
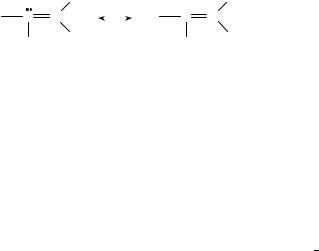
11. Electronic effects of nitro, nitroso, amino and related groups |
509 |
(polynitrotriphenyl)methanes188,189; the deprotonation of bis(2,4-dinitrophenyl)methane promoted by 1,1,3,3-tetramethylguanidine190; and the deprotonation of 1-phenyl-1- nitroethane and of 1-phenyl-2-nitropropane promoted by hydroxide ion in water or alkoxide ion in alkanol191.
4. Correlation analysis of C H acidity
It is commonly supposed that the stabilization of carbanions by CR groups is due to delocalization of negative charge from the carbanionic carbon. In the case of NO2, resonance structures as in 24 are written184:
− |
O |
|
|
O |
R1 C |
N |
|
R1 C |
N − |
|
||||
R2 |
O |
|
R2 |
O |
|
|
(24) |
|
|
with the additional possibility that some groups R1 and/or R2 may also participate in the delocalization. It might therefore be expected that correlation analysis of the effects on C H acidity of the substituent X in X C H would require electron delocalization parameters, perhaps related in some way to constants. In fact the problem of dealing with proximate substituents is much more difficult than treating the effect of a remote p-X on the acidities of phenols or anilinium ions (Sections II.A and III.D). It is much more analogous to dealing with the ortho-effect (Section IV).
Attempts at correlation analysis for X C H acidity have been made for a long time. Bowden and colleagues192 examined the problem in 1970 for the pKa values of 9-X- fluorenes and related series. For a limited selection of substituents X in 9-X-fluorenes, a correlation with Taft’s Ł values for X was found89. A more general correlation, albeit with considerable scatter, was found with M, a parameter based on LCAO MO calculations. Several dinitro-substituted diphenylmethanes fitted the line quite well and 2-nitrofluorene fitted passably; 9-cyanofluorene also fitted quite well, but malononitrile deviated strongly.
In recent years Pagani and coworkers have made detailed studies of the problem. In the space available we can only outline their work and interested readers should consult the very detailed papers. The authors have developed special scales of substituent constants for dealing with ‘contiguous functionalities’193. These new substituent constants are c (which seems to be related fairly closely to the ordinary ), IB (which bears some relationship to I, but not that close), and R , a special delocalization parameter. It is claimed194 that ‘these scales are appropriate for describing interactions between contiguous functionalities, as opposed to literature values which account for remote interactions’. Various C H acidities in gas phase and in solution were successfully correlated by means of multiple regressions on IB and R . The same parameters were used in the correlation of 13C chemical shifts for the central carbon in the carbanions.
A recent paper (1993)195 is devoted largely to the behaviour of cyano carbanions. It is concluded that the stabilization of carbanions by CN does not actually involve considerable transfer of charge from the carbanionic carbon to CN. This does not, however, appear to have any implications for the explanation of stabilization of carbanions by the nitro group in terms of delocalization.
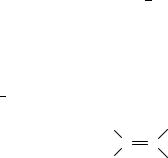
510 |
John Shorter |
B. Nucleophilic Substitution
NO2 can exert important effects on reactivity both as a substituent in the substrate and in the nucleophile, and both in aliphatic196 and in aromatic197 substitution. We shall illustrate all the relevant features of the effects of NO2 in this respect.
The normal effect of NO2 on SN1 solvolysis of substrates such as benzhydryl chloride is to retard reaction. Thus in the ethanolysis of XC6H4CHPhCl, log k values (first-order rate coefficients, s 1) are as follows (50 °C): H, 3.05; m-NO2, 5.64; p-NO2, 5.99198. The value for this reaction is about 3.7, so the log k values for the m-NO2 and p-NO2 derivatives correspond fairly closely to the values of 0.71 (or 0.73) and 0.78, respectively (Section III.B). This reaction is, of course, strongly accelerated by R para-substituents through cross-conjugation in the carbocationic transition state (Section II.A).
