
brechignac_f_desmet_g_eds_equidosimetry_ecological_standardi
.pdf6
where the labels of equations (1, 2, 3) are used. For a specific freshwater water body, where S=2 km2, H=4 m, Kb=1000, F=0.7, the maximum permissible release of radionuclides is Nb<17.1 TBq in the water of the whole water body. At the same time the maximum permissible release of radionuclides into a water body for its benthos was estimated using equation (6) Nk< 0.18 TBq. This magnitude is 70 times less than the permissible release of radionuclides, which was assessed for the population of the water column of a water body.
In general , the ratio of assessments of maximum permissible releases of radionuclides into a water body is determined by the following equation through two critical links (population of water column and benthos):
N k |
|
hKb 1 F |
(8) |
Nb |
|
HkF |
|
|
|
3. Similar evaluations of maximum permissible releases of radionuclides can be carried out also for other types of ecosystems. Particularly, in a system of cascades of reservoirs (such as the Dnieper cascade), the first reservoir (the Kiev reservoir) is critical for the dose commitment. In bottom sediments of the upper part of Kiev reservoir, the levels of radionuclide content in bottom sediments reach about 370 kBq kg-1 and more. In fact, this means that at the upper part of the reservoir the level of effective release of radionuclides reaches the maximum permissible value. In the population of benthos, it is therefore possible to expect noticeable ecological consequences. The theoretical calculated maximum permissible release of radionuclides in the Kiev reservoir is estimated to be a total of 83 TBq, while the real content of 137Cs in bottom sediments is estimated on location measurements at a 200 TBq. This significantly exceeds the maximum permissible concentration >7 .
4. In marine ecosystems, the bioproductivity develops mainly in shallow coastal water. On an average concentration 10 g m-3 of biota in water forms, the radiocapacity reaches 0.9-0.99. In that case, the release of large amounts of water with radionuclide content of 1.2-12 kBq l-1 can result in radionuclide contamination of the community up to 115 kBq kg-1. This is higher than an ecological permissible level.
3.3.ASSESSMENT OF MAXIMUM PERMISSIBLE RELEASES AND DISPOSAL OF RADIONUCLIDES IN SLOPE ECOSYSTEMS
The developed prime model of an evaluation of slope ecosystem radiocapacity permits assessment of dynamics, time and place of expected concentration of radionuclides in some elements of the slope ecosystem. The factor of radiocapacity of the slope ecosystem may be assessed by the equation (5).
Where, Pi is the probability of a flow of radionuclides from an appropriate element of the slope ecosystem in a year. P1 = flow from forest ecosystem. P2 = flow from stony area. P3 = flow from meadow ecosystem, P4 = from terrace in a lake.
As an example of a slope ecosystem, the simplest version: Forest Stony areaMeadow Terrace Lake was selected. The computing curves in dynamics of long-lived radionuclides redistribution in such a model of ecosystem are represented in

7
Figure1. The initial contamination of the forest was 3.7 TBq. (For the sake of simplicity radioactive decay was not taken into consideration).
PERSENTAGE,%
1 0 0 |
|
|
|
|
|
9 0 |
|
|
|
|
|
|
|
|
|
|
|
8 0 |
|
|
|
|
1 - F O R E S T |
|
|
|
|
2 - S T O N Y P L O T |
|
|
|
|
|
||
|
|
|
|
|
3 - M E A D O W |
|
|
|
|
|
|
|
|
|
|
|
4 - T E R R A C E |
|
|
|
|
|
|
7 0 |
|
|
|
|
5 - L A K E |
|
|
|
|
||
6 0 |
|
|
|
|
|
5 0
4 0 |
|
|
|
|
|
|
|
|
|
|
|
|
|
|
|
|
|
|
|
|
3 0 |
|
|
|
|
|
|
|
|
|
|
|
|
|
|
|
|
|
|
|
|
2 0 |
|
|
|
|
|
|
|
|
|
|
|
|
|
|
|
|
|
|
|
|
1 0 |
|
|
|
|
|
|
|
|
|
|
|
|
|
|
|
|
|
|
|
|
0 |
|
|
|
|
|
|
|
|
|
|
|
|
|
|
|
|
|
|
|
|
1 |
2 |
3 |
4 |
5 |
6 |
7 |
8 |
9 |
1 0 |
1 1 |
1 2 |
1 3 |
1 4 |
1 5 |
1 6 |
1 7 |
1 8 |
1 9 |
2 0 |
2 1 |
|
|
|
|
|
Y E A R S |
|
A F T E R |
C H E R N O B Y L |
A C C I D E N T |
|
|
|
|
|||||||
|
|
|
|
|
|
|
|
|
F i g . |
1 |
|
|
|
|
|
|
|
|
|
Fig. 1. Radiocapacity of Slope Ecosystems.
