
- •Protein phosphorylation as a switch in cellular functioning
- •Cyclic AMP and the amplification of signals
- •Protein kinase A
- •Protein kinase A and the regulation of transcription
- •Activation of the CREB transcription factor
- •Attenuation of the cAMP response elements by dephosphorylation
- •Protein kinase A and the activation of ERK
- •Actions of cAMP not mediated by PKA
- •Regulation of ion channels by cyclic nucleotides
- •Epac, a guanine nucleotide exchange factor directly activated by cAMP
- •Protein kinase C
- •Discovery of a phosphorylating activity independent cAMP
- •The protein kinase C family
- •Structural domains and activation of protein kinase C
- •The C1–C4 regions
- •Activation of protein kinase C
- •Multiple sources of diacylglycerol and other lipids activate protein kinase C
- •Differential localization of PKC isoforms
- •Different types of PKC-binding proteins
- •Holding back the PKC response
- •A matter of life or death: PKC signalling complexes in the evasion of the fly-swat
- •Phorbol ester and inflammation
- •References
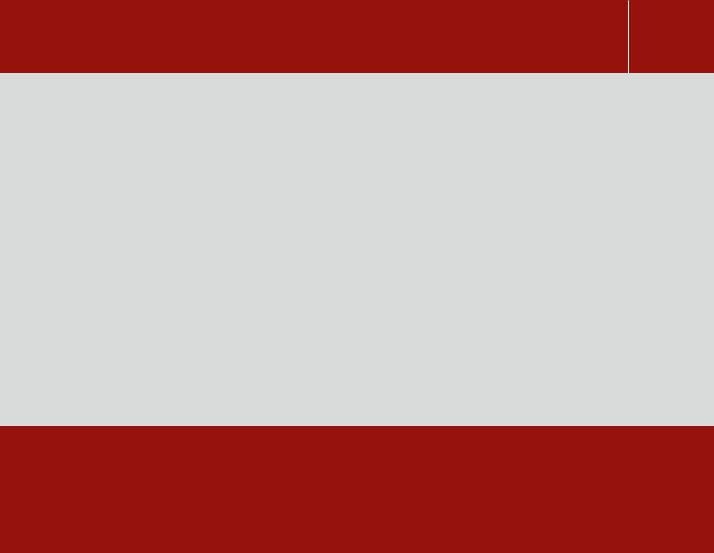
Chapter 9
Phosphorylation and dephosphorylation: protein kinases A and C
Protein phosphorylation as a switch in cellular functioning
The first indications that protein phosphorylation might be an important regulator of enzyme activities emerged at a time when the regulatory
roles of non-covalent interactions of various substances (substrates, cofactors, end products, etc.) were already well established. In particular, the allosteric influence of end products, interacting with regulatory subunits
of multisubunit enzymes provides feedback control over metabolic pathways comprising many steps. The regulation of the activation state of phosphorylase by 5 -AMP (positive feedback) and glucose-6-phosphate (negative feedback) is a pertinent example. Although neither of these metabolites takes part in the reaction catalysed by phosphorylase, the
243
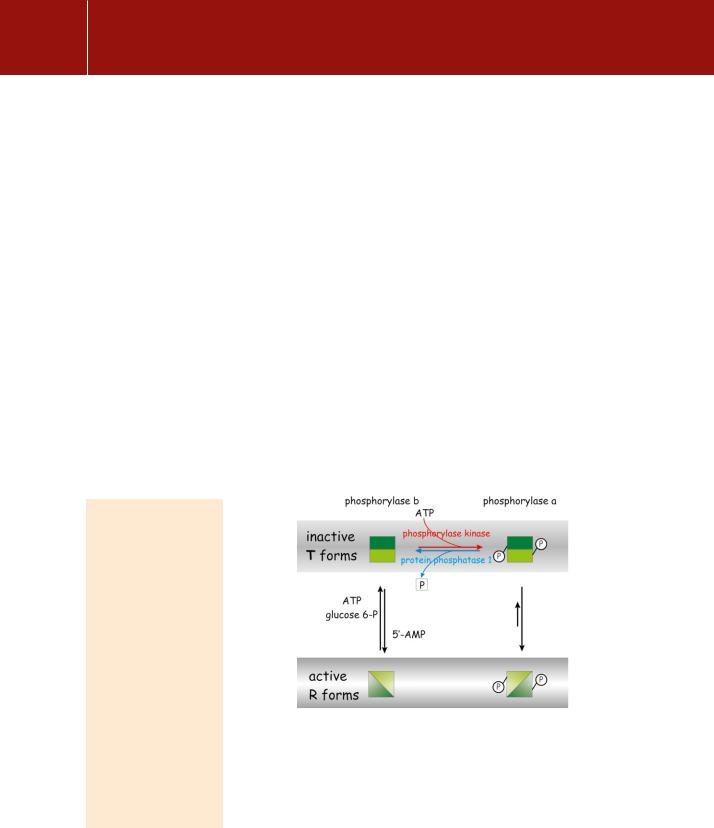
Signal Transduction
The mammalian kinases fall into seven main groups or classes:
AGC: includes PKA, PKG, and PKC families (this chapter)
CAMK: includes Ca2 / calmodulin-dependent protein kinase (Chapter 8)
CK: casein kinase 1 (Chapter 14)
CMGC: containing CDK, MAPK, GSK3, CLK families (Chapters 12, 14, and 18)
STE: homologues of yeast Sterile 7, Sterile 11, Sterile 20 kinases (Chapter 12)
