
2918
.pdfIssue № 1 (37), 2018 |
ISSN 2542-0526 |
termined according to the first law by Konovalov considering the Raoult’s and Dalton’s law and the Antoine equation [11]:
|
|
i |
|
|
|
|
1- i |
|
|
|
|
|
||
Х Р |
|
|
|
|
|
|
|
|
, |
(4) |
||||
|
|
А |
|
В2 |
|
|
А |
В1 |
|
|
||||
|
|
|
|
|
|
|
|
|||||||
|
|
Р 10 |
2 |
С2 t |
|
|
Р 10 |
1 |
С1 t |
|
|
|||
|
|
|
|
|
|
|
|
|
|
|
|
|
where Р is an absolute pressure of the zeotropic mixture in the evaporator and condenser of the heat pump, Pа.105; А1, В1, С1 are the coefficients that are typical of a component with a lower temperature of boiling and condensation at the pressure of the mixture Р in certain temperature ranges t; А2, В2, С2 are the coefficients that are typical of a component with a higher temperature of voiling and condensation at the pressure of the mixture Р in certain temperature ranges t. The coefficients А1, В1, С1 and А2, В2, С2 used in the formula (4) are given in [14].
The temperature of the start of boiling of the zeotropic mixture in the evaporator is calculated in the Celcius degrees using the formula (4) when a current temperature t is made equal to tси.н , i.e. t = tси.н at the relative amount of the boiled away zeotropic mixture Х = 0 and the end of boiling t = tси.к at Х = 1. The temperature of the start of condensation of the zeotropic mixture in the condenser t = tскд.н is determined in the Celcius degrees using the formula (4) at the relative amount of the condensed zeotropic mixture Х = 1 and the end of condensation t = tскд.к at Х = 0. t at specified Х is calculated using the formula (4) by means of the trial-and-error method. Note that a difference caused by the use of the calculation formula (4) based on Dalton’s and Raoult’s laws and the Antoine equation for zeotropic mixtures particularly for saturated hydrocarbons at the absolute pressure of up to 1.0 МPа with the data of immediate measurements is 4.5 % [20].
Changes in the temperature of the boiled away or condensed mixture in the range of changes in the dryness Х from 0 to 1.0 first of all causes changes in the temperature of the heated or cooled medium with a limited heat capacity. The initial temperature of a medium with a limited heat capacity, e.g., the air depending on changes in the temperature, dryness and consumption of the zeotropic mixture for the counterflow heat exchanger is determined using the equilibrium equation between a working substance and medium with a limited heat capacity in the evaporator and condernser of the heat pump:
–– for the evaporator with a limited heat capacity:
|
|
|
и |
и |
и |
X |
и |
и |
и |
и |
|
|
|
t |
и |
|
Gв св |
tв.к Gc rс |
с |
cс |
(tс.к tс.н) |
; |
(5) |
||||
в.н |
|
|
cи |
G |
|
|
|
|
|
||||
|
|
|
|
|
|
|
|
|
|
|
|||
|
|
|
|
|
в |
в |
|
|
|
|
|
|
|
61

Russian Journal of Building Construction and Architecture
–– for the condenser:
|
|
|
кд |
кд |
|
кд |
кд |
кд кд |
|
|
|
t |
кд |
|
Gв св |
tв.к Gc rс Хс |
cс |
(tс.н tс.к ) |
. |
(6) |
|||
в.н |
|
|
cкд G |
|
|
|
|||||
|
|
|
|
|
|
|
|
|
|||
|
|
|
|
|
в |
в |
|
|
|
|
|
Here Gс, Gв is a molar consumption of the zeotropic mixture and a medium of a limited heat capacity, mole/h; rcи, rcкд is the average latent heat of evaporation (vapor formation) of the zeotropic vapor and liquid mixture in the evaporator and condenser, kJ/mole; сви, свкд is the average specific heat capacity of a medium with a limited heat capacity, e.g., the air in the evaporator and condenser, kJ/(mole К); сси, сскд is the average specific heat capacity of the zeotropic vapor and liquid mixture in the temperature range of boiling away and condensation in the evaporator and condenser, kJ/(mole К); Xси, Xскд is a relative amount of the boiled away or condensed (dryness) zeotropic mixture in the evaporator and condenser.
Working substances for the zeotropix mixture and molar concentration of its low-boiling component that a minimum difference between the average temperatures correspond with are chosen in the following sequence.