Although the effect of NO2 as a remote substituent on SN1 reactions is exerted through CI and CR electronic effects, there is evidence that groups which are normally -electron acceptors may function as -electron donors under extreme electron demand. Molecular orbital calculations support this idea167,172,199 and there is some experimental evidence. Much of this concerns CN rather than NO2200 203. It is mainly of the nature that when CN is actually attached to the reaction centre for SN1 solvolysis, it is not quite so retarding as might be expected from the operation of its inductive effect. However, ˛-nitrodiarylmethyl cations have been obtained and characterized by NMR204; -electron donation by NO2, as in 25, may contribute to their stabilization. There are also said to be signs of a cancelling of the normal CR effects of substituents such as NO2 and CN in the acidities of para-substituted pyridinium ions, which may indicate the onset of -electron donor behaviour172,205 207.
O R1 + N C
O R2
(25)
In contrast to the usual behaviour of the nitro group as a remote substituent in systems undergoing SN1 reactions, o-NO2 and p-NO2 are strongly activating in a substrate undergoing aromatic nucleophilic substitution. The attack of chlorobenzene by EtO in ethanol provides a suitable example. Chlorobenzene itself is not appreciably attacked below 150 °C, but the reactions of o-nitrochlorobenzene and p-nitrochlorobenzene with this reagent can be conveniently studied around 60 °C. Values of log k (second-order rate coefficients, dm3 mol 1 s 1) extrapolated to 25 °C are 6.45 and 6.05, respectively. The reaction of 2,4-dinitrochlorobenzene with EtO in EtOH can be studied at room temperature and log k at 25 °C is 1.31. Nucleophilic aromatic substitution is also activated by CN in the substrate, but not so strongly as by NO2. Thus it does not appear possible to study the effect of CN by itself on the reaction of chlorobenzene with EtO , but for 2-cyano-4-nitrochlorobenzene and 2-nitro-4-cyanochlorobenzene log k at 25 °C has the values 3.15 and 3.11, respectively, i.e. replacing NO2 by CN decreases log k by 1.84 and 1.80, respectively. Thus the reactivity decreases by a factor of about 70.
The activating effect of NO2 or CN is due to the electron-attracting effect of these groups, and in particular the CR component, assisting the delocalization of negative charge away from the reaction centre. This may be represented for the intermediate complex in terms of resonance involving structures such as 26. The relevant transition states are similarly affected197. A heterocyclic N atom is also able to assist the delocalization of negative charge away from the reaction centre. The reaction of 2-chloro- or 4-chloro- pyridine with EtO in EtOH can be studied at around 100 °C. Log k values extrapolated
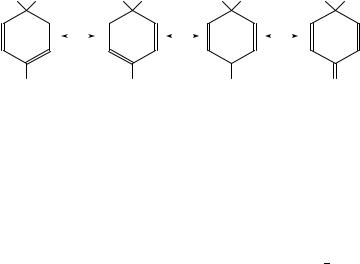
|
11. Electronic effects of nitro, nitroso, amino and related groups |
511 |
||||||||
EtO |
Cl |
|
EtO |
Cl |
|
EtO |
Cl |
|
EtO |
Cl |
|
− |
|
− |
|
|
|
|
|
|
|
|
|
|
|
|
|
|
|
|
|
|
|
|
− |
|
NO2 |
NO2 |
NO2 |
NO2 − |
|
|
(26) |
|
to 25 °C are 8.4 and 6.8, respectively208, rather lower than those for the corresponding chloronitrobenzenes. However, the presence of two heterocyclic N atoms exerts a powerful influence, e.g. log k for 2-chloropyrimidine at 25 °C is 2.60208.
The reaction of EtO with chloro compounds in ethanol is actually not an entirely satisfactory system for studying the activating effect of CN on aromatic nucleophilic substitution, because there is a tendency for CN itself to react, forming the conjugate base of iminoether, C(OEt)DNH209.