TK: tyrosine kinase (Chapter 12)
TKL: tyrosine kinase-like.
presence of glucose-6-phosphate signals metabolic sufficiency, while 5 -AMP signals hunger. Both can be described as allosteric effectors, interacting
with the enzyme at sites remote from its catalytic centre. Then it emerged that the addition of a single phosphate group to phosphorylase b also switches the enzyme between inactive and active states1 (Figure 9.1). Thus, in addition to allosteric influences, covalent modification by phosphorylation (or dephosphorylation) also affects enzyme activity. Although these two regulatory processes operate through similar conformational changes, phosphorylation is primarily a response to extracellular influences, expressed through hormone receptors, while the allosteric effectors allow the system to respond to intracellular conditions.
The importance of phosphorylation is underlined by the fact that the genome of the budding yeast Saccharomyces pombe specifies more than 120 different protein kinases.3 And this is only yeast! The human genome specifies 518. One in three cytoplasmic proteins contains covalently bound phosphate. Phosphorylation modifies proteins by the addition of negatively charged groups to serines, threonines, and less commonly, tyrosines. These neutral hydroxyamino acid residues are often exposed on surfaces and in the interfaces between the subunits of regulatory proteins. Phosphorylation of a protein can alter its properties substantially. It can result in the recognition, binding, activation, deactivation, phosphorylation, or dephosphorylation of its substrate. Phosphorylation of an enzyme can switch it on or off.
FIG 9.1 Regulation of phosphorylase through phosphorylation and by the action of allosteric effectors. Catalytically inactive (T) and active (R) conformations of the enzyme. When phosphorylase is phosphorylated (phosphorylase a), its ‘relaxed’ R form is favoured. Phosphorylase b adopts a ‘tense’ T conformation except under conditions of starvation, signalled by the presence of 5 -AMP, when it adopts its active R form. The activity of phosphorylase is mainly determined by its phosphorylation status. The terms tense and relaxed refer to the alternative quaternary structures of allosteric enzymes.2 This terminology was originally applied in the analysis of oxygen binding to haemoglobin.