1. A zeotropic mixture consisting of two components is chosen that has
––an initial condensation temperature tскд.н that is larger than a medium with a limited heat capacity compared by the temperature pressure that is assumed to be about tкд = 5.0 7.0 К;
––an initial boiling temperature tси.н that is smaller than a heat source with a limited heat ca-
pacity by the temperature pressure that is assumed to be about tи = 5.0 7.0 К.
2. According to the formula (4) using the method of selection, the absolute pressures Ркд, current t and final condensation temperatures in the condenser tскд.к as well as the absolute pressures Ри, current t and final boiling temperatures in the evaporator tси.к are determined for different values of molar concentration ψi = ψн,…ψх,...ψy,…ψk, mole %.
3. Calculations to determine the difference tскд.ср tси.ср using the formulas (2) or (3) for a
range of values of molar concentration ψi = ψн,…ψх,...ψy,…ψk and then the minimum value is selected.
Therefore according to the suggested method, the components of a zeotropic mixture and concentration of its low-boiling component are determined that will provide a minimum difference between the temperatures tскд.ср tси.ср min and thus a maximum energy efficiency of the heat pump.
62
Issue № 1 (37), 2018 |
ISSN 2542-0526 |
Based on the suggested methodological assumptions, the components of a zeotropic mixture and the concentration with a lower boiling temperature were chosen when a minimum difference is provided between its temperatures in the condenser and evaporator of the heat pump used for heating and colling of media with a limited heat capacity in systems of gas and heat supply and ventilation.
The selection was performed using the example of two zeotroipc mixtures with each having similar physical properties of the components:
––a zeotropic mixture “R600а (isobutane) — R601 (n-pentane)” with the concentration of a low-boiling component R600а (isobutane) accepted in the range ψi = 0.0 100.0 mole % with the step 2.0 mole %.
––a zeotropic mixture “R290 (propane) — R600 (n-butane)” with the concentration of a low-
boiling component R290 (propane) accepted in the range ψi = 0.0 100.0 mole % with the step 2.0 mole %.
The temperature pressure between heat-exchanging flow in the counterflow condenser and evaporator is accepted to be tкд = tи =7 0С. The temperature of a medium with a limited heat capacity at the output of the condenser is tвкд.к = 65 0С, then
tскд.н tвкд.к tк
is tскд.н = 65+7 = 72 0С.
The temperature of a medium with a limited heat capacity at the output of the evaporator is tвкд.н = 43 0С, then
tси.н = tви.к − tи
is tси.н = 43−7 = 36 0С.
According to the results of the calculations, a minimum difference between the average temperatures of the condensation and boiling ( tскд.к −tси.ср ) =18 оС = min based on the expression
(4) is achieved for a zeotropic mixture “R600а (isobutane) — R601 (n-pentane)” for the molar concentration of a low-boiling component R600а (isobutane) in the mixture is ψi = 45 mole %. The results of the calculations also show that as the molar concentration of a low-boiling component which is ψi = 0.0 mole% and ψi = 100 mole% is achieved, the mixture turns into a pure substance with constant condensation temperatures of the vapor phase tcкд. 0,0% const , tcкд. 100% const in the condenser and with constant evaporation temperatures of the vapor phase tcи.ψ=0,0% const , tcи.ψ=100% const in the evaporator.

Russian Journal of Building Construction and Architecture
According to the expression (1), the difference between the average temperatures of condensation in the condenser and that of boiling increases up to the maximum values that are
tcкд. 0,0% tcи. 0,0% = 36 оС, tcкд. 100,0% tcи. 100,0% = 36 оС,
and conversely, the energy efficiency of the heat pump decreases down to the minimum.
The cycle of the heat pump at ψi = 0.0 mole% and ψi = 100 mole% (see Fig. 2) is presented as a -b -c -d -a . According to the calculations, the temperatures of heated and cooled media with a limited heat capacity have a significant effect on the choice of the components of a zeotropic mixture and concentration of its component with a lower temperature of boiling. Changes in the temperatures of a medium with a limited heat capacity at the output of the condenser tвкд.к and evaporator tвкд.н causes a choice of other brands of zeotropic mixtures and other values of the proportion of a low-boiling component in them.
Conclusions. 1. The analysis of possible use of working substances as components of a nonazeotropic mixture in heat pumps showed that the mixtures R22/R142b, R32/R134а, R32/R152а have a lower ozone-depletion activity and mixtures of saturated hydrocarbons R290/600 (propane and butane), R600а/R601 (isobutene and n-pentane), R290/ R601а (propane and isopentane), R600а/R601b (isobutene and new pentane) are considered completely safe based on the degree of the depletion of the Earth’s ozone layer. These gases do not have any significant greenhouse effect and no impact on climate change, human body. Mixtures of hydrocarbons are more cost-efficient than other working substances.
