
3291
.pdf
Issue № 3(31), 2016 |
ISSN 2075-0811 |
UDC 625.717
A. N. Popov1, A. A. Xatuncev2, A. N. Masalykin3
MODELLING A TEMPERATURE MODE
OF A MULTILAYER AIRFIELD SURFACING
Military Educational and Scientific Center of the Air Force
«N. E. Zhukovsky and Y. A. Gagarin Air Force Academy» (Voronezh) Russia, Voronezh, tel.: +7-919-243-32-17, e-mail: popalnik@mail.ru
1PhD in Engineering, Assoc. Prof., Head of the Dept. of Engineering and Airfield Support
2PhD in Engineering, Head of the Laboratory of SRC (Combat Application and Support of the AF)
3Lecturer of the Dept. of General Military Disciplines
Statement of the problem. Absence of a standard technique of designing of strengthening structures, the uniform approach to defining the character of the distribution of temperature fields and estimation of temperature pressure of asphalt concrete strengthening layer complicates a process of coming up with design solutions. In the article the physical and mathematical model of the formation of a temperature field of a multi-layered air field based on which the finite element model was implemented in the software complex COMSOL is considered.
Results. The experimental factorial model for the definition of the law of the fluctuation of a temperature in a plane of contact of layers on the basis of computing active experiments in COMSOL with use of the obtained finite element model is constructed.
Conclusions. The obtained data can be used in the course of calculations to design asphalt concrete layers for strengthening assembled air field pavings from prestressed ferro-concrete plates PAG.
Keywords: airfield pavements, asphalt concrete strengthening layer, temperature effect, finite element method, modeling.
Introduction
Safety of passengers and air freights is one of the country’s top agendas and relies on the technical condition of the overall aircraft infrastructure and artificial pavement in particular. The technical condition of the surfacing and capacity of an airfield pavement is responsible for damage and its dynamics.
© Popov А. N., Khatuntsev А. А., Masalykin А. N., 2016
51

Scientific Herald of the Voronezh State University of Architecture and Civil Engineering. Construction and Architecture
Assembled airfield pavements from ferroconcrete pre-stressed airfield smooth plate are increasingly used and its surface layer has proven to be most vulnerable. Following 8 or 10 years of operation it is in need of a major maintenance. The plate largely remains monolith and the structure of an airfield pavements retains its carrying capacity but performs poorly in terms of flight safety. The performance of assembled airfield pavements is normally improved by replacing deteriorating plates. However, the industrial potential of ferronconcrete plants is not able to meet growing aviation demands.
Alternatively the performance can be improved by strengthening assembled pavements with asphalt concrete. However its low crack resistance and thus reflected cracks in the reinforced layer constrain the use of this material. Degradation of operational and technical condition of an asphalt concrete reinforcement structure is due to a combination of factors such as industrial and technological, mechanical, climatic and operational loads and impacts. Considering all of these is crucial for the durability with minimum operational costs.
The generalization and analysis of the existing calculation methods showed that they do not account for changes in the temperature in the depth of a multi-layer structure and shearing stress occurring at the intersection of the pavement layer and/or the base and physical and mechanical characteristics of asphalt concrete and temperature. Designing guidelines only make allowances for mechanical loads. The other factors, that are highly ambiguous, are only accounted for with a few conditional coefficients. As a result, reinforced pavement fails before the expected period.
Therefore no guidelines regarding the methods of designing reinforcement structures and determining the character of the distribution of temperature fields and estimation of temperature strains of an asphalt concrete reinforcement layer makes it far more challenging to make design decisions.
1. Physical and mathematical model of designing a temperature field of a multilayered
airfield pavement. The dynamics of changes in the stress-strain of a multilayered airfield pavement under the effect of the temperature is impacted by its oscillation range and the character of the distribution in the depth of a structure depending on the construction parameters and thermal and physical characteristics of the materials of layers.
The difference between the temperatures in the pavement layers is due to molecular heat transfer, i.e. thermal conductivity. Heat transfer occurs in space and time and the temperature distributed inside an airfield structure can thus be presented as a function:
T f (x, y, h, τ) , |
(1) |
where x, y, h are spatial coordinates in the rectangular coordinate system; τ is the time.
52
Issue № 3(31), 2016 |
ISSN 2075-0811 |
A number of temperature values at all of the points of a particular space makes up a temperature field.
Experimental studies of a temperature mode of an airfield pavement show that changes in the temperature of a pavement are harmonic as those of the air temperature [1].
Each layer of an airfield pavement has a certain thickness Hi and thermal physical characteristics: the thermal conductivity coefficient λi, density ρi, specific thermal conductivity ci that change stepwise as they move from one construction layer to another and therefore the temperature field is not distributed evenly along the thickness of a pavement.
Hence temperature fields in a structure of an airfield pavement is assumed to be heterogeneous, non-stationary and harmonically changing.
Temperature fields inside an airfield structure are due to a combination of internal and external factors. The internal factors of thermal effects include conductive displacement of heat and eigen (effective) radiation of an airfield pavement surface. The external thermal effects make up natural and climatic and operational factors:
––constantly changing temperature of the air;
––total (direct and dispersed) solar radiation;
––atmospheric radiation and forced air convention;
––effects of gas steams of air engines.
The effects of operational factors are local and short-term with no significant influence on the temperature field of a pavement overall. According to V.M. Sidenko and S.A. Gridnev [2], migration and phase transition of moisture has no considerable effect on the thermal exchange of construction layers. Thus the effect of operational factors and thermal transfer inside the materials of construction layers in the calculation of temperature fields can be neglected.
The air temperature and solar radiation are particularly important as they are directly involved in the formation of a temperature field of an airfield structure. In order to determine temperature fields in a structure of an airfield pavement, the fundamentals of the non-stationary theory of heat conduction described by the Fourier equation [3, 4] are used [3, 4]:
|
|
2 |
|
2 |
|
2 |
|
|
|
λi |
|
|
Ti ai |
Ti |
|
Ti |
|
T2i |
, |
ai |
, |
(2) |
|||
2 |
2 |
|
||||||||||
τ |
|
x |
y |
h |
|
|
|
ρici |
|
where Ti is the temperature of the i-th layer; аi is the heat conductivity coefficient of the i-th layer; x, y, h are spatial coordinates in the rectangular coordinate system; τ is the time; λi is the heat conductivity coefficient of the i-th layer; ρi is the density of the material of the i-th layer; сi is the specific heat conductivity of the i-th layer.
53