244
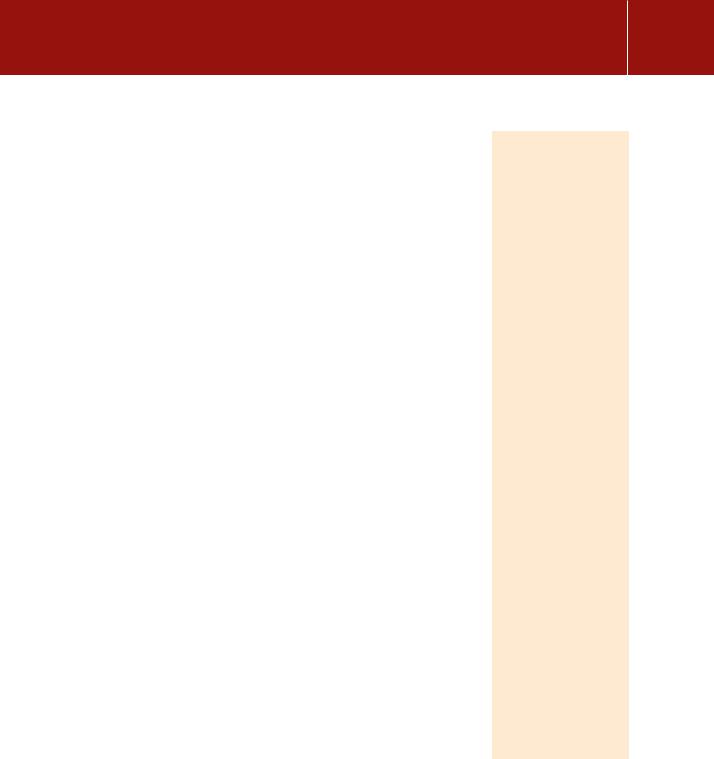
Phosphorylation and Dephosphorylation: Protein Kinases A and C
Things can go badly wrong when the balance of phosphorylation and dephosphorylation is disturbed. Examples are the explosive diarrhoea and hepatotoxicity caused by the inhibition of protein phosphatases by toxins present in infected shellfish (okadaic acid) and in cyanobacteria (microcystins). The reverse is even more grim: for example, the consequences of uncontrolled stripping of phosphate groups which occurs in cells affected by the virulence factor of Yersinia pestis (the agent of bubonic plague, still a major killer). This factor is a protein tyrosine phosphatase.4 The prospects are just as bad when tyrosine kinases become constitutively activated (oncogenic mutations, Chapters 11 and 12). On a happier note, however, a number of important therapeutic procedures (tumour suppression, immunosuppression, and treatment of inflammation) are based on drugs that either promote or suppress phosphorylation reactions. For example, ciclosporin A, used as an immunosuppressive agent, interacts with calcineurin, a subunit of which is protein phosphatase 2B (see page 230).
Cyclic AMP and the amplification of signals
Most but not all of the signals conveyed by cAMP are mediated through phosphorylation reactions catalysed by protein kinase A (PKA). For example, following stimulation of -adrenergic receptors on liver cells by adrenaline, at concentrations around 10 10 mol L 1, the intracellular concentration of cAMP increases to about 1 mol L 1. As a rule of thumb, such ‘typical’ receptors are present at a density of 105 per cell and, in general, only a small proportion of these need to be occupied or activated to maximize the response
(see page 32). This small signal can activate a great deal more of the enzyme glycogen phosphorylase (100 mol L 1) and this can liberate truly enormous quantities of glucose into the bloodstream.
Intermediate amplification of the signal provided by cAMP is provided through two rounds of protein phosphorylation. The first of these is catalysed by the cAMP-dependent protein kinase (PKA). This phosphorylates and activates phosphorylase kinase, which in turn phosphorylates and activates glycogen phosphorylase (Figure 9.2). During exercise or under conditions of starvation, the elevated level of 5 -AMP acts as an allosteric signal for the activation of
phosphorylase b, regardless of its state of phosphorylation. Thus, phosphorylation of the enzyme and its activated state are not synonymous. However, it is fair to say that phosphorylase b is generally inactive and that phosphorylase a is generally active. On the other hand, if there is no need to break down glycogen, then
why do so? Under resting conditions, the catalytic activity of phosphorylase b is suppressed by the presence of glucose-6-phosphate and ATP (which competes at the binding site for 5 -AMP) (see Figure 9.1). In effect, an elevated level of 5 -
Okadaic acid, named from the marine sponge
Halichondria okadai, from which it was fist isolated, is actually the product of a symbiotic dinoflagellate. It accumulates in bivalves and causes diarrheoretic shellfish poisoning.