The activating effects of NO2, CN and heterocyclic N have been much studied in the reactions of 2-chloro-3-cyano-5-nitropyridine (and related compounds) with aniline and substituted anilines and other amine nucleophiles in various solvents210 212. 2-Chloro- 3-cyano-pyridine is too unreactive for study, but the reaction of 2-chloro-5-nitropyridine with aniline in methanol gives a log k value of 5.31 at 40 °C210. For the corresponding
reaction of 2-chloro-3- |
cyano-5-nitropyridine log k is |
|
1.68, i.e. the activation by CN is |
||
|
211 |
|
|
||
by a factor of over 4000 |
|
. The influence of inserting methyl groups adjacent to the |
nitro group of 2-chloro-3-cyano-5-nitropyridine was also studied. For 2-chloro-3-cyano- 6-methyl-5-nitropyridine reacting with aniline in methanol at 30 °C, log k D 2.50, and for 2-chloro-3-cyano-4,6-dimethyl-5-nitropyridine log k D 4.38, compared with 1.93 for the substrate without methyl groups. Thus 6-Me decreases the rate by a factor of about 3.7, while methyl groups in both positions adjacent to the nitro group together decrease the rate by a factor of about 282. The latter is certainly due to the methyl groups twisting the nitro group out of the plane of the ring and thereby inhibiting the CR effect (Section III.B,D). The small retarding influence of 6-Me by itself may be partly due to the I effect of the methyl group, which is in the meta position with respect to the reaction centre, but will largely be due to the onset of the effect of twisting the nitro group.
The kinetics of the reactions of 2-fluoro-3-cyano-5-nitropyridine with various amine nucleophiles have also been studied211. These are complicated by the occurrence of base catalysis.
It might be expected that the activating effect of p-NO2 on nucleophilic aromatic substitution would be related to the value of the substituent. From various studies of nucleophilic aromatic substitution, Miller and Parker213 obtained a value for p-NO2 of 1.27, very close to the values based on the ionization of substituted phenols or anilinium ions (Section III.D).
Numerous observations have been made of the relative activating effects of o-NO2 and p-NO2 in a substrate undergoing nucleophilic aromatic substitution214. In some systems o-NO2 < p-NO2; this may be ascribed to differences in the orientation of dipoles and to a change in the CR effect of the group as between the ring positions (see Section IV). In other systems, however, the activating effects are in the order o-NO2 > p-NO2, sometimes markedly so. In such cases a favourable influence of hydrogen-bonding involving the o-NO2 group and the nucleophile has been invoked.
512 John Shorter
It should also be mentioned that NO2 is the electron-attracting substituent which is most commonly used to stabilize Meisenheimer-type complexes. See, for example, recent studies of the reaction of 1,3,5-trinitrobenzene and phenoxide ions215, and the reactions of 4,6-dinitrobenzofuroxan with 5-substituted indoles216. See also the appropriate chapter in each year of the Series Organic Reaction Mechanisms181.
The introduction of NO2 into the aniline molecule would be expected to reduce the nucleophilicity of NH2 and for p-NO2 this should be more or less according to . Examples of this are not easy to find, but there are the necessary data for the reactions of picryl chloride with substituted anilines in methanol. Log k for the reaction of aniline itself is about 0.2 at 25 °C (extrapolated from measurements at much lower temperatures). For the reaction of p-nitroaniline log k is 4.06; is about 3.4. These values give an apparent sigma value of 1.13 for p-NO2 in this system, a little below the value for of about 1.25 (Section III.D).
An example of the reduction of amine nucleophilicity by the CI effect of NO2 is provided by the results of Grob and Schlageter217 on the quaternization of 4-X-substituted quinuclidines (cf structure 18) with methyl iodide in methanol at 10.0 °C. According to Charton109, the I value for this reaction is 1.12, and log k values for X D H and NO2 are2.35 and 3.18, respectively217. These give an apparent I value of 0.74 for NO2, a little higher than Charton’s value of 0.67 (Section III.A). Grob and Schlageter217 carried out an analogous correlation in terms of Iq, an inductive parameter based directly on the pKa values of 4-X-substituted quinuclidinium ions (cf structure 18) as a standard system218, for which I is defined as 1.000. The Iq value for NO2 is 3.48. Grob has made extensive use of the Iq scale in correlating the effects of substituents on log k for various reactions, including the solvolyses of 1-substituted 3-bromoadamantanes219, 4-substituted 2-chloro- 2-methylbutanes220 and 4-substituted bicyclo[2.2.2]octyl p-nitrobenzenesulphonates221 NO2 features as a substituent in all of these, except the last-mentioned221, and they thus present examples of the retarding effect of remote NO2 in the SN1 reactions of saturated aliphatic and carbocyclic systems222.
C. Electrophilic Aromatic Substitution
The nitro group is deactivating in electrophilic aromatic substitution. There is much less literature to deal with than there was for the article on ether and hydroxyl groups5. As in the case of the previous articles5,6. Roger Taylor’s excellent monograph223 is a good guide to the literature up to about 1990 and references to particular parts of this book will be appropriate.
