
Учебное пособие 2254
.pdf
Russian Journal of Building Construction and Architecture
Thus, when analyzing the research carried out, the features of heat transfer through the outer corner, the main solutions were obtained in the form of empirical dependences of determining the temperature in the corner of the outer walls. In addition, they consider only the design of the outer corner and do not take into account the thermal properties of internal enclosing structures, such as floor slabs, partitions, windows and their effect on the temperature on the inner surface of the outer corner.
It should also be noted that all the obtained empirical equations characterize a stationary mode that does not take into account the change in the air temperature inside the room. According to [17], this is due to the fact that "the mathematical relationship between the temperature on the surface of the wall and other thermal properties of the wall has not been established".
1. Theoretical justification for calculating the temperature in the outer corner. Calculation of temperature fields of external enclosing structures and their elements by finite element methods according to modern programs is not always possible for most designers. Determination of the patterns characterizing the change in temperature in the corner of the enclosing structure is an important task that must be solved by the method of engineering calculations at the design stage. In this regard, the author, in the development of research by O. E. Vlasov [5], L. A. Semenova [15] and K. F. Fokin [17], the problem of determining the temperature along the axis of the outer corner is solved, taking into account the amplitude of oscillations of the internal air in the room Аt. In this case, the calculation takes into account the discontinuity of the operating heating, in particular, using the example of fluctuations in the temperature of the indoor air of the room from stove heating.
Let us consider the case when the amplitude of the heat flux oscillation Aq occurs between the conditional inner surface of the outer corner Qcor and the inner surface of the smooth surface of the wallQs, and determining the value ofAq
|
Аq |
Qcor Qs , |
|
|
|
|
|
|
(6) |
|
or |
|
|
|
|
|
|
|
|
|
|
А cor (t |
int |
cor ) |
int |
(t |
int |
|
int |
), |
(7) |
|
q |
int |
int |
|
|
|
|
where intcor – heat transfer coefficient oftheconditionalinner surfaceoftheoutercorner,W/m20C;
int – heat transfer coefficient on the surface of the wall, W/m2 0C; intcor – temperature on the inner surface of the corner, 0С; int – temperature on the inner surface of the wall surface, 0C. In equation (7), the surface area is 1 m2.
10
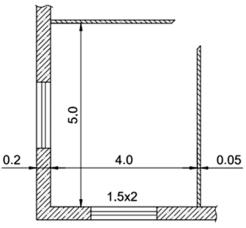
Issue № 4 (52), 2021 |
ISSN 2542-0526 |
By performing a series of transformations of the temperature equation on the inner surface of
the wall surface |
int |
t |
int |
|
tint |
text |
R taking into account formula (7), we obtain an equation |
|
|
||||||
|
|
|
|
|
int |
||
|
|
|
|
|
|
R0 |
|
for calculating the temperature on the inner surface of the outer corner |
cor t |
|
t |
int |
t |
Аq |
|
|
|
|
|
ext |
|
|
. |
(8) |
||
|
|
|
cor |
|||||
int int |
|
cor R |
|
|
||||
|
|
|
|
int 0 |
int |
|
|
Since the amplitude of the heat flux is Aq = Aq В, then the equation for calculating the temperature of the inner surface of the outer corner will be
|
|
|
t |
int |
t |
ext |
|
АВ |
|
|
cor t |
|
|
|
|
|
t |
. |
(9) |
||
|
|
|
|
|
|
|||||
int |
int |
|
cor R |
cor |
|
|||||
|
|
|
|
|
int 0 |
int |
|
When solving the equation for calculating the temperature in the corner of the outer wall based on the analysis of the heat-shielding properties of wall structures and the parameters of the air environment of the room for the inner surface of the outer corner, we take the heat transfer coefficient corint 5.0 W/m2 0C.
Let us find the amplitude of fluctuations in the temperature of the internal air in the living quarters of the first floor of a two-story cottage (Fig. 1), located in the Voronezh region. The room has dimensions of 4 × 5 m, height from floor to ceiling h = 2.5 m. The room is heated 1 and 2 times a day. We will take in accordance with building codes the temperature of the internal air tint = 20 0С and outdoor temperature text = –25 0С.