It is visible precisely, that the permissible levels of radionuclide contamination decrease noticeably in accordance with the approach of the place of contamination to a lake. Strictly speaking, the ecological norm depends on the ecosystem character. The closer to the critical unit (lake) a subsystem is situated and the higher the value of probability of drain, the lower the ecological specification of permissible contamination.
4. Existing available approaches to evaluating of the effect of the various toxic factors on the condition of ecosystems
Let us consider the basic concept of our approach for an evaluation of the effect of the toxic factors (radiation, chemical, biological and other pollutants) on of ecosystem biota. This goes about a comparison of effects of the different factors, through a modification of the parameters of radiocapacity.
Let the system consist at all of two blocks: an environment (water, for example) and biota. Then the chamber model looks as follows:
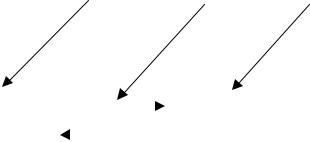
8
INTERNAL FACTORS (POLLUTANTS)
|
|
a 12 |
||
ENVIRONMEMT |
|
|
|
BIOTA |
(WATER) |
|
|
|
|
|
|
|
|
|
|
|
|
|
|
a 21
Fig. 2. Box-model of simplest of ecosystem.
Biota in ecosystem can be one-way, two-specific, and multispecific. Ecosystems can be model systems (maize, Lemnaceae etc.) or actual natural ecosystems. In order to evaluate the influence of the different factors of an external medium on biota some strategies are used:
1. The main strategy is an evaluation of the condition of biota. For this purpose, in the literature, up to 30 different parameters (variety of type of ecosystems, number at use, the biomass of the different ecosystem types, velocity of a duplication etc.)(8). This evaluation strategy of the condition of biota as response on contamination by a multitude of factors is used widely among ecologists. This strategy differs by this property, that the measurable results of toxic effects (depressing of kinds, loss of kinds, succession etc.) are observed in ecosystems after significant times after the start of the impacting factors. Herewith is dealt with the remote visible results of an action of contaminants on biota. Thus in case such an approach is needed to evaluate effects on biota, there is a possibility to determine already existing effects, which as a rule, are irreversible and result in depressing and - or losses biota or part of it. This approach allows only to stating an eventual result of influence of pollutants on biota.
2. The second method of evaluating the influence of the toxic factors on biota in ecosystems is based on the right choice of a highly sensitive biological test - object, which is capable to react noticeably and sharply in a medium of toxic substances. Such type by indicators of a toxic effect can be among the different types of plants, animal (mouse) and fish (gold fishes) etc. It is a highly sensitive method for testing toxicity of different pollutants. It is also known that the type of indicator can be highly sensitive to the of one pollutant and not to react on an other pollutant. On the one hand, this method allows to show a probable toxic effect at a low level for one factor, and on the other hand be not suitable for testing of other factors. A whole system of different types of indicators is required to assess the problem.
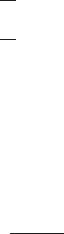
9
3. A third approach is also possible; it is to evaluate the interaction of biota with their environment. This comes to finding a method for evaluating of rates of exchanges between a medium and biota, this means the rate of absorption (nutritious substances for example) and rate of outflow of substances from biota into the environment.
The approach is based on the known fact that any biologically significant effect on biota, finds its reflection in a modification of the parameters of absorption and outflow of substances between an environment and biota..
The worse the condition , it is the more possible to suppose, proceeding from the block diagram, that the toxic effect is necessarily exhibited through a modification of parameters a12 and a21, or both simultaneously. The worse the condition of biota after an external exposure, the more this effect should reduce parameter a12 and - or increase the parameter of outflow - a21.. Especially noticeable modifications can come about on the magnitude of the relation of parameters -a12/ a21.