Table 6 displays data for the isomer proportions formed in the nitration and halogenation of nitrobenzene224. As with all CI, CR substituents, the formation of the meta isomer predominates. The reactions are far slower than those of benzene itself. Partial rate factors
for the reaction of nitrobenzene with HNO3 |
|
H2SO4 are as follows: fo, 1.08 ð 10 8; fm, |
||||||||||
|
||||||||||||
TABLE 6. Isomer distributions (%) for nitration or chlorination of PhNO2224 |
|
|
||||||||||
|
|
|
|
|
|
Temp. |
|
|
|
|
|
|
Reaction |
Reagent |
(°C) |
|
o |
m |
p |
1/2o:p |
1/2m:p |
||||
Nitration |
HNO3 |
|
|
|
0 |
6.4 |
93.2 |
0.3 |
10.7 |
155 |
||
|
HNO3 |
|
|
H2SO4 |
25 |
6.1 |
91.8 |
2.1 |
1.5 |
21.9 |
||
|
|
|
||||||||||
|
HNO3 |
|
|
CF3COOH |
75 |
10.5 |
84.7 |
4.8 |
1.1 |
8.8 |
||
|
|
|
||||||||||
Chlorination |
HOCl |
|
|
HC |
? |
17.6 |
80.9 |
1.5 |
5.9 |
27.0 |
||
|
|

11. Electronic effects of nitro, nitroso, amino and related groups |
513 |
16.2 ð 10 8; fp, 7.26 ð 10 9 (25 °C)224. In the formation of the minor products, ortho substitution tends to be favoured relative to para, as shown by the ratios 1/2o:p. At one time special interactions between the reagent and the substituent were often invoked to account for the ortho preference, which is now known to be widespread for other CR substituents and other electrophilic reagents. It is more likely that a general greater deactivation of the para position is responsible. The pattern of 1/2m:p ratios (Table 6) is also in accord with this. It has been suggested that the greater deactivation of the para position is due to it carrying a slightly greater share of the positive charge than either ortho position in the Wheland intermediate224, and there is quantum-mechanical support for this idea.
In highly acidic media the actual state of substituents such as NO2 with regard to protonation or hydrogen-bonding is somewhat problematical. The slightly reduced meta preference for reactions in HNO3 CF3COOH (Table 6) suggests some difference in the state of the substituent in this medium, but it should be noted that these reactions were at a rather higher temperature.
There are extensive data for the acid-catalyzed protiodesilylation of XC6H4SiMe3 in methanol aqueous perchloric acid or acetic acid aqueous sulphuric acid at 50 °C225. Correlation analysis of the partial rate factors (relative rate constants) by means of the Yukawa Tsuno equation (Section II.B) finds D 5.3 and rC D 0.65. These values are consistent with a relatively low demand for stabilization of the transition state by electron delocalization, i.e. the transition state is ‘early’ along the reaction coordinate. p-NO2 is highly deactivating with f D 14 ð 10 5, but o-NO2 is even more deactivating, with f D 6.8 ð 10 5. This contrasts with the deactivation order discussed above for nitration and chlorination (Table 6), and may be explained in terms of the ‘early’ transition state, well removed from the Wheland intermediate.
Data also exist for the base-catalyzed protiodesilylation of XC6H4SiMe3 in aqueous DMSO226. This reaction involves the base-catalyzed cleavage of the silicon compound to give an aromatic anion Ar , and the usual substituent effects in electrophilic substitution are reversed: electron-attracting groups increase the reaction rate and electron-withdrawing groups decrease it. Thus the m- and p-nitro compounds are about 105 times more reactive than the parent (X D H) compound.
Partial rate factors for the sulphonation of compounds Ph(CH2)nNO2 have been measured227, n being 0, 2 or 3. This is another system in which the ortho position is deactivated more than the para. Under the highly acidic conditions used it seems likely that the effective substituent is the hydrogen-bonded or even protonated group.
In Taylor’s tabulation of sigma values228, C and for p-NO2 are given identical values of 0.79228. No distinction between these two types of substituent constant is to be expected for p-NO2 in connection with electrophilic aromatic substitution. Some slight distinction between them is drawn for m-NO2: D 0.72, C D 0.73.