Its toxicity, expressed
as LD50, in p388 and Ll1210 cells is 17 and
1.7 nmol L 1 respectively. It is a potent inhibitor
of serine/threonine phosphatases, having a Ki of 30 pmol L 1 against protein phosphatase 2A.
The microcystins are a group of cyclic heptapeptides. The
peptide ring is made up of two protein amino acids and five nonprotein amino acids. Microcystins accumulate in the liver and affect the organization of the cytoskeleton, causing the hepatocytes to collapse inwards. Although not intrinsically carcinogenic, the microcystins appear to hasten the progress of cancer. It has been suggested that the
high incidence of liver cancer in Qidong near Shanghai in China may be due to the presence of cyanobacterial toxins in drinking-water.5
245
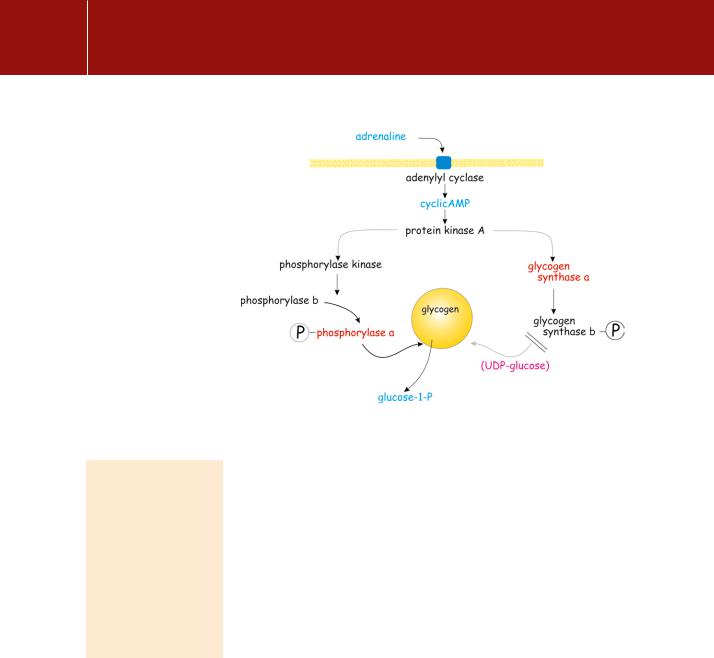
Signal Transduction
FIG 9.2 Control of glycogen breakdown.The effect of a receptor activating PKA and the phosphorylation of phosphorylase (active) and glycogen synthase (inactive)
In general, the concentration of ATP is unaffected even after prolonged periods
of acute exercise or starvation, but the level of 5 -AMP is elevated as the cell attempts to maintain its level of ATP by transphosphorylation of ADP in the reaction catalysed by myokinase: 2ADP ATP AMP
AMP indicates metabolic insufficiency. Phosphorylase a is insensitive to allosteric regulation by 5 -AMP, ATP, or glucose-6-phosphate.