Scientific Herald of the Voronezh State University of Architecture and Civil Engineering. Construction and Architecture
As the pavement is impacted by a one-dimensional temperature field and the ratio of the sizes of the pavement to the thickness is significant, the temperature in the thickness of a structure does not depend on the coordinates in the plan (x, y) and the equation is thus the following:
T |
a |
2T |
(3) |
i |
i . |
||
τ |
i h2 |
|
In order to identify the laws of the distribution of the temperature in the thickness let us determine the boundary and initial conditions.
The initial temperature T (h, 0) at any point hi in the thickness of a structure of the pavement is [5]:
|
T п h , 0 |
T в |
( ) |
Tг |
Tсрв ( ) Rп |
RH |
, |
(4) |
|
|
|
|
|||||
|
i |
ср |
|
|
R |
|
|
|
|
|
|
|
|
|
|
|
|
where T в |
( ) is the average temperature of the air in the period ∆τ, оС; Rп is the thermal resis- |
|||||||
ср |
|
|
|
|
|
|
|
|
tance characterizing the thermal exchange on the pavement surface, (m2·degrees)/Watt; Тг is the temperature of the subbase at the level of the foot of the last pavement layer outside the subbase, °С; i is the number of the construction layer of the pavement; ∑RH is the thermal resistance of the layers over h, h·degree·m2/Watt; R is the total thermal resistance of all the layers of an aircraft and subbase layers, h·degree·m2/Watt.
The boundary conditions are the following:
–– the amplitude of the temperature oscillations on the pavement surface is determined using the law of change in the air temperature and resulting heat flow:
|
T п (0, ) |
п |
в |
|
QRES ( ) Iэф , |
|
i |
1 |
|
||||
|
|
(0, ) Tср |
(5) |
|||
|
h |
T |
( ) |
|||
|
|
|
|
|
|
where Тп(0, τ) is a daily change in the temperature of the surface of the pavement, оС; QRES (τ) is a resulting heat flow impacting the upper pavement layer over the period ∆τ, Watt/m2;
Iэф is the value of the effective radiation of the surface of an airfield pavement, Watt·h/m2;
–– non-stationary heterogeneous temperature fields at the contact boundaries of the layers of the pavement and in the thickness of a structure as heat moves from the pavement surface to the foot and back (depending on the time of the day) are designed using conductive (qк (τ)) heat displacement. We admit that there is a perfect heat contact and physical mechanical characteristics of the materials are different for each layer:
λi T1 (hi , τ) |
|
λi 1 Ti 1 (0, τ) |
; |
(6) |
|
h |
h |
||||
|
|
|
54