VII. SUBSTITUENT CONSTANTS OF NO2 AND OF GROUPS CONTAINING IT:
RECAPITULATION AND SOME EXTENSION
A. Introduction
At the end of this long account of the electronic effects of NO2, it seems useful to bring together and summarize some of the material which is scattered throughout it. Also, there are some relevant substituents which have not so far been mentioned, including polynitro groups and various other substituents in which NO2 is attached to some atom or group, which is in turn bonded to the molecular skeleton.
514 John Shorter
B. Recapitulation
For NO2 the recommended values of m and p (benzoic acid scale) in the IUPAC document79 are 0.73 and 0.78, respectively, compared with the ‘traditional’ values of 0.71 and 0.78, respectively (Section III.B). When these values are used for correlations of processes taking place in other than highly aqueous media, the possibility of specific solvent effects should be borne in mind. The fact that the values of NO2 are very much at the upper end of the scale for commonly used substituents means that they exert a strong influence in regression analysis and there is danger of their biassing a correlation unduly.
For correlations in which 0 values are considered relevant, the ordinary m and p should be used (Section III.C). Similarly for correlations of electron-demanding processes in which the use of C values is appropriate for R substituents, the ordinary m and p values of NO2 should be used (Section VI.C). When the use of values is appropriate for CR substituents, the value 1.25 may often be used for NO2 (Section III.D, but see also Section V.A).
As to I, the value of 0.67 for NO2 seems to be of wide application (Section III.C), but a slightly lower value, 0.65, may also be considered (Section V.A). Here also the possibility of solvent effects and the dangers of bias in correlations must be borne in mind. With R or R0 the range of values obtained by different methods presents a problem. A generally useful value for both these parameters appears to be 0.10, but it must be borne in mind that there is a school of thought which favours an appreciably higher value, at least 0.15 (Section V.A).
The I value for CH2NO2 may be taken as 0.25 (Section III.A).
C. The Effects of Substituents Containing NO2
The various compilations of sigma values provide rather patchy information on this topic78,109,118,150. It would be very interesting to have full information on the series CHn(NO2)3 n, but what is available is rather incomplete. As mentioned above, there is a I value for CH2NO2 of 0.25, but there are no values for m and p in the literature. There are a few values for nitro-substituted alkyl groups which can be arranged in a sensible series as follows (substituent, m, p): CMe2NO2, 0.18, 0.20; CMe(NO2)2, 0.54, 0.61: CEt(NO2)2, 0.56, 0.64; C(NO2)3, 0.72, 0.82. The p values were experimentally determined, but not directly based on the ionization of substituted benzoic acids, while the m values were calculated from the p values by applying a factor, and Exner regards them as rather uncertain78. Presumably the inductive effect is easily the more important component of the electronic effect of these groups and the value of p for CMe2NO2 seems quite reasonable for the influence of a nitro group damped by an interposing carbon atom. Successive nitro groups become less effective: the cumulative effect of nitro groups attached to the same carbon atom would not be expected to be strictly additive.
This would be an appropriate point to deal with what is known about that little used substituent ONO2. From the pKa value of 2.26 for the appropriately substituted acetic acid in water, Charton112 calculated I for ONO2 to be 0.62, which he later revised to 0.66109. Thus the inductive effect of the group appears to be very similar to that of NO2. Presumably the damping effect of the interposed O on the inductive effect of NO2 is just about counterbalanced by the contribution of the electron-attracting O atom. Various sources give values for m and p of 0.55 and 0.70, respectively78,150. Exner78 considers these to be estimated rather than experimental values and regards them as rather uncertain. The resonance effect of this group would be expected to be small; the normal R effect of an O attached to a benzene ring5 will be opposed by the electron-attracting effect of

11. Electronic effects of nitro, nitroso, amino and related groups |
515 |
the NO2 moiety. The above values of m and p do not seem to harmonize very well with the value of I.
VIII. ELECTRONIC EFFECTS OF THE NITROSO GROUP
The NO group is a strongly electron-attracting group. The dipole moment of nitrosobenzene is 3.14D, compared with 3.97 for nitrobenzene. The typical dipole moments of aliphatic compounds involving these groups are smaller: 2.51D and 3.14 respectively229. These values indicate that NO, like NO2, may exert CI and CR electronic effects (Section II.B). There is, however, one important difference between these two groups: the N atom in NO has a lone pair of electrons, and in consequence the group is also capable of exerting a R effect. This dual nature of NO is indicated in the structures 27. A consequence of the possibility of a R effect is that the NO group is ortho/para directing for aromatic electrophilic substitution. This matter does not seem to have been much investigated, but played a part in the dispute between Robinson and Ingold regarding electronic theories of organic chemistry (Section I.B46,50,53).