Fig. 1. Floor-to-ceiling floor plan h = 2.5 m and an area of 20 m2 in a cottage
This room has the following enclosing structures:
1. The outer walls of the cottage consist of a wooden beam with a section of 0.2 × 0.2 m. Heat transfer resistance of the wall along the surface R0 = 1.27 m2 0C/W and the heat transfer coefficient K = 0.787 W/m2 0C.
11
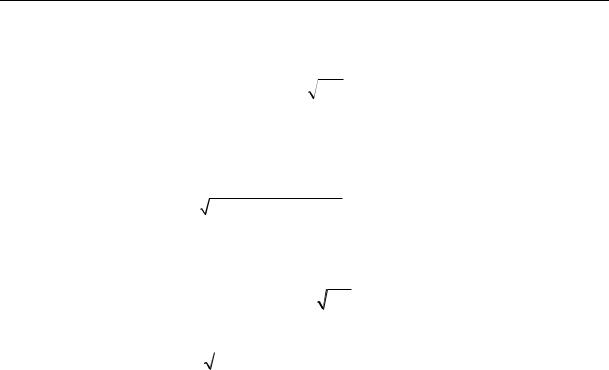
Russian Journal of Building Construction and Architecture
The coefficient of heat assimilation of the wall material, s is determined for furnace heating of the room 2 times a dayaccording to the formula
s12 0.012 с , (10)
where λ – thermal conductivity coefficient of wood, equal to 0.18 W/(m0C); с – specific heat capacity ofa wooden beam across the fibers equal to 2.51 kJ/(kg0С); γ – density ofa wooden beam equalto 550 kg / m3. Substituting the valuesoftheparameters into formula(10), weobtain
s12 0.0120.18 2.51 1000 530 5.98 W /(m2 0С).
The coefficient of heat assimilation of the wall material s is determined for furnace heating of a room with a firebox once a day according to the formula
|
s24 0.0085 с . |
(11) |
|
Substituting the values of the parameters into the formula and we get |
|
||
s24 0.0085 |
|
4.23 W /(m2 |
0С) . |
0.18 2.51 1000 530 |
Since the thermal inertia of the cobbled wall is D > 1 and D = Rs = 1.27 ∙ 2.51 = 3.18, the heat absorption coefficient of the inner surface Yint will be equal to the heat absorption coefficient of the material s. Then we can write
|
Y |
s |
|
5.98 |
W /(m2 0С), |
(12) |
|||||||||
|
12 |
|
12 |
|
|
|
|
|
|
|
|
|
|
|
|
and |
Y |
|
s |
24 |
4.23 W /(m2 0С). |
(13) |
|||||||||
|
24 |
|
|
|
|
|
|
|
|
|
|
|
|
||
The heat absorption coefficient of the surface for the period z = 12 hours will be |
|
||||||||||||||
|
|
|
|
В |
|
|
|
1 |
|
|
. |
(14) |
|||
|
|
|
|
|
|
|
|
|
|||||||
|
|
|
|
|
12 |
|
1 |
|
|
1 |
|
|
|
||
|
|
|
|
|
|
|
Y12 |
int |
|
||||||
|
|
|
|
|
|
|
|
|
|||||||
Heat absorption coefficient of the surface for the period z = 24 hours |
|
||||||||||||||
|
|
|
|
В |
|
|
1 |
|
. |
(15) |
|||||
|
|
|
|
|
|
|
|||||||||
|
|
|
|
|
24 |
|
1 |
|
|
|
1 |
|
|
|
|
|
|
|
|
|
|
|
|
Y24 |
|
int |
|
||||
|
|
|
|
|
|
|
|
|
|
|
Substituting the values into formulae (14) and (15), we obtain the numerical values of the heat absorption coefficients of the surface of the outer walls:
|
В |
|
|
|
1 |
|
|
|
3.32 W /(m2 |
0С) |
|
|
1 |
|
|
1 |
|||||||
|
12 |
|
|
|
|
|
|
||||
|
|
|
5.98 |
|
8.7 |
|
|
|
|||
|
|
|
|
|
|
|
|
||||
and |
В |
|
|
|
1 |
|
|
|
2.67 W /(m2 |
0С). |
|
|
1 |
|
|
|
1 |
|
|||||
|
24 |
|
|
|
|
|
|
||||
|
|
|
|
|
|
8.7 |
|
|
|
||
|
|
|
4.23 |
|
|
|
12

Issue № 4 (52), 2021 |
ISSN 2542-0526 |
2.The overlap over the cold underground consists of:
grooved floorboards 40 mmthick. Heat transfer resistance will beR = 0.222 m2 0C/W, the heat absorption coefficient of wooden floorboards (wood) will be s12 = 5.98 W/m2 0C, thermal inertia D = Rs = 0.222 · 5.98 = 1.32;
mineral wool slabs made of stone fiber 50 mm thick, density γ = 40 kg / m3, thermal resistance R = 1.11 m2 0С / W. The coefficient of heat assimilation of the insulation s is determined for furnace heating of the room once in a day according to the formula (10):
s12 0.0120.045 0.84 1000 40 0.466 W /(m2 0С), thermal inertia of the insula-
tion layer D = Rs = 1.111 · 0.466 = 0.693;
roll from boards with a thickness of 30 mm with a density of γ = 550 kg / m3 on cranial blocks with thermal resistance R = 0.166 m2 0C/W, = 5.98 W/(m2 0C) and thermal inertia D = Rs = 0.166 ∙ 5.98 = 0.693.