2.The novelty of the suggested method is that minimum differences between the eaverage temperatures of condensation and boiling are achieved by choosing each of the two components of a zeotropic mixture with similar physical properties and the molar concentration of its lowboiling component ψi based on the dependence of the temperature of its saturation in the condenser and evaporator of the heat pump on a relative amount of the boiled away mixture.
3.The temperatures of heated and cooled media with a limited heat capacity are proved to have a significant effect on the choice of the components of a zeotropic mixture and the molar concentration of its low-boiling component ψi when a minimum difference between the average temperatures of condensation and boiling and thus a maximum energy efficiency is achieved.
References
1. Bukin V. G., Kuz'min A. Yu. Kholodil'nye mashiny, rabotayushchie na neazeotropnykh smesyakh khladogentov
[Refrigerating machines, working on non-azeotropic mixtures of refrigerants]. Astrakhan, AGTU Publ., 2007. 156 p.
64
Issue № 1 (37), 2018 |
ISSN 2542-0526 |
2.Bukin V. G., Kuz'min A. Yu. Eksperimental'noe issledovanie malykh kholodil'nykh mashin na smesi R22/R142b [Experimental study of small refrigerating machines in a mixture of R22/R142b]. Kholodil'naya tekhnika, 1996, no. 5, pp. 12—14.
3.Kiotskii protokol k ramochnoi konventsii Organizatsii Ob"edinennykh Natsii ob izmenenii klimata [The Kyoto Protocol to the framework Convention United Nations on climate change]. Available at: http://bellona.ru/2007/05/08/kiotskij-protokol-k-ramochnoj-konvents (accessed 05.04.17).
4.Kutepov A. M., Sterman L. S., Styushin N. G. Gidrodinamika i teploobmen pri paroobrazovanii: ucheb. posobie dlya vuzov [Hydrodynamics and heat transfer during evaporation: proc. manual for schools]. Moscow, Vysshaya shkola Publ., 1977. 352 p.
5.Martynovskii V. S. Tsikly, skhemy i kharakteristiki termotransformatorov [Cycles, schemes and characteristics of thermoformers]. Moscow, Energiya Publ., 1979. 285 p.
6.Mikheev M. A., Mikheeva I. M. Osnovy teploperedachi [Basics of heat transfer]. Moscow, Energiya Publ., 1973. 320 p.
7.Monreal'skii protokol po veshchestvam, razrushayushchim ozonovyi sloi [The Montreal Protocol on substances that Deplete the ozone layer]. Available at: http://www.un.org/ru/documents/decl_conv/conventions/ pdf/montreal.pdf (accessed 05.04.17).
8.Ogurechnikov L. A., Mezentseva N. N. Analiz effektivnosti ispol'zovaniya smesei ozonobe-zopasnykh khladogentov v parokompressionnykh teplovykh nasosakh [Analysis of the efficiency of using mixtures of ozonesafe refrigerants in steam compression heat pumps]. Energetika i teplotekhnika, 2008, no. 12, pp. 57—66.
9.Ogurechnikov L. A. Kondensatsiya R32/R134a v tekhnologii teplonasosnogo teplosnabzheniya [Condensation of R32/R134a in heat pump technology heating]. Kholodil'naya tekhnika, 2011, no. 2, pp. 46—48.
10.Preobrazhenskii N. I. Szhizhennye gazy [Liquefied gas]. Leningrad, Nedra Publ., 1975. 227 p.
11.Rulev A. V., Usachev A. P., Shuraits A. L., Usacheva T. A. Sistemnye issledovaniya po povysheniyu intensivnosti teploobmena regazifikatorov szhizhennogo uglevodorodnogo gaza [System researches on increase of heat exchange intensity of liquefied petroleum gas regasifiers]. Saratov, Sarat. gos. tekhn. un-t Publ., 2010,
244p.
12.Staskevich N. L., Vigdorchik D. Ya. Spravochnik po szhizhennym uglevodorodnym gazam [Handbook of liquefied hydrocarbon gas]. Leningrad, Nedra Publ., 1986. 543 p.
13.Sukhikh A. A., Generalov K. S., Akimov I. A. [Tests of the heat pump for heat supply of the individual house]. Trudy MGUIE «Tekhnika nizkikh temperatur na sluzhbe ekologii» [Proc. MSUEE "low temperature systems in the service of ecology»]. Moscow, MGUIE Publ., 2000, pp. 49—53.