− |
+ |
|
N O |
|
+ |
|
− |
N O |
|
|
|
N O |
|||
|
(27) |
|
|
|
|
|
|
|
The main quantitative indication of the C |
I |
effect which is |
available is from 19F NMR |
|||
|
|
|
96,230 |
, but this has |
|||
shielding (Section V.A). This originally yielded a I |
value of 0.33 |
|
now been revised to 0.41150. (A different regression equation was used.) At all events, the inductive effect of NO is clearly somewhat less than that of NO2, which seems reasonable in relation to nitroso having one electronegative O atom and nitro having two. The 19F NMR method yielded a R value of 0.3297,230, but this has now been revised to 0.25150. Thus the CR effect of NO appears to be rather stronger than that of NO2. This point is referred to again below. It must be remembered that for a CR substituent the resonance parameter derived from 19F NMR is not to be identified with a R0 constant, because the resonance effect is slightly enhanced by cross-conjugation between the substituent and F.
On the basis of the older values of I and R, Exner78 calculated m0 and p0 values of 0.49 and 0.65, respectively, by employing equations 6 and 7 (Section II.B). From the newer values of I and R, Hansch and colleagues150 used a slightly more complicated procedure to calculate substituent constants c m and c p (c D calculated) as 0.48 and 0.62, respectively. The two estimated sets of sigma values for NO agree fairly well with each other. Unfortunately the experimental values of m and p for NO, based on the dissociation constants of the substituted benzoic acids, are considerably higher: 0.62 and 0.91, respectively150,231. When interpreted in terms of equations 4 and 5 (Section II.B), these values yield I D 0.48 and R D 0.43. The latter value seems highly improbable; no dipolar CR substituent is recorded as having such a large value.
There is a further problem regarding a value for the resonance parameter of NO. The infrared method (Section V.A) yields a R0 value of š0.07154, cf 0.31 or 0.25 above.
The area of chemical reactivity for which there is most information regarding the electronic effects of NO is that involving processes to which a p constant might be applicable, but even here the experimental evidence is not entirely straightforward. From studies of aromatic nucleophilic substitution (Section VII.B), Miller and Parker213 obtained a

516 John Shorter
value for p-NO of 1.46. i.e. appreciably higher than that of p-NO2 in aromatic nucleophilic substitution, 1.27. (Table 9 of Reference 197 gives 1.486 for p-NO. Presumably this is a value that was recalculated from Reference 213 for some reason.) Miller232 supposed that the inductive effect of NO would be rather smaller than that of NO2, so that the higher value of NO must indicate a greatly superior conjugative power of NO for activating nucleophilic aromatic substitution. This he attributed to the internal conjugation of the group being greater for NO2 than for NO.
There are several other putative estimates of an exalted CR effect of NO. A value of 1.63 for of p-NO was based on the dissociation constants of substituted phenols in water233, and of 1.60 on the dissociation constants of phenols in 1:1 EtOH H2O134. These values are in reasonable agreement with the above value based on aromatic nucleophilic substitution. However, the measurement of the dissociation constants of substituted N,N- dimethylanilinium ions in water at 25 °C233 led to the extraordinary value of 0.15 for. It must be emphasized that the experimental work in this paper233 appears to be very reliable. Further, there are indications of a similar anomaly in limited data for N,N- dipropylanilinium ion and its 4-NO derivative, whose pKa values in water are about 5.7 and 4.2, respectively. Since the value for this ionization will be around 3, the apparent value indicated for p-NO is about 0.5, certainly very much lower than the values of about 1.4 to 1.6 discussed above. There is no doubt that these anomalies arise from tautomerism of the substrate. The authors233 argue that the protonated form of 4- nitroso-N,N-dimethylaniline exists as a tautomeric equilibrium of a ‘nitroso’ form and a ‘quinonoid’ form, with the latter greatly predominating, i.e. the protonation is of NO rather than NMe2. This would account for the greatly reduced apparent value of NO. The same type of tautomerism might occur with 4-nitrosophenol, but the reasonable agreement of the apparent value for NO with that based on nucleophilic aromatic substitution shows that the ‘nitroso’ form greatly predominates.