Let for an example a simple model system be considered: a hydroponics plant culture, where some radioactive tracer, for example Cs-137 is added. With this tracer, the redistribution (absorption and - or outflow) should reflect a condition of biota. The parameters of the relation of biota with their environment (in this case with water) show the balance between the input of nutrients to biota (a12) and their outflow to their environment - a21. In this ecosystem model - the system of differential equations, in a boxmodel, is represented by: (MAPLE 6):
ω
dif1 := ωx y(x) a21 z(x) a12 y(x)
ω
dif2 := ωx z(x ) a12 y(x) a21 z(x)
(9)
inicond := y(0 ) 1, z(0 ) 0
Where y (x)- is the contents of the tracer Cs-137 in water, and z(x)) the content of tracer in biota of the model ecosystem. Here at the entry conditions–at the initial time (Ȥ = 0) the total content of the tracer is in the water, and the content in the biota is equal = 0. The solution of this set of equations is represented below (10):
|
|
|
|
|
|
|
|
|
a12e( (a12 a21) x) a21 |
|
(10) |
||
|
a21 |
|
( (a12 a21) x) |
|
|
|
a12a21 |
||||||
|
|
|
|
|
|
|
|||||||
|
|
a12e |
|
|
|
|
a12 a21 |
|
a12 a21 |
||||
|
|
|
|
, z(x) |
|
|
|
|
|
|
|||
y(x) |
a12 |
|
a12 |
|
|
a21 |
|
|
|
|
|||
|
a21 |
a21 |
|
|
|
|
|
|
|
Using the above, the size of parameters a12 and a21, deducted from the experiment, it is not difficult to constructing the graph of the dynamics of distribution of tracer in the boxes:
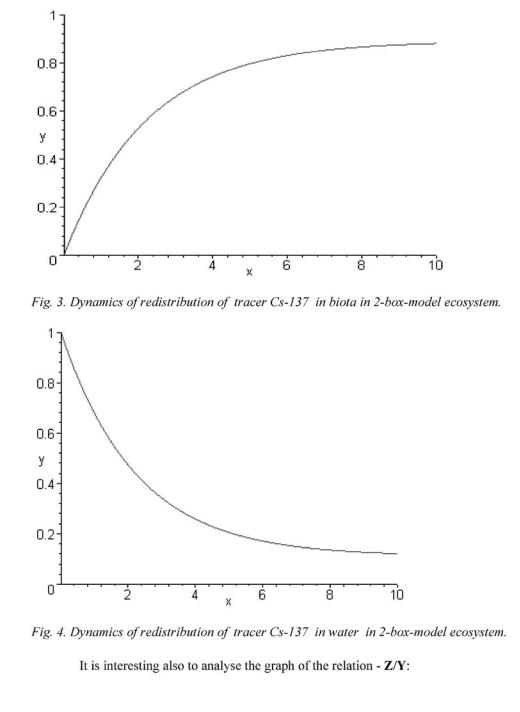
10

11
The outcome of the modelling allows to evaluating a number of the important parameters of the model ecosystem, for long time observation time ranges. In particular, the magnitude of the radiocapacity of biota and water, is determined as the magnitude of the contribution of the tracer radionuclides, which remains in water or biota.
From the equations (9,10 ) it is possible to see that for a range of x ο φ, the magnitudes of radiocapacity can be determined by the following formulas:
F w = a21 / a21 + a12 ; |
F b = a12 / a21 + a12; |
And their relation by: |
|
F b / F w = a21 / a21 |
(11) |
It is not difficult to show, that in the given simple case, the factor of tracer (Cs137accumulation by biota ) Tf # a12 / a21 + a12. Thus Tf - Fb - factor of radiocapacity of the biota model ecosystem is proportional.
Thus, if the external toxic effect on biota of ecosystems is important, it will be reflected in a diminution of exchange rate parameters between boxes (biota - environment) Then a modification of magnitude of the factors of a radiocapacity F b and F w, will entail, and as a corollary the sizes Tf to biota which are directly connected with parameters of a radiocapacity will be changed y. The most sensitive measure of a modification of the condition of a biota ecosystem, in response to external factors
12
(chemical, natural and other nature) could be the relation of the radiocapacity factors F b / F w = a21 / a21 . This relation can be an important adequate measure of well being of biota ecosystem.
5. Assessment of parameters of radiocapacity as of indexes of stability and reliability of ecosystems
5.1. INTRODUCTION
Model calculations have shown, that essential effects on ecosystems are accompanied by convertible or irreversible changes of radiocapacity parameters of biotic components of the ecosystem. It depends on the intensity and duration of effect.
Changes in the radiocapacity factors for Cs137 under influence of external gamma-irradiations were detected in preliminary experiments on hydroponics’ crop cultivation. It was manifest by a decreasing the radiocapacity factor of the plants rooted system in response to a stress-factors.