The boundary of the layer of sharp fluctuations is in the first layer, therefore, the heat assimilation coefficient is determined by the following formula
Y |
Rs2 |
s |
|
||
1 1 |
|
2 |
, |
(16) |
|
|
|
|
|||
int |
1 R s |
|
|||
|
|
1 |
2 |
|
|
and the heat absorption coefficient according to the formula
В |
|
1 |
|
. |
(17) |
|
|
|
|||
|
1 |
|
1 |
|
|
|
Yint |
int |
|
||
|
|
|
Substituting the values into formulae (16) and (17), we obtain the numerical values of the coefficients of heat absorption and heat absorption of the floor surface above the underground:
|
|
0.222 5.982 0.466 |
|
|
|
2 |
|
0 |
||||
Y |
|
|
|
|
|
|
7.21 |
W |
/(m |
|
|
С), |
|
|
|
|
|
|
|||||||
int |
|
1 0.222 0.466 |
|
|
|
|
|
|
||||
|
|
|
|
|
|
|
|
|||||
and |
|
|
|
|
|
|
|
|
|
|
|
|
|
|
В |
1 |
|
3.95 W /(m2 |
0С). |
|
|||||
|
|
1 |
1 |
|
||||||||
|
|
|
|
|
|
|
|
|
|
|
|
|
|
|
|
|
8.7 |
|
|
|
|
|
|
|
|
|
|
7.21 |
|
|
|
|
|
|
|
By analogy with the previous calculation for a furnace, once a day, we determine the heat absorption coefficients of the inner surface of the floor Yint and the heat absorption coefficient of its inner surface B according to the formula (16) and (17). In this case, the values of the heat assimilation coefficients of wood s24 = 4.23 W / (m2 0С) and mineral wool insulation based on stone fiber s24 = 0.33 W / (m2 0С).
13
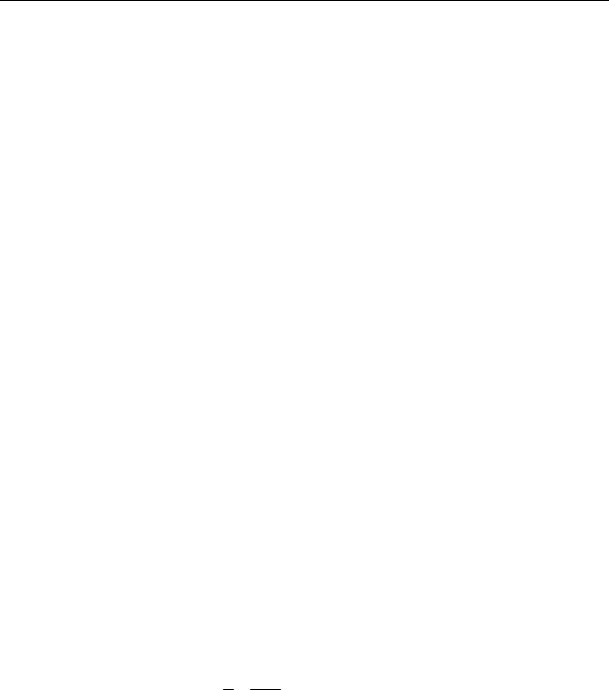
Russian Journal of Building Construction and Architecture
Then
Y |
|
R1s12 s2 |
|
|
0.222 4.232 0.306 |
4.0 W /(m2 0С), |
||||||||
|
|
|
|
|
|
|
|
|||||||
int |
|
1 Rs |
2 |
|
|
|
1 0.222 0.306 |
|
||||||
|
1 |
|
|
|
|
|
|
|
|
|
|
|||
|
В |
|
1 |
|
|
|
1 |
|
2.74 W /(m2 |
0С). |
||||
|
|
|
|
1 |
1 |
|
1 |
|||||||
|
|
1 |
|
|
|
|
|
|
||||||
|
|
|
Yint |
int |
4.0 |
8.7 |
|
|
|
|||||
|
|
|
|
|
|
|
|
|
3.The intermediate floor consists of:
ceiling boards 30 mm thick with density γ = 550 kg / m3 with thermal resistance
R=0.166m2 0С/W,s12 =5.98W/(m2 0С)andthermalinertiaD =Rs=0.166∙5.98=0.693;
clay-sand layer 20 mm thick with density γ = 1800 kg / m3 with thermal conductivity
λ = 0.29 W / (m 0C), c = 0.84 kJ / kg 0C, s12 = 7.94 W / (m2 0C), thermal resistance
R = 0.069 m2 0C / W and D = Rs = 0.069 ∙ 7.94 = 0.547;