One final anomaly: we have already commented on the enhanced value for the effect of para-substituents on the ionization of 2,6-di-tert-butylphenol in 1:1 v/v EtOH H2O at 25 °C (Section III.D). In this solvent phenol itself has a pKa value of 11.16, and the values for the 4-NO and 4-NO2 derivatives are 6.9 and 7.49, respectively134, i.e. NO has a greater acid-strengthening effect on phenol than NO2 does, as we have already seen above for solutions in water. However, the pKa values of 2,6-di-tert-butylphenol and its 4-NO and 4-NO2 derivatives in 1:1 v/v EtOH H2O are 14.22, 9.41 and 7.49, respectively134, i.e. the acid-strengthening effects of NO and NO2 are reversed. The apparent value of p-NO is now about 0.9. Presumably there is a considerable amount of ‘quinonoid’ form in this system.
IX. ELECTRONIC EFFECTS OF THE AMINO GROUP AND RELATED GROUPS
A. Introduction
In the space available this account will have to be rather concise. However, the much more detailed account of the nitro group contains much background material which will not need to be repeated.
The electronic effects of groups of the general formula NX1X2 are governed primarily by the electronegative character of N relative to C and by the lone pair of electrons on the N atom. They therefore exert CI and R effects, moderated by the electronic effects of X1 and X2. As these moieties become more electron-attracting in character, the CI effect of the substituent as a whole is increased and the R effect is decreased, and in the limit may become effectively zero.
These substituents present certain problems for the study of their electronic effects. These arise from the behaviour of the substituents as basic centres. Thus they are liable
11. Electronic effects of nitro, nitroso, amino and related groups |
517 |
to be protonated in acidic media and the study may then be of the electronic effects of NX1X2HC rather than of NX1X2, or the electronic effect may be composite because of partial protonation. This type of complication may also arise in the absence of any acidic medium if the compound under study contains an acidic grouping, such as COOH. Thus aliphatic amino acids tend to exist in solution as zwitter-ions, and measurements of the pKa values yield information about the effect of the pole NH3C and not the dipole NH2. In aromatic amino-acids, however, the NH2 is less basic and the problem is then the need to apply corrections for a small content of zwitter-ion. The general problem becomes less serious when any acidic group in the molecule becomes very weak and also for NX1X2 when basicity is reduced by the electron-attracting nature of X1 and/or X2.
The amino group is both a hydrogen-bond donor and a hydrogen-bond acceptor. Solvents may therefore modify the electronic effects of the amino group by engaging with it in hydrogen-bond acceptor or hydrogen-bond donor interaction, respectively. For the general amino substituent NX1X2 the possibility of the group acting as a hydrogen-bond donor will clearly depend on X1 or X2 being H. Electron-attracting groups X1 and/or X2 will decrease the tendency of N to act as a hydrogen-bond acceptor, but there may be the possibility of X1 and/or X2 acting as hydrogen-bond acceptor.
B. Inductive Effect of the Amino Group and Related Groups
For NH2 and certain related groups the most convenient study of chemical reactivity which gives information about the inductive effects of the groups is the measurement of the pKa values of 4-substituted quinuclidinium ions (cf Section III.A). In this way Charton109 finds values for I as follows: NH2, 0.17; MeNH, 0.13; Me2N, 0.17; EtO2CNH, 0.28. (The experimental work was by Grob and Schlageter218.) When the substituent NX1X2 contains more electron-attracting groups, Charton considers that values of I may be safely based on the ionization of substituted acetic acids, because zwitter-ion formation will be negligible. In this way he finds the following values109: PhNMe, 0.15; AcNH, 0.28; BzNH, 0.28; PhNH, 0.30; HCONH, 0.33; PhSO2NH, 0.33; ClCH2CONH, 0.35; Me(NO2)N, 0.39.
In his work on the analysis of substituent effects in aliphatic ester reactions, Taft87,89 derived Ł values, from which I values may be calculated as follows: NH2, 0.10; Me2N,
0.10; AcNH; 0.28. Thus there are some discrepancies as between the results of Charton109 and of Taft87,89.
The largest number of I values has been obtained from 19F NMR substituent chemical shifts (Section V.A). Taft has been involved in measurements of this kind for around 40 years96,97. Fairly recently these data have been compiled and reanalyzed, with an emphasis on data for dilute solutions of substrates in hydrocarbon solvents. Some values for the amino group and related groups are as follows150: NH2, 0.09; NHNH2, 0.17; PhNH, 0.31; AcNH, 0.34; NHCN, 0.44; N(CF3)2, 0.55; N(COF)CF3, 0.62. A value for NMe2 is given as 0.17, but this is considered to be unreliable ‘because of magnetic or other complications’150.