Issue № 3(31), 2016 |
ISSN 2075-0811 |
The program startup
Input of the original data: location of the objects, thermal physical and physical mechanical characteristics of materials, geometry of the sections
Iteration process
Generation of the original massives
Obtaining the original distribution of the temperature fields:
Specifying the original and boundary conditions:
Calculating the matrices of the temperature characteristics of the elements:
Designing the matrices of the thermal conductivity of the system:
Calculating the radiation vector:
Calculating the vector of the nodal forces:
Solving the thermal conductivity task:
No |
Checking the consistency |
|
|
|
|
|
of the iteration process |
Yes
Designing the final distribution of the temperature at each point of a multi-layer structure
Output of the results
End
Fig. 1. Algorithm for modelling the temperature fields of a multi-layer airfield pavement
55

Scientific Herald of the Voronezh State University of Architecture and Civil Engineering. Construction and Architecture
–– oscillations of the temperature of the construction layers occur till they reach some depth of the natural subbase:
Tг |
(hг |
, ) |
const . |
(7) |
|
h |
|
||
|
|
|
|
The equation (3) for the specified initial (4) and boundary conditions (5)—(7) are solved using the finite element method by means of the heat transfer model of the radiation conductive thermal exchange for the i-th element in the COMSOL software according to the algorithm. The modelling algorithm is in Fig. 1.
2. Numerical modelling of a multi-layer airfield pavement for a thermal effect. Finiteelement modelling of the operation of a multi-layer airfield pavement for a thermal effect is performed in the software COMSOLMultiphysics using the physical mechanical model described above.
An area of an assembly airfield pavement reinforced with dense asphalt concrete with a airfield smooth plate on the crushed stone subbase. Its size is assumed to be 18×18 m. Along the perimeter the area is restrained with expansion joint along the entire artificial pavement. All the longitudinal and transverse deformation joints in assembly and joint windows inside the area have welded joints, which allows the effort to move from one plate to another.
As the impacts and deformations are symmetrical in relation to the centre of the investigated area, we will proceed to examine not the entire area but only its fourth sized 9×9 m (Fig. 2) to make the calculations easier to perform. The red arrows denote the deformations inside each plate caused by a reduction in the temperature of the surrounding air and the blue ones those inside the investigated area.
Fig. 2. Geometric model of the area of an airfield pavement: 1 — deformation joint; 2 — axis of the plate centre;
3 — temperature joint; 4 — symmetry plane
56

Issue № 3(31), 2016 |
ISSN 2075-0811 |
The lower boundary of the subbase is rigidly fixed. The levelling sublayer between the subbase and plates can be neglected in the model.
The calculation scheme of a multi-layer airfield pavement is presented in Fig. 3.
|
|
0,01 m |
|
|
|
|
3 m |
|
6 m |
|
|
||
|
|
|
|
|
||
|
|
|
|
|||
|
|
0,05÷0,2 m |
||||
|
|
|
|
|
|
0,14 m
0,5 m
0,05÷0,2 m 0,14 m 0,5 m
Fig. 3. The calculation scheme of a multi-layer airfield pavement:
1 — factors impacting the pavement; 2 — asphalt concrete reinforcement layer; 3 — smooth airfield plate; 4 — crushed stone subbase; 5 — line of zero temperature oscillations; 6 — rigid seal;
7 — mobile joints; 8 — statical joints (welded joints); 9 — asymmetry axis
The following assumptions are made:
––the original temperature of the pavement and foot of the structure is stable;
––convection and radiation heat exchange occurs not only through the upper layer of a structure, the rest are isolated;
––conductive heat exchange occurs due to the heat conductivity of the pavement;
––the pavement material is assumed to be thermoelastic;
––the temperature of zero stress in the pavement is 5 °С [6];
––the contact between the construction layers is ideal.
The temperature fields of a multi-layer airfield pavement is modelled over a 24-hour cycle for three temperature modes: positive temperature mode, variable temperature mode (moving through the ―0‖ threshold), negative temperature mode.
57

Scientific Herald of the Voronezh State University of Architecture and Civil Engineering. Construction and Architecture
The temperature modes are designed in two steps. Firstly, the external factors and original conditions are specified and the initial distribution of the temperature fields along the thickness at the averaged temperature of the surrounding air in the investigated road climate area. Secondly, the fields of the distribution of the temperature in the thickness of an airfield structure for radiation convection exchange of the upper layer with the air is determined.
As a result of modelling the isofields are obtained in the pavement (Fig. 4), the graphs of change in the temperature deep in the pavement over a 24-hour cycle are designed (Fig. 5).
The obtained finite-element model of a multi-layer airfield pavement implemented in the software COMSOL Multiphysics can be employed to determine the law of the oscillations of the temperature in the plane of the contact of the layers ∆Тгр.
а) |
b) |
Fig. 4. Temperature isofields in the airfield pavement:
а) along the thickness; b) in the plan
58