wood waste in the form of shavings, chips and sawdust 40 mm thick with density γ = 550 kg / m3 thermal conductivity λ = 0.17 W / m 0С with thermal resistance
R =0.235m2 0С/ W, s_12 =5.98W/ (m2 0С) andthermalinertiaD=Rs=0.235∙ 5.98=1.4;
air gap 60 mm thick with thermal resistance R = 0.2 m2 0C / W and D = 0;
the floor of the first floor made of floorboards and a wooden roll with a thickness of
60 mm with a density of γ = 550 kg / m3 with thermal resistance R = 0.333 m2 0С / W, s12 = 5.98 W / (m2 0С) and thermal inertia D = Rs = 0.333 ∙ 5.98 = 1.93.
The heat transfer coefficient of the floor is K = 0.99 m2 0С / W.
The conditional middle of the overlap, determined by the formula, is located in the layer of wood waste at a distance of 20 mm from the boundary with the air gap. Then, with a layer thickness of wood waste of 20 mm, the thermal resistance will be,
R 0.02 0.117 m2 0C /W .
0.17
For the conditional middle of the overlap, we take s = 0 W / m2 0С and for the surface of wood waste the heat absorption coefficient will be
|
R s2 |
0 |
0.117 5.982 0 |
W /(m2 0С). |
|
||
Y |
2 2 |
|
|
|
4.18 |
(18) |
|
|
|
|
|||||
3 |
1 R 0 |
1 0.117 0 |
|
|
|||
|
|
2 |
|
|
|
|
|
Determine the coefficient of heat assimilation of the inner surface of the second layer of claysand putty
|
R s2 |
Y |
0.069 7.942 4.18 |
W /(m2 0С). |
|
|||
Y |
2 2 |
|
3 |
|
|
6.42 |
(19) |
|
|
|
|
|
|||||
2 |
1 R Y |
1 0.069 4.18 |
|
|
||||
|
|
2 |
3 |
|
|
|
|
|
14

Issue № 4 (52), 2021 |
ISSN 2542-0526 |
Determine the coefficient of heat absorption for the ceiling surface (the first layer)
|
|
R s2 |
Y |
0.166 5.982 6.42 |
W /(m2 0С). |
|
|||
Y Y |
|
1 1 |
|
2 |
|
|
5.99 |
(20) |
|
|
|
|
|
||||||
1 int |
|
1 RY |
1 0.166 6.42 |
|
|
||||
|
|
|
1 |
2 |
|
|
|
|
|
Heat absorption coefficient of the ceiling surface
|
|
1 |
|
1 |
|
|
2 |
0 |
||
В |
|
|
|
|
|
|
|
3.52 W /(m |
|
С). |
1 |
|
1 |
1 |
|
1 |
|
||||
|
Y1 |
int |
|
5.93 |
8.7 |
|
|
|
||
|
|
|
|
|
|
|
4. The internal partition consists ofwooden beams 50 mm thick with a density ofγ = 550 kg / m3 with a thermal resistance R = 0.376 m2 0С / W, s12 = 5.98 W / (m2 0С). Half of its thickness has a thermal resistance R = 0.138 m2 0С / W. For the middle of the partition we take s = 0 W / (m2 0С) and the coefficients of heat absorption and heat absorption of the partition surface are
|
Rs2 |
0 |