It will be seen that the I values for NX1X2 increase as the electron-attracting character of X1 and X2 increases. To set these values in context in the I scale, we quote a few values for other kinds of substituents150: OMe, 0.30; Cl, 0.43; COF, 0.45; CF3, 0.46; CN, 0.57; SO2Me, 0.61; NO2, 0.64. The largest I values are provided by positive poles, e.g. NMe3C , 0.99. The significance of such values in relation to the wider field of linear free energy relationships is, however, a matter of debate234.
Exner tabulates I values for many NX1X2 substituents, mainly measured by the 19F NMR method78.
518 |
John Shorter |
C. Resonance Effect of the Amino Group and Related Groups
The resonance effects of these groups are greatly enhanced when they are exerting an influence on highly electron-demanding processes. This topic will be discussed later. Here we shall be concerned with the resonance effects as shown under milder conditions and measured either by R0 or by R(BA) (Section II.B). For brevity we shall refer to the latter simply as R. The distinction between the two types of resonance parameter is important with I groups, cf the nitro group (Sections III.C and V.D).
Charton derives R values simply by subtracting I values from p values, i.e. classical
Hammett benzoic acid-based substituent constants109. In the next section the derivation of refined values for NH2 etc. will be discussed, i.e. the matter of applying a correction to allow for the zwitter-ion content of the substituted benzoic acid. Here we shall simply accept that Charton109 uses what he believes to be the most reliable values of p available to him. In this way Charton finds values for R as follows: NH2, 0.80; Me2N, 0.88; PhNH, 0.86; EtO2CNH, 0.48; BzNH, 0.47; ClCH2CONH, 0.42; HCONH, 0.40; AcNH, 0.35; PhSO2NH, 0.36.
We set these in context in the R scale by quoting values for a few substituents of other types; Me, 0.16; ClCH2, 0.08; Cl, 0.25; F, 0.48; MeO, 0.58. The NH2 group and its simplest derivatives in fact define the negative end of this resonance scale. The effect of introducing electron-attracting groups into NH2 and making the lone pair of electrons less available for delocalization is clearly seen.
A large number of R0 values has been obtained from 19F NMR substituent chemical shifts (Section V.A.). A selection may be drawn from the compilation of data to which reference has already been made150: NH2, 0.48; NMe2, 0.56; NHNH2, 0.42; PhNH,0.35; NHCN, 0.30, AcNH, 0.21; N(CN)2, 0.21; N(COF)CF3, 0.01; N(COF)2, 0.01; N(CF3)2, 0.02.
The R0 value for a given group is much less negative than the R value. The effect of introducing electron-attracting moieties is clearly seen; in the limit the R effect is completely inhibited; the very small positive values may be taken as zero within experimental error. We set these values in context by quoting a few values of R0 for other types of substituents: Me, 0.13; CH2Cl, 0.02; OMe, 0.43; Cl, 0.16. It is also of interest to show a few of Charton’s R0 values, reactivity-based and determined as described in his review109: NH2, 0.42; NMe2, 0.44; AcNH, 0.25; HCONH, 0.24. Agreement with the 19F NMR based values seems only fair, but those are for rather non-polar conditions, while Charton’s values are for aqueous or aqueous organic solvents.
Values of R0 for a large number of NX1X2 groups have been determined by infrared
intensity measurements154 (Section V.A). A few values are as follows: NH2, 0.47; Me2N, 0.53; NHNH2, 0.49; PhNH, 0.50; AcNH, 0.41; Ph2NH, 0.44; N(CF3)2,0.13. It should be recalled that the sign of the resonance effect is not actually given by this method, but must be inferred from other knowledge. Agreement with the 19F NMR values is quite good in several cases, but there are particularly marked discrepancies for AcNH and PhNH.
Exner tabulates R0 values for many NX1X2 substituents, measured by the 19F NMR method and/or the infrared intensity method78.
D. Electronic Effects of the Amino Group and Related Groups on the Strengths of Benzoic and Other Acids
The ionization of m- or p-aminobenzoic acid is characterized by two pKa values, for which we shall use the symbols pK1 and pK2. The former is essentially concerned with the ionization of NH3C under the influence of COOH, the latter with the ionization of the