание карбонатов комплексонометрическим методом по С.А. Кудрину; гранулометрический состав почвы методом пипетки с пирофосфатной подготовкой пробы [2].Валовое содержание Cu в почве определяли рентгенфлуоресцентным методом с использованием спек-
троскана «MAKC-GV».
Подвижные формы Cu были определены тремя параллельными вытяжками, характеризующие непрочно связанные (НС) соединения ТМ в почве [11]: 1 н. ацетатноаммонийный буфер (ААБ) с pH 4,8, извлекающий обменные формы ТМ; 1% ЭДТА в ААБ с pH 4,8, извлекающий обменные и комплексные формы и 1 н. HCl, извлекающий кислоторастворимые формы. По разнице между содержанием металла в вытяжке смешанного реагента и ААБ определялось количество комплексных соединений. Количество специфически сорбированных соединений находили по разнице между содержанием металла в вытяжке HCl и ААБ. Содержание Cu в составе прочносвязанных (ПС) соединений находили по разности между общим содержанием металла в почве и содержанием НС соединений.
Для выявления роли почвенных компонентов в связывании элемента был использован широко распространенный метод Тессье [14]. Данный метод обеспечивает выделение пяти фракций соединений ТМ: обменной (1MMgCl2), связанной с карбонатами (1MCH3COONa), связанной с (гидр)оксидами Fe и Mn (0,04MNH2OH·HCl в 25% CH3COOH), связанной с органическим веществом (0,02MHNO3+30% H2O2, pH 2, затем
3,2 MCH3COONH4 в 20% HNO3) и остаточной фракции (HF+HClO4, затем HNO3конц.).
Содержание металлов в почвенных вытяжках определено методом атомно-абсорбцион- ной спектрометрии (ААС).
Все анализы выполнены в трехкратной аналитической повторности и статистически обработаны.
Общее содержание Cu в верхнем (0-20 см) слое исследуемой почвы составляет 45 мг/кг, что превышает кларк элемента для почв по А.П. Виноградову [3] более чем в 2 раза (20 мг/кг). Это свидетельствует об относительном обогащении исследуемой почвы Cu. Главной особенностью является низкая подвижность Cu в исследуемой почве (табл. 1), благодаря присутствию высокодисперсных карбонатов и общей высокой буферности черноземов по отношению к металлам [6].На долю
298
непрочно связанных соединенийCu приходится всего 7% от общего содержания металла в почве.В черноземе, по сравнению с другими почвами, существует гораздо больше возможностей для прочного закрепления металлов, чему способствует тяжелый гранулометрический состав, относительно высокая емкость катионного обмена, большее количество гумуса и соединений железа, а также присутствие глинистых минералов монтмориллонитовой группы [5].
Установлена следующая закономерность в распределении Cu в составе НС соединений, мг/кг: специфически сорбированные > комплексные > обменные
(табл. 1).
Таблица 1
Подвижные формы Cu в черноземе обыкновенном Ростовской области
Обменные со- |
Комплексные со- |
Специфически сорбированные |
Непрочно связан- |
единения |
единения |
соединения |
ные соединения |
|
|
мг/кг |
|
0,3±0,01 |
0,5±0,1 |
2,2±0,3 |
3,0±0,6 |
|
% от общего содержания |
|
|
1 |
1 |
5 |
7 |
Количество обменных соединений Cu в исследуемой почве очень низкое и составляет всего 0,3 мг/кг, что не превышает 1% от общего содержания (табл.1). Растения в таких условиях могут испытывать недостаточную обеспеченность этим элементом, что может повлиять на их рост и развитие. Непрочно связанные соединения Cu в основном представлены специфически сорбированными формами (5% от общего содержания), что объясняется присутствием карбонатов, их высокодисперсной мицелярной формой в виде «карбонатной плесени» и слабощелочной реакцией среды [1].
Специфически сорбированные формы представлены в основном (табл.2) соединениями металла с Fe-Mn оксидами (11% от общего содержания).Значительная часть Cu (24%) связана с органическим веществом почвы (табл. 2), чему способствует, помимо электрохимических особенностей самого металла, высокое содержание в черноземе гумуса, в составе которого преобладают гуминовые кислоты (СГК:СФК=1,9) [12]. Вероятно, что с наиболее высокомолекулярными гуминовыми кислотами Cu формирует прочносвязанные соединения, в то время как фульвокислоты наиболее склонны к ионообменной сорбции катионов металла. Органическое вещество является важным регулятором подвижности химических элементов в почвах [9]. Поэтому чернозем может прочно связать большее количество металла, чем другие почвы [5].
Таблица 2
Фракционный состав Cu в черноземе обыкновенном Ростовской области
|
Фракция, свя- |
Фракция, свя- |
Фракция, свя- |
|
|
|
Обменная |
занная с орга- |
Остаточная |
Общее со- |
|||
занная с кар- |
занная с Fe- |
|||||
фракция |
ническим ве- |
фракция |
держание |
|||
бонатами |
Mn оксидами |
|||||
|
ществом |
|
|
|||
|
|
|
|
|
||
0,4 |
1,2 |
5,0 |
11,1 |
27,3 |
45,0 |
|
1 |
3 |
11 |
24 |
61 |
||
|
Примечание. Над чертой – мг/кг, под чертой –% от общего содержания
Главной особенностью фракционного состава соединений Cu в исследуемой почве является значительное преобладание остаточной фракции (61% от общего содержания), характеризующей связь металла с силикатами (табл. 2).Методом тонкой структуры спектра рентгеновского поглощения (EXAFS) установлено, что поглощенные почвой катионы Cu2+ могут замещать часть ионов Al3+ в октаэдрических позициях глинистых минералов [13]. Возможна также адсорбция
299