0.138 5.992 |
0 |
W /(m2 0С), |
||||||||||||
Y |
1 1 |
|
|
|
|
|
|
|
|
|
|
4.23 |
|||||
|
|
|
|
|
|
|
|
|
|
|
|||||||
int |
1 R 0 |
|
1 0.138 0 |
|
|
|
|
||||||||||
|
|
|
1 |
|
|
|
|
|
|
|
|
|
|
|
|
|
|
and |
|
|
|
|
|
|
|
|
|
|
|
|
|
|
|
|
|
|
|
|
1 |
|
|
|
|
1 |
|
|
|
|
|
2 |
|
0 |
|
В |
|
|
|
|
|
|
|
|
|
3.14 W /(m |
|
|
С). |
||||
1 |
|
1 |
|
1 |
|
1 |
|
|
|||||||||
|
|
Yint |
int |
|
|
|
8.7 |
|
|
|
|
|
|
||||
|
|
|
|
|
4.23 |
|
|
|
|
|
|
The values of the heat loss of the room and the heat absorption of the inner surface, obtained on the basis of the calculations carried out, are presented in tables 1 and 2.
Heat loss and heat assimilation of the surface of the room with a period of z = 12h |
|
Table 1 |
|||||||||
|
|
||||||||||
|
|
|
and the firebox of the room 2 times a day |
|
|
|
|
||||
|
|
|
|
|
|
|
|
|
|
|
|
Fencing |
|
Heat loss of the room |
|
|
Heat absorption of internal surfaces |
||||||
K, |
|
|
tint – text, |
|
|
Yint, |
В, |
Fint, |
|
ВFint, |
|
design |
|
Fint, m2 |
|
Q, W |
|
||||||
|
m2 0C/W |
|
|
0C |
|
|
W/(m2 0C) |
W/(m2 0C) |
m2 |
|
W/ m2 |
Exterior walls |
0.787 |
|
16.5 |
45 |
|
584.35 |
5.98 |
3.32 |
16.5 |
|
54.78 |
Window |
1.82 |
|
6 |
45 |
|
491.4 |
|
1.68 |
6 |
|
10.08 |
Partitions |
7.24 |
|
20 |
|
|
|
4.99 |
3.14 |
20 |
|
62.8 |
Interfloor ceiling |
0.99 |
|
20 |
|
|
|
5.99 |
3.52 |
20 |
|
70.4 |
Floor slab above |
0.67 |
|
20 |
33.75 |
|
452.25 |
7.21 |
3.95 |
20 |
|
79.0 |
the cold underground |
|
|
|
||||||||
|
|
|
|
Σ = |
|
1528 |
|
|
Σ = |
|
277.06 |
|
|
|
|
|
|
|
|
Let us determine the amplitude of fluctuations in the air temperature in the room according to the data in table 1 with a furnace wall thickness of one brick (0.25 m) for a period of z = 12 hours and a two-time firebox per day. To do this, we use a formula of the form
15

Russian Journal of Building Construction and Architecture
А |
0,7mQ |
, |
(21) |
|
|||
t |
ВF |
|
|
|
int |
|
where Q is the average hourly heat transfer of the heating device, equal to the heat losses of the room, W; m is the coefficient of uneven heat transfer for a period of z = 12 hours and with a furnace 2 times a day m = 0.8 and for a period of z = 24 hours and a disposable furnace per day m = 0.7; Σ − heat absorption value of all surfaces of the room, W / 0С.