14.Tilicheev M. D. Fiziko-khimicheskie svoistva individual'nykh uglevodorodov. Vyp. 2 [Physical and chemical properties of individual hydrocarbons. Vol. 2]. MoscowLeningrad, Gostoptekhizdat Publ., 1947. 458 p.
15.Usachev A. P., Rulev A. V. Razrabotka metodicheskikh polozhenii po teplovomu raschetu promyshlennykh trubnykh isparitelei smesei szhizhennogo uglevodorodnogo gaza [Development of methodical provisions on thermal calculation of industrial pipe evaporators of mixtures of liquefied petroleum gas]. Teplovye protsessy v tekhnike, 2013, vol. 5, no. 8, pp. 343—353.
16.Ho-Saeng Lee, Hyeon-Ju Kim, Dong-gyu Kang, Dongsoo Jung. Thermodunamic performance of R32/R152a mixturu for water source heat pumps. Enege, 2012, vol. 40, iss. 1, pp. 100—106.
65

Russian Journal of Building Construction and Architecture
17.Jianyong Chen., Janlin Yu. Performance of a new refrigeretion cycle using refrigerant mixture R32/R134a for resintial air-conditijner applications. Enerdy and Buildings, 2008, no. 40 (11), pp. 171—179.
18.Kim M., Kim M. S., Kim Y. Experimental study on the performance of a heat pump system with refrigerant mixtures’ composition change. Energy, 2004, vol. 29, iss. 7, pp. 1053— 1068.
19.Kim T. S., Shin J. Y., Kim M. S., Ro S. T. Cycle analysis and heat transfer characteristics of a heat pump
using R22/ R142b refrigerant mixtures. International Journal of Refrigeration, 1994, vol. 17, no. 6, pp. 391—399.
20.Nysewander C. H., Sage B. H., Lesey W. N. Phase Equillbria in hydrocarbon systems. Industrial and Engineering Chemistry, 1940, vol. 32, no 1, pp. 118—123.
21.Shurayts A. L., Rulev A. V., Usacheva E. Yu. Assessing Energy Efficiency of Compression Heat Pumps in Drying Processes when Zeotropic Hdrocarbon Mixtures are Used as Working Agents. International Conference «Topical Problems of Architecture, Civil Engineering, Energy Efficiency and Ecology: MATEC Web Conf., 2016, vol. 73, pp. 1—9. doi: 10.1051/matecconf/20167302015. Available at: https://www.matecconferences.org/articles/matecconf/pdf/2016/36/matecconf_tpacee2016_02015.pdf (accessed 05.04.17).
66

Issue № 1 (37), 2018 |
ISSN 2542-0526 |
DESIGNING AND CONSTRUCTION OF ROADS, SUBWAYS,
AIRFIELDS, BRIDGES AND TRANSPORT TUNNELS
UDC 624.21:533.6 : 699.83
V. A. Kozlov1
STRESS-STRAIN OF ELEMENTS OF BRIDGE STRUCTURES WITH A VARYING THICKNESS OF WALLS ALONG THE LENGTH
Voronezh State Technical University
Russia, Voronezh, tel.: (473) 276-40-06, e-mail: v.a.kozlov1@yandex.ru
1D. Sc. in Physics and Mathematics, Prof., Head of the Dept. of Theoretical and Applied Mechanics
Statement of the problem. During erection of bridge passageways spans can be thin-walled structures with one fixed transverse section. With elements of bridge structures being fixed in an uncommon way, during assembly the distribution of stresses might differ significantly from stress fields during the operation of the end object. A preliminary calculation of the stress-strain of construction elements during erection allows safe assembly during bridge construction.
Results. Stress fields in a thin-walled weakly conic structure with a rectangular contour of a transverse section solidly fixed along a skewed edge of one support contour with the other one free. Unlike other popular papers, in this one a varying thickness of the walls of a structure along its length is taken into account. The solutions are presented both numerically and analytically using a tool of special functions. Numerical examples of calculations under a transverse force and a concentrated rolling moment applied to a free end of a structure are presented.
Conclusions. While a considered thin-walled structure is being rolled, there is an edge effect both in the plane of the solid fixation due to the tightness of warping of the contour and at the end section due to the conic shape and change in the thickness. Considering warping of the transverse contour due to a bend has little effect on the stress-strain and can thus be neglected in actual designing.