Issue № 3(31), 2016 |
ISSN 2075-0811 |
Foot of the airfield smooth plate 14
Fig. 5. Distribution of the temperature along the depth of an airfield pavement in winter with the thickness of the
reinforcement layer of 5 сm and maximum oscillations of the air temperature
Let us design an experimental factor model based on the calculation active experiments in COMSOL using the obtained finite-element model.
For that we specify the controlled and managed factors impacting the object in question: the thickness of the reinforcement layer Hsup, mm; thermal conductivity λsup, Wt/m·°С; amplitude of the oscillations of the air temperature ∆Тв, °С. The response function
Y f sup , Hsup , TВ Tгр
is the temperature gradient at the boundary of joining of the construction layers at nonstationary temperatures.
In order to obtain the second-order regression model, rotatable central compositional uniform second order plan was designed.
Using the results of the calculation experiment, a regression model of the dependence of the oscillations of the temperatures at the boundary of joining ∆Tгр of the asphalt concrete layer on the thermal conductivity of asphalt concrete λ, thickness of the reinforcement layer H and amplitude of the oscillations of the air temperature ∆T:
59
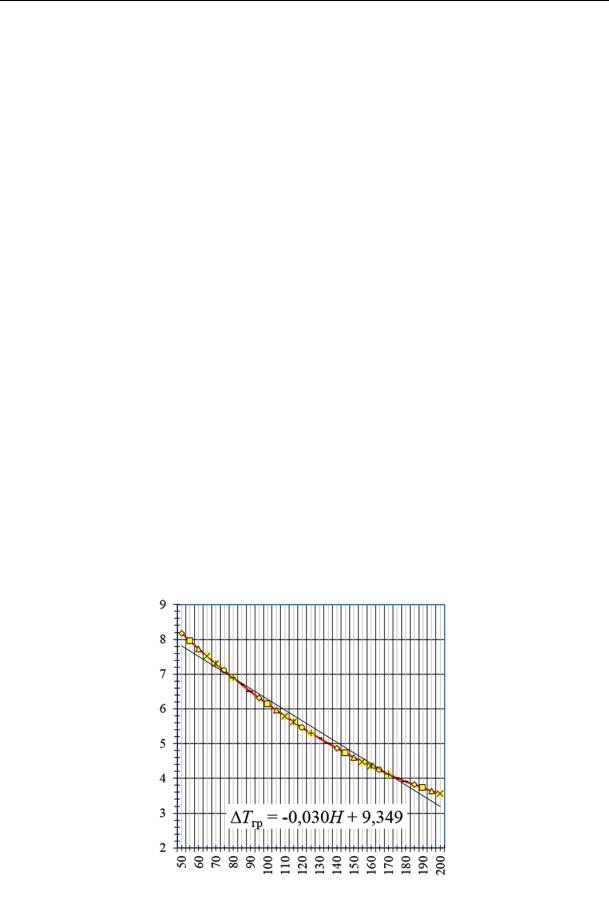
Scientific Herald of the Voronezh State University of Architecture and Civil Engineering. Construction and Architecture
T |
2,5709 7,3814 0, 0392 H 0, 2778 Т 3, |
0119 2 |
гр |
|
(8) |
|
|
|
0, 0001 H 2 0, 0005 T 2 0, 0054 H 0, 0786 T |
0, 001 H T. |
The estimation of the obtained regression model using the Fisher criterion
F 50,839 Fкр 4, 41
and the determination coefficient
R2 0,99 0, 75
showed it to be suitable for use.
The analysis of the mathematical model (8) allows one to estimate the effect of the investigated factors on ∆Tгр. Using the pairs of variables, first order variables and quadratic ones thermal conductivity of a material has a significant effect on the temperature gradient. The combination of the thermal conductivity of asphalt concrete and amplitude of the oscillations of the temperature causes ∆Tгр to increase.
However the analysis of the graphs (Fig. 6—8) designed using the expression (8) shows that the considerable oscillations of the temperature at the boundary of joining of the layers ∆Tгр occur as the thickness of the asphalt concrete reinforcement layer drops or the amplitude of the oscillations of the air environment increase. These dependencies are exclusively linear. The thermal conductivity of asphalt concrete layer of growth has no effect on the temperature gradient ∆Tгр for a fixed average thickness of the growth layer and amplitude of the air as it is fairly small.
Temperature oscillations along the thickness, ̊C
Thickness of asphalt concrete, mm
Fig. 6. Dependence of the temperature gradient ∆Tгр on the thickness of the asphalt concrete reinforcement layer
60