Heat loss and heat assimilation of the surface of the room with a period of z = 24 h |
|
Table 2 |
||||||||
|
|
|||||||||
|
|
and the firebox of the room once a day |
|
|
|
|
||||
|
|
|
|
|
|
|
|
|
|
|
Fencing structure |
|
Heat loss of the room |
|
|
Heat absorption of internal surfaces |
|||||
K, |
Fint, |
tint – text, |
|
Q, W |
Yint, |
В, |
Fint, |
|
ВFint, |
|
|
m2 0C/W |
m2 |
0C |
|
|
W/(m2 0C) |
W/(m2 0C) |
m2 |
|
W/m2 |
Exterior walls |
0.787 |
16.5 |
45 |
|
584.35 |
4.24 |
2.67 |
16.5 |
|
44.06 |
Window |
1.82 |
6 |
45 |
|
491.4 |
|
1.68 |
6 |
|
10.08 |
Partitions |
7.24 |
20 |
|
|
|
4.0 |
2.74 |
20 |
|
54.8 |
Interfloor ceiling |
0.99 |
20 |
|
|
|
4.0 |
2.74 |
20 |
|
54.8 |
Floor slab over |
0.67 |
20 |
33.75 |
|
452.25 |
4.02 |
2.75 |
20 |
|
55.0 |
cold underground |
|
|
||||||||
|
|
Σ = |
|
1528 |
|
|
Σ = |
|
218.74 |
|
|
|
|
|
|
|
|
For the room under consideration, the amplitude of fluctuations in the temperature of the internal air during the firebox 2 times a day will be
А 0.7 0.8 1528 3.080С .
t |
277.06 |
|
In a similar way, we determine the amplitude of fluctuations in the temperature of the internal air in the room according to the data in table 2 with a period ofz = 24 h and a disposable furnace according tothe formula(10)
А 0.7 0.7 1528 3.420С .
t |
218.74 |
|
2. Experimental research results. Conducted full-scale heat engineering studies in a cottage with wooden walls made of a bar with a cross section of 0.2 × 0.2 m, which occurred at tint = 19.6 °C and text = –18.4 °C, made it possible to obtain the temperature distribution on the outer surface of the wall (Fig. 2). It shows that the temperature of the inner surface in the corner is 11.24 °C, then it rises and at a distance of about 0.4 m reaches 15.9 °C.
In addition, in the course of field observations, temperatures were measured on the outer surface of the wall in the zone of the outer corner. In Fig. 2 it can be seen that in the corner the temperature is (–18.12) 0С, then it gradually rises and on the smooth surface of the outer surface is (–16.8) 0С.
16
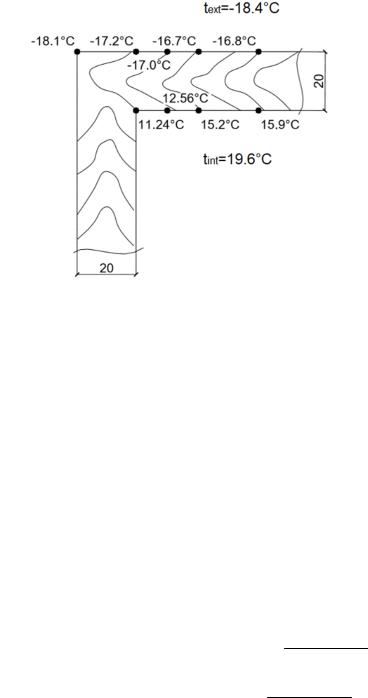
Issue № 4 (52), 2021 |
ISSN 2542-0526 |
To compare the calculated data obtained by the developed technique with the experimental values, we will use the temperature distribution over the outer corner surface and the obtained
At and B values given in tables 1 and 2.
Fig. 2. Temperature distribution over the inner and outer surfaces in the corner of the cobbled wall
Using equation (9), we determine the temperature on the inner surface of the outer corner for the period z = 12 hours (the furnace 2 times a day)
cor |
|
tint text |
|
АtВ |
|
(19.6 18.4) |
|
3.04 3.39 |
0 |
int |
tint |
|
|
|
19.6 |
|
|
|
11.56 C , |
cor R |
cor |
5 1.27 |
5 |
||||||
|
|
int 0 |
|
int |
|
|
|
|
|
and for the period z = 24 hours (firebox once a day)
cor |
|
tint text |
|
Аt В |
|
(19.6 18.4) |
|
3.42 2.67 |
0 |
int |
tint |
|
|
|
19.6 |
|
|
|
11.87 С . |
corR |
cor |
5 1.27 |
5 |
||||||
|
|
int 0 |
|
int |
|
|
|
|
|
Thus, it can be noted that the calculations of the temperature on the inner surface of the outer corner showed the convergence ofthe results obtained according to the formula (9) proposed by the author with the results of experimental studies. So the discrepancy between the temperature determined in the corner by formula (9) and thetemperatureobtained experimentally was:
with a period of z = 12 hoursand a firebox 2 times a day 11.56 11.24 100% 2.84%;
11.24
with a period of z = 24 hoursand a firebox once aday 11.87 11.24 100% 5.6%.