Keywords: stress-strain, elements of bridge structures, varying thickness.
Introduction. During the erection of different building structures in order to optimize their performance during the assembly and operation, it is necessary to provide even distribution of stresses in construction elements under the impact of applied loads and forces. In some cases it is achieved by varying geometric parameters, construction shape, thickness, fastening, etc.
© Коzlov V. А., 2018
67

Russian Journal of Building Construction and Architecture
As for bridge spans, during assembly they are commonly a structure that has no support at the ends and there is a console with a fastened transverse section where stresses can be distributed significantly differently from the way they are in an assembled span. The assembly period up to the final stage may take a few years when construction elements are impacted by a variety of force factors and loads as well as a low bending and rolling rigidity at different stages of the assembly. Thus it is necessary to perform corresponding calculations and develop a set of measures to ensure safety of the assembly of construction elements during the erection of bridges or other building structures.
Development of the theory and practical calculation methods for elements of bridge structures as well as thin-walled systems has been addressed in a lot of studies, e.g., [3, 7, 10—12], etc. The current paper looks at the stress-strain of a skew cone structure supported by a transverse and longitudinal set of a regular structure with a rectangular contour of a transverse section that can be singly or multiply connected. Besides, the walls of a structure along its length are supposed to be of a varying thickness. The transverse set (stringers) can also have a varying area of the transverse section. Fig. 1 shows a calculation model of the above structure.
|
d1 |
|
|
h1 |
|
d2 |
l |
h2 |
|
||
|
|
|
|
O |
|
|
x0 |
|
x
y |
l1 |
Mx |
Qy |
z |
|
||||
|
|
|
Mz |
|
|
|
|
|
l0 |
Fig. 1. Calculation model of a construction element
General solutions obtained in the analytical form using a tool of special functions for prismatic structures with a multiply isolated contour of the transverse section are presented in [6, 9], free oscillations are dealt with in [8].
68
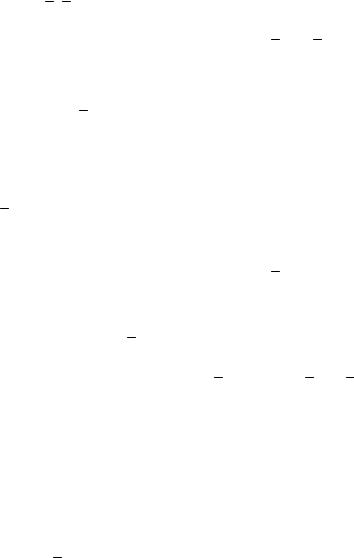
Issue № 1 (37), 2018 |
ISSN 2542-0526 |
1. Resolving system of regular differential equations and its analytical solution. A system of resolving differential equations
6 n |
|
|
bijVi cjiVi |
/ Z |
|
Rj / jG |
(1) |
|||||
ZajiVi |
bjiVi |
|
|
|||||||||
i 1 |
|
|
|
|
|
|
|
|
|
|
||
and natural boundary conditions |
|
|
|
|
|
|
|
|
|
|
||
|
|
|
|
|
|
|
|
|
|
|
|
|
Pj Pj U j |
|
Z |
Z |
0, j=1, 2,… 6 + n, |
(2) |
|||||||
|
|
|
1 |
|||||||||
Z |
0 |
obtained based on the variational principle by Lagrange are assumed to be as in [7]. Here aji ,bji,сji are the coefficients that depend on the law of changes in the thickness of the wall of a structure along its length and area of the transverse section of the stringer set; Z 1 Z,Z z / l is a relative coordinate along its full length l ; Rj is an operator that corresponds with the external load; Vi Ui Z i Z , Vi are unknown functions and their z derivatives; Ui are initial generalized movements, first three of which are displacements of the contour Z const as a sold body along the axis Ox,Oy,Oz , U4,U5,U6 are rotation angles of this contour along the axis and the remaining n degress of freedom determine warping of the contour; j Z for j 4,5,6; j 1 for the other values of j; G is a shear modulus;
Pj , Pj are specified and unknown generalized forces in these sections of a structure; U j are variations of the generalized movements.