The net result of all this is the transduction of a very weak first signal (low receptor occupancy by hormone) into a large kinetic signal (rapid
glycogenolysis). However, although activated target enzymes can process their substrates rapidly (in our example the release of glucose-1-phosphate from liver glycogen), the penalty for all this stage-by-stage complexity, control, and amplification is a delay in the onset of the response. The really fast systems, such as the signalling for neurotransmitter release or the contraction of skeletal muscle, are not regulated in this way. In skeletal muscle, phosphorylase kinase is activated by elevation of cytosol Ca2 which is also the signal that initiates contraction. The effect of phosphorylation by PKA is not only to maximize the rate of conversion of phosphorylase b to phosphorylase a, but also to increase its affinity for Ca2 . This ensures that muscle phosphorylase kinase is operative even under the ambient conditions of low Ca2 . In terms of the fright, fight, and flight response, adrenaline readies the muscle for maximal activity even before it receives its signal to contract (acetylcholine). The cAMP signal also acts to inhibit dephosphorylation of phosphorylase and hence its deactivation. We return to this topic in Chapter 21.
Protein kinase A
Protein kinase A is a broad-spectrum kinase. The catalytic subunit is directed at serine or threonine residues embedded in the sequence RRxS/Tx (x is
246
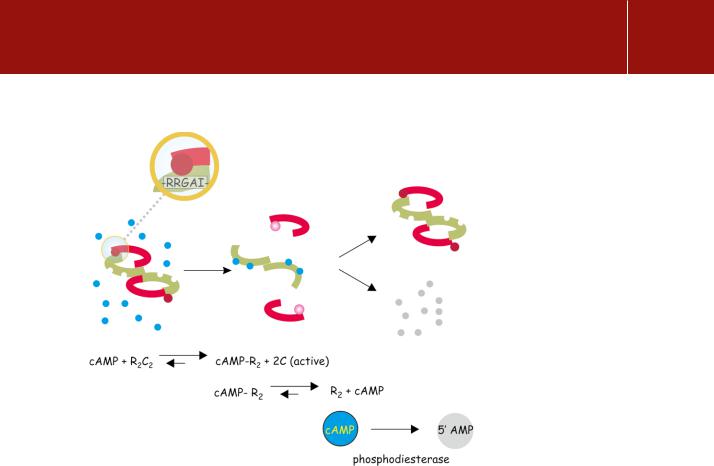
Phosphorylation and Dephosphorylation: Protein Kinases A and C
variable) and this motif is widespread. What distinguishes the different forms of PKA are the tissue-specific regulatory subunits with which they are associated.
In the absence of cAMP, PKA is inactive and exists as a stable tetramer (KD 0.2 nmol L 1), R2C2, composed of two regulatory subunits (R) and two catalytic subunits (C) (Figure 9.3). The tetramer is stabilized through an interaction between the catalytic sites and a pseudosubstrate sequence present in the regulatory subunits, which closely resembles the substrate phosphorylation consensus sequence.6, 7 For type I regulatory subunits, isolated from skeletal muscle, this is a true pseudosubstrate motif, in which the serine is replaced by glycine or alanine, RRxG/Ax, neither of which can be phosphorylated. In type II isoforms (from cardiac muscle) the serine is retained and the regulator is itself a substrate and undergoes phosphorylation.8
Autoinhibition by such motifs is a common feature of the serine/threonine protein kinases (see, for example, page 557). Here, the regulatory subunits, tightly and stably attached to each other as R2, both possess two similar binding sites for cAMP (35% identity). The binding of cAMP is cooperative, so that occupation of the first site by cAMP enhances the affinity of the vacant site. With both binding sites occupied, the stability of the R2C2 complex declines by a factor of 104 – 105, so liberating the catalytic units that can then phosphorylate their target substrates.9
FIG 9.3 Activation of protein kinase A by cAMP.
The holoenzyme is composed of two regulatory components (green) that each bind two molecules of cAMP and two catalytic units (red). The components are linked between the catalytic sites and a ‘pseudo-substrate’ sequence on the regulatory units. This has the sequence -RRGAI- which closely resembles the consensus sequence for phosphorylation (- RRGSI-). On binding four molecules of cAMP the catalytic units are released and their catalytic sites exposed for interaction with real as opposed to ‘pseudo’ substrates. The affinity of the regulatory subunits declines allowing the bound cAMP to dissociate.
It is converted to 5 -AMP by a phosphodiesterase.
247