11.24
The obtained values of the discrepancies between the calculated and experimental values are 2.86 % and 5.6 %, respectively, for the periods z = 12 hours and z = 24 hours, which satisfies the accuracy of engineering calculations of the enclosing structures.
17

Russian Journal of Building Construction and Architecture
Conclusions. In conclusion, we can state the following:
1.A new engineering calculation method has been developed that allows one to determine the temperature of the inner surface of the outer corner under non-stationary conditions, taking into account the heat-shielding properties of the wall structure, the amplitude of fluctuations in the temperature of the indoor air in the room, the heat absorption of the inner surfaces of intermediate slabs (floor, ceiling), partitions, windows and the coefficient heat exchange of the inner surface of the outer corner.
2.The analysis of the results of analytical calculations of the temperature on the inner surface of the corner according to the developed method and experimental data showed the convergence of the obtained temperature values according to formula (9) and according to the results of the experiment. The difference in the results is at the level of 2.84% and 5.6%, which allows this calculation method to be used in the design of external building envelopes.
References
1.Bogoslovsky V. N. Stroitel'naya teplofizika (teplofizicheskie osnovy otopleniya, ventilyatsii, konditsionirovaniya vozdukha) [Building thermophysics (thermophysical foundations of heating, ventilation, air conditioning)]. St. Petersburg, AVOK Severo-Zapad Publ., 2006. 400 p.
2.Borodin A. I. Postroenie temperaturnogo polya v sechenii naruzhnogo ugla ograzhdayushchei konstruktsii [Construction of the temperature field in the cross-section of the outer corner of the enclosing structure]. In- zhenerno-fizicheskii zhurnal, 2006, no. 5, vol. 81, pp. 971––976.
3.Borodin A. I. Opredelenie temperaturyna vnutrennei poverkhnosti vuglu naruzhnoi steny[Determination of temperature on the inner surface at the corner of the outer wall]. Izvestiya vysshikh uchebnykh zavedenii. Stroitel'stvo, 2007, no. 12 (588), pp.76–79.
4.Borodin A. I., Cherepanov Z. B. Problema obrazovaniya kondensata v naruzhnykh uglakh zdanii v svete normativnykh trebovanii [The problem of condensation formation in the outer corners of buildings in the light of regulatory requirements]. Vestnik Tomskogo gosudarstvennogo arkhitekturno-stroitel'nogo universiteta, 2009, no. 1, pp. 142––147.
5.Vlasov O. E. Osnovy stroitel'noi teplotekhniki [Fundamentals of building heat engineering]. Moscow, VIA RKKA Publ., 1938. 95 p.
6.Gagarin V. G., Kozlov V. V. Teoreticheskie predposylki rascheta privedennogo soprotivleniya teploperedache ograzhdayushchikh konstruktsii [Theoretical prerequisites for calculating the reduced resistance to heat transfer of enclosing structures]. Stroitel'nye materialy, 2010, no. 12, pp. 4––8.
7.Danilov N. D., Shadrin V.Yu. Prognozirovanie temperaturnogo rezhima uglovykh soedinenii naruzhnykh ograzhdayushchikh konstruktsii [Prediction of the temperature regime of corner joints of external enclosing structures]. Promyshlennoe i grazhdanskoe stroitel'stvo, 2010, no. 4, pp. 20––22.
8. Kozloborodov A. N., Tsvetkova D. N. Prostranstvennyi teploperenos v stene maloetazhnogo zdaniya iz profilirovannogo uteplennogo brusa [Spatial heat transfer in the wall of a low-rise building made of profiled insulated timber]. Vestnik Tomskogo gosudarstvennogo arkhitekturno-stroitel'nogo universiteta, 2013, no. 3,
pp. 298––307.