Let us assume that the pole of the contour Z const is a point of the intersection of a plane of this contour with the axis Oz. Then a vector function i (S) that is responsible for the displacement of the contour Z const as a solid body in the resolution of an elastic movement vector
|
|
|
|
6 n |
|
|
|
|
|
|
|
U |
Z |
, S |
Ui Z |
i |
Z |
|
i S , |
||
|
|
|
|
i 1 |
|
|
|
|
|
|
is determined using the expressions |
|
|
|
|
|
|
|
|||
|
|
|
|
|
k , |
|
|
|
|
|
1 |
i , |
2 |
j, |
3 |
4 |
y0k |
x0ctg 0 j, |
|||
|
|
|
|
|
|
|
|
|
|
|
|
5 x0 (ctg 0i k ), |
6 |
x0 j |
y0i . |
The components of the coordinate vector functions 7 and 8 associated with warping of the contour Z const caused by bending and rolling respectively are as follows
|
71 |
x y |
, |
x2 y |
0 |
y |
, |
|||
|
0 |
0 |
|
81 |
0 |
|
0 |
|
||
|
72 7n 0, |
82 |
8n 0, |
69

Russian Journal of Building Construction and Architecture
where is the coefficient of orthogonalization:
|
|
|
|
|
|
x2 y dS |
|
y2dS. |
|
|
|||||
|
|
|
|
|
|
|
|
|
|
0 |
0 |
|
0 |
|
|
|
|
|
|
, let us present (1) as |
|
||||||||||
Moving to the absolute coordinate z l0Z |
|
||||||||||||||
6 n |
|
|
|
|
|
|
|
|
|
|
|
|
|
|
|
|
bjiVi Pj / jG,( j 1,2,...,6); |
|
|
||||||||||||
ZajiVi |
|
|
|||||||||||||
i 1 |
|
|
|
|
|
|
|
|
|
|
|
|
|
|
(3) |
6 n |
|
|
|
|
|
|
|
|
|
|
|
|
|
|
|
|
bijVi |
|
|
|
Rj |
/ jG,( j 7,8,...,6 |
n), |
||||||||
ZajiVi bjiVi |
cjiVi / Z |
||||||||||||||
i 1 |
|
|
|
|
|
|
|
|
|
|
|
|
|
|
|
where Pj for j = 1, 2, 3 are the components of the main vector of the external load applied to
the cut off part of a structure and for j = 4, 5, 6 are the components of the main moment of external forces in relation to the top of a conic surface. Resolving the system of equations (3) in relation to the initial unknown values and adjusting the solutions to the boundary conditions (2), it is easy to find the constant integrations of the task. E.g., if the contour Z const has 6 + n degrees of freedom, the boundary conditions are reduced to 6 + n kinematic and static boundary conditions at each face of the shell. If a section is rigidly fastened, kinematic boundary conditions are reduced to the equation to the zero of generalized movements:
|
|
|
|
U j |
|
|
|
|
|
0, ( j 1, 2,..., 6 n) , |
|
(4) |
||||||
|
|
|
|
|
|
|
|
|
|
|||||||||
|
|
|
|
|
Z 0 |
|
||||||||||||
i.e. in this case the variation U j |
|
|
|
|
|
|
|
|
|
|
|
|
0 is met. Assuming that |
|||||
|
|
|
|
0 |
|
|
|
|
||||||||||
|
|
|
|
and (2) for the section Z |
||||||||||||||
|
|
Z |
0 |
|
|
|
|
|
|
|
|
|
|
|
|
|
|
|
|
|
|
|
|
|
|
|
|
|
|
|
|
|
|
|
|||
in the end section z l there are concentrated force factors, (2) |
yields the equation P P* |
|||||||||||||||||
1 |
|
|
|
|
|
|
|
|
|
|
|
|
|
|
|
|
j |
j |
that if when expanded, yields |
|
|
|
|
|
|
|
|
|
|
|
|
|
|
|
|
|
|
|
|
|
|
|
6 n |
|
6 n |
|
|
|
|
|
|
|
||||
|
|
|
|
|
|
|
|
|
|
|||||||||
G j |
Z ajiVi bjiVi |
|
|
|
Pj* (Z1). |
(5) |
||||||||||||
|
|
|
|
|
|
i 1 |
|
i 1 |
|
|
Z Z |
|
|
|
|
|||
|
|
|
|
|
|
|
|
|
|
|
|
1 |
|
|
|
|
||
Note that the generalized forces Pj |
are a sum of the operation of internal efforts in the section |
Z const of a structure on geometrically possible movements determined with the conditions
1, j i,
U j 0, j i.
E.g., let in the end section z l1 of the above structure a concentrated force Qy and moments Mx ,Mz be applied. Then for a weakly conic structure with a rectangular contour of a transverse section the static boundary conditions (5) in the end section z l1 are reduced to the following system of equations:
70