Therefore, the radiocapacity factors can be used as parameters for the condition and well-being of an ecosystem under various chemical, physical and biological influences.
The theory and the models of ecosystems radiocapacity have allowed to formulate and to define the approaches to the substantiation of ecological standards on permissible levels of ecosystems and the contamination of their components, and also on permissible releases of radionuclides >4 .
The present hypothesis consists of the following elements: we presume that some rather few long-lived radionuclides have contaminated an ecosystem in its steady;. the radionuclides are arranged in compartments of the ecosystem and are described by appropriate values of the radiocapacity factors; the external harmful factors (toxic, radiation and other nature) act on the ecosystem. If the effect is essential, it may affect the ecosystem and its parameters. It will bring about sharp changes of the values of the radiocapacity factors of the ecosystem components [9, 10].
We assume that the noticeable radionuclide redistribution has taken place in an ecosystem with known initial distribution of radionuclide-tracer (or tracers). It signifies that values of radiocapacity factors have changed ( thence values of ecosystem radiocapacity). A predicting evaluation of an ecosystem condition, its stability and reliability is supposedly to be made at measurable changes of radiocapacity parameters. The predicting evaluation can be conducted long before a response on effect of integral parameters (variety of species, biomass, number and rate of reproduction of species in the ecosystem) is noticed.
5.2. MODELLING OF CHANGE OF RADIOCAPACITY PARAMETERS OF HARMFUL EFFECTS IN THE ELEMENTARY ONE-SPECIES SYSTEM
As an initial model we shall consider a simple fresh-water ecosystem such as a reservoir (lake) with one species of algae, representing the phytoplankton, and determining bioproductivity of the lake. Parameters of the reservoir are: V (volume) =1010; S(surface) = 2 km2; H (depth) = 5 m; concentration of algae Υ=10 g m-3, transfer
13
factor (Tf) of algae Kb=104. In the first moment after a peak release in the reservoir of 3.7*1010 Bq 137Cs (in summer period) the factor of radiocapacity of bottom sediments will become 0.7 (k=103, h = 0.1 m, and factor of radiocapacity of a water after release = 0.3 (1)). It is known, that the redistribution of radionuclides between water and bottom sediments (1) in such reservoir will take place very quickly, practically in the course of number of days >7 . Thus pursuing to our approaches the radiocapacity factors of the components are estimated by the formulas (12):
|
|
|
kh |
||||
°Fbs |
|
|
|
|
|
|
|
|
|
kh H HΥKb |
|||||
° |
|
|
|||||
° |
|
|
H |
||||
®Fw |
|
|
|
(12) |
|||
|
|
||||||
° |
|
kh H HΥKb |
|||||
° |
|
|
HΥKb |
||||
°Fb |
|
|
|
|
|
|
|
kh H HΥKb |
|||||||
¯ |
An estimation can now be made of the parameters of the considered reservoir coming to : Fbs=100/605= 0.165; Fw = 5/605 = 0.008; Fb =500/605= 0.826.
By definition the total radiocapacity factor of a reservoir is equal to 1.
|
F |
Fbs Fw Fb |
1 |
|
(13) |
||||
Situation changes resulting from stress-effect: |
|
|
|||||||
|
dF |
|
dFbs |
|
dFw |
|
dFb |
0 |
(14) |
|
dt |
dt |
dt |
dt |
|||||
|
|
|
|
|
|
Obviously the stress-factor impacts on biota. However, as a rule, there is no direct influence to water and bottom sediments of such stress-factors . Then it is possible to observe a noticeable decrease of Fb, and at the expense of it an increase of remaining values.