9.Kozloborodov A. N., Ivanova E. A. Analiz sovmestnogo vliyaniya neskol'kikh teplonapryazhennykh elementov na teplovoe sostoyanie stroitel'noi konstruktsii [Analysis of the joint influence of several heatstressed elements on the thermal state of a structure]. Vestnik Tomskogo gosudarstvennogo arkhitekturnostroitel'nogo universiteta, 2016, no. 1, pp. 133––139.
10.Kozloborodov A. N., Ivanova E. A. Issledovanie vliyaniya nagrevatel'nogo kabelya na teplovoe sostoyanie ograzhdayushchei konstruktsii v oblasti naruzhnogo ugla [Investigation of the influence of the heating cable on the thermal state of the enclosing structure in the area of the outer corner]. Vestnik Tomskogo gosudarstvennogo arkhitekturno-stroitel'nogo universiteta, 2019, no. 21 (5), pp. 127––137.
18
Issue № 4 (52), 2021 |
ISSN 2542-0526 |
11.Kornienko S. V. Otsenka vliyaniya kraevykh zon ograzhdayushchikh konstruktsii na teplozashchitu i energoeffektivnost' zdanii [Assessment of the influence of the edge zones of enclosing structures on thermal protection and energyefficiency of buildings]. Inzhenerno-stroitel'nyi zhurnal, 2011, no. 8, pp. 22––29.
12.Kornienko S. V. Teplotekhnicheskii raschet stroitel'nykh konstruktsii s kraevymi zonami [Heat engineering calculation of structures with marginal zones]. Vestnik Volgogradskogo arkhitekturno-stroitel'nogo universiteta. Stroitel'stvo i arkhitektura, 2013, no. 34 (53), pp. 22––29.
13.Machinsky V. D. Teploperedacha v stroitel'stve [Heat transfer in construction]. Moscow, Gosstroiizdat Publ., 1939. 343 p.
14.Nazirov R. A., Podkovyrin V. S., Podkovyrina K. A. Opredelenie temperatury vnutrennikh poverkhnostei v naruzhnykh uglakh zdanii [Determination of the temperature of internal surfaces in the outer corners of buildings]. Izvestiya vysshikh uchebnykh zavedenii. Ser. Stroitel'stvo, 2016, no. 10-11, pp. 106––111.
15.Semenov L. A. Teplootdacha otopitel'nykh pechei i raschet pechnogo otopleniya [Heat transfer of heating stoves and calculation of stove heating]. Moscow, Stroiizdat Narkomstroya Publ., 1943. 81 p.
16.Shil'd E., Kassel'man Kh. F., Damen G., Polenz R. Stroitel'naya fizika [Building physics]. Moscow, Stroiizdat Publ., 1982. 296 p.
17.Fokin K. F. Stroitel'naya teplotekhnika ograzhdayushchikh chastei zdanii [Building heat engineering of enclosing parts of buildings]. Moscow, AVOK-PRESS Publ., 2006. 256 p.
18.Tolstova Yu. I. Kharitonova T. Teplopoteri ostrykh uglov zdanii [Heat loss of acute corners of buildings].
Santekhnika, otoplenie, konditsionirovanie, 2011, no. 8 (16), pp. 52––53.
19.Beattie K., Tang D. Analysis of heat transfer from corner structure of building. Transactions on Engineering Sciences, WIT Press, 1998, vol. 20.
20.Jeong Y. S. The heat transfer simulation for thermal bridge effect of the corner walls of building according to thermal condition. Proceedings Building Simulation, 2007, pp. 169––174.
21.Oreszczyn T. Cold bridging at corners: Surface temperature and condensation risk. Building Serv. Eng. Res. Technol., 1988, no. 9 (4), pp. 167––175.
22.Simoes N., Joana Prata J., Tadeu A. 3D dynamic simulation of heat conditions through a building corner using BEM Model in the FrequencyDomain. Energy, 2019, no. 12, pp. 4595. doi: 10.3390 / en12234595.
23.Tang D. Temperature distribution and heat transfer through a homogeneous corner. Building and Environment, 1997, vol. 32, no. 5, pp. 457––463.
24.Tang D., Beattie K. Calculation of heat transfer from corners buildings. Proc. CIBSE A: Building Serv. Eng. Res. Technol., 2000, no. 21 (4), pp. 249––255.
19