Then:
If |
|
dFb |
¢0 , and |
dFw |
|
|
dFbs |
²0 |
|||||
|
dt |
dt |
dt |
||||||||||
|
|
|
|
|
|
|
|
|
|||||
|
dF |
§dF |
|
dF |
· |
|
|||||||
|
|
|
b |
|
¨ |
w |
|
|
|
bs |
|
¸ |
(15) |
|
|
dt |
dt |
dt |
|||||||||
|
|
© |
|
¹ |
|
14
Now we revolve to the formula (12) and take a derivative from Fb:
|
§ |
§ d (ΥK |
b |
)·· |
|
|
|||
dFb |
¨ |
kh H H¨ |
|
|
¸¸ |
|
|
||
dt |
|
|
|
|
|||||
¨ |
© |
|
¹ |
¸ |
¢0 |
(16) |
|||
dt |
|
|
|
|
|||||
¨ 2(kh h ΥKb h) ¸ |
|
|
|||||||
|
¨ |
|
|
|
¸ |
|
|
||
|
© |
|
|
|
¹ |
|
|
It is right to assume, that in initial moment of stress-effect the integral index of a species biomass density will not yet has time to react. Change of Fb will happen at the expense of change (decreasing) Kb (factor of accumulation of biota).Because of stresseffect in ecosystem Fb shows a 10 times decrease and comes to 103. Consequently the structure of the radiocapacity factors of ecosystem elements will vary and will come to : (In parentheses the values before change)
Fb = 0.32 (0.85), Fbs = 0.67 (0.165 ), Fw = 0.03 (0.008).
It should be recalled that at a rather small change of Fb (coefficient of biota accumulation) the radiocapacity factors of ecosystem elements changes essentially (3-5 times). The ecosystem, through change of the factors of radiocapacity (factors of accumulation), witnesses already to significant ecological events when is not yet marked by essential changes of integral indexes (for example, density of a population).
It is possible to generalize the considered above versions of responses of the ecosystems radiocapacity factors to the case of multispecies ecosystems. Such process is essentially capable to change the initial tracer distribution on the ecosystem species, and so to change the values Fi for various biota species. In multispecies ecosystem a predictable change of the radiocapacity factors may take place much more sharply and more appreciably, alongside the fact that changes in values of the radiocapacity factors at separate species can be small.
5.3. EXPERIMENTAL INVESTIGATION.
There are not many experimental data on the influence of the external factors on parameters of ecosystem radiocapacity. Some data will be given.
The researches were carried out on experimental models, such as hydroponics’ cultures of peas and maize.
Half litre glass vessels containing growing plants (4-5 twenty-four hours) were irradiated by external gamma-irradiation at doses of 1-2 Gy. It was shown, that this dose provokes depressing of growth of the main root and additional roots. Thus it is possible to observe decrease of volume of a rooted system and absorption surface. A small amount of 137Cs (1000 Bq l-1) is introduced into these culture vessels . It has been found for this experimental model that exactly the volume and surface of a rooted system determines the accumulation of the radionuclide in plants, and determines the factor of biota radiocapacity.
It was shown in the experiments (Fig 6,7), that the external gamma-irradiation provokes a noticeable decrease of the accumulation of the tracer (137Cs) in rooted and over ground biomass of plants during growth. It turns out that the larger the dose, the
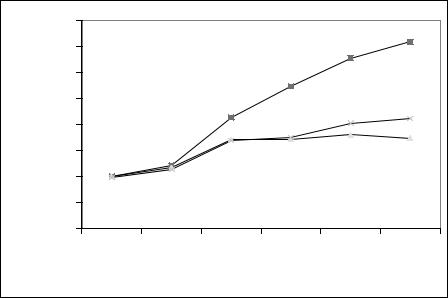
15
smaller is the biota radiocapacity factor in this experimental model. A precise relation between the degree of decline of biota and its radiocapacity factor was established.
These researches have shown, that similar effects are reached at adding heavy metals (for example Cd) in salt solutions(Figs 6,7).
Prolongation of researches in this direction will supposedly allow extending these ideas and approaches to the most various experimental models. Study of the action of the physical, chemical and biological factors on parameters of biota radiocapacity and comparison of a level of changes of radiocapacity with an action of external gamma-irradiation doses will allow to enter an independent method of ecological dosimetry of the indicated factors.
(mm) |
160 |
|
|
|
|
|
|
140 |
|
|
|
|
|
|
|
120 |
|
|
|
|
|
|
|
Plants |
|
|
|
|
|
|
|
100 |
|
|
|
|
|
|
|
80 |
|
|
|
|
|
|
|
of Root |
|
|
|
|
|
|
|
60 |
|
|
|
|
|
|
|
40 |
|
|
|
|
|
|
|
ine |
|
|
|
|
|
|
|
20 |
|
|
|
|
|
|
|
|
0 |
|
|
|
|
|
|
|
1 |
2 |
|
3 |
4 |
5 |
6 |
|
|
Time of |
ro |
th of plants (days) |
|
Fig 6. Dynamics of Growth of Root Plants in Control ( ͏), under action of Cd ( X) and after action of gamma-irradiation (ǻ)