

11. Electronic effects of nitro, nitroso, amino and related groups |
499 |
the undissociated form of the acid relative to the carboxylate ion, thereby enhancing acid strength. The deconjugation effect is shown most clearly in o-t-butylbenzoic acid, whose pKa value is 3.54: cf the acid-weakening effect of the group in the para-position, pKa D 4.40. The deconjugation effect must play some part in the case of the more bulky polar groups, for example SO2Me, but not much in the case of F. In the case of NO2 much will depend on whether the group is essentially co-planar with the ring or twisted at right angles to the ring (see below). (According to a recent paper by Exner and coworkers, the importance of the deconjugation of the carboxyl group by twisting has sometimes been exaggerated138.) Another factor in the case of some substituents is hydrogen-bonding. Thus the very large increase in acidity as between p-hydroxy- and o-hydroxy-benzoic acid. pKa values 4.58 and 3.03 respectively, is attributed to the stabilization of the anion of salicylic acid by internal hydrogen-bonding139.
Some further light on the behaviour of the nitro group as an ortho-substituent in affecting the strength of benzoic acid can be obtained by correlation analysis employing the extended Hammett equation (Section II.B) in the form of equation 14:
pKa D ˛ I C ˇ R C C h |
14 |
where I and R are, respectively, the inductive and resonance constants of Taft’s analysis of ordinary Hammett constants (see Section II.B) and is the steric substituent constant developed by Charton140 142. There are plenty of data for the ionization of ortho- substituted benzoic acids in water at 25 °C, but unfortunately correlation analysis proves not to be altogether straightforward. The establishment of the regression equation cannot be done unambiguously through the use of ‘well-behaved’ substituents (cf Section IV.C), and in the various equations that may be derived by taking various selections of substituents, the regression coefficients and the intercept term vary quite a lot. It would not be appropriate in this chapter to go into great detail. Suffice it to say that a fairly satisfactory equation may be based on 16 substituents: H, Me, Et, Pri, But, F, Cl, Br, I, OMe, OPh, SMe, NH2, SH, NO2, Ph. Charton’s values of I and R have been used (these were derived originally in connection with the correlation analyses outlined in Section IV.C) and NO2 and Ph are assumed to lie orthogonal to the benzene ring (see below). Regression equation 15 was obtained:
pKa D 2.478 I |
C 1.803 R |
C 0.763 4.093 |
(15) |
(š0.132) |
(š0.130) |
(š0.109) |
|
n D 16, R D 0.988, s D 0.111, D 0.178
(R D multiple correlation coefficient; the other symbols have the same meanings as in connection with equations 12 and 13 in Section III.D.)
A rather striking feature of this equation is that the intercept 4.093 does not correspond closely to the observed pKa value of 4.205 for benzoic acid itself, i.e. this acid is not acting as the true parent of the series of ortho-substituted benzoic acids. Examination of the data and the results of the correlation analysis suggests that all the ortho-substituted acids receive an initial increment in acidity caused in some way by the replacement of o-H by any other substituent. The regression was therefore repeated without the benzoic acid point and equation 16 was the result:
pKa D 2.360 I |
C 1.910 R |
C 0.588 3.920 |
(16) |
(š0.140) |
(š0.135) |
(š0.142) |
|
n D 15, R D 0.990, s D 0.103, D 0.166
From this equation it appears that the effective parent acid is at a pKa value of 3.92. The regression coefficients of both the inductive effect and resonance effect terms indicate
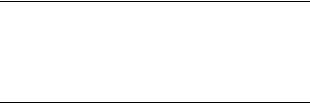
500 John Shorter
a considerable increase in the transmission of these effects as compared with the para- position (in the corresponding regression the coefficients would be about unity), with a marginal increase in the importance of the inductive effect compared with the resonance effect. The term indicates a significant contribution from the steric effect of the substituent deconjugating the carboxyl group by twisting. For the nitro group in an orthogonal conformation with respect to the benzene ring the substituent constants have the valuesI D 0.67, R D 0.00 and D 0.35. The insertion of these values into equation 16 gives a pKa(calc) value of 2.133, in very good agreement with the observed value 2.173. If the nitro group is coplanar with the benzene ring, the values of the substituent constants areI D 0.67, R D 0.10 and D 1.39. It is not possible to obtain an acceptable regression equation incorporating these values for the nitro group. Thus it appears that in an aqueous solution of o-nitrobenzoic acid the nitro group is essentially orthogonal to the benzene ring.
Before we leave the ortho-effect of NO2 as manifested in the ionization of o- nitrobenzoic acid, we will examine briefly the cumulative effect of two nitro groups on the ionization of benzoic acid. The necessary data are in Table 5. The effect of the two groups in the 3,5- and 2,4-dinitrobenzoic acids is almost strictly additive. The 3,4 acid shows a slight departure from additivity, probably due to mutual twisting of the nitro groups diminishing the resonance effect, particularly of the p-NO2. In 2,5-dinitrobenzoic acid there is a greater departure from strict additivity, probably the ‘saturation’ effect of having two strongly electron-attracting groups in the para disposition to each other. The most marked departure from additivity is shown by the 2,6 acid, which is considerably weaker than required by a strictly additive effect. The most likely explanation lies in steric inhibition of solvation of the carboxylate ion. The smaller departure from additivity shown by 2,3-dinitrobenzoic acid may be an indication of some steric inhibition of solvation, produced by the 3-nitro group increasing the steric interaction of the 2-nitro group with
the carboxylate group |
|
the ‘buttressing’ effect. |
|
|
|
|
|
||
There are data for |
the effect of o-NO2 in |
several acids |
of the general type |
|
Ph G COOH. The pKa values (water, 25 °C) |
of the parent |
acids and p-NO2, o- |
NO2 pairs for various G are, respectively, as follows: CH2, 4.31, 3.85, 4.00; CH2CH2, 4.66, 4.47, 4.50; OCH2, 3.17, 2.89, 2.90; SCH2, 3.38, 3.09, 3.10. (The data for the acids with G D SCH2 are at 20 °C and are ‘mixed’ constants at an ionic strength of 0.10.) Thus the rather small effects of NO2 on the acidities of these acids differ little as between the para- and the ortho-position. This is typical of the effects of polar substituents in these systems, in which no steric inhibition by the ortho-substituent of conjugation involving the carboxyl group can occur. Presumably any increase in the transmission of the inductive effect of the substituent in moving from the para- to the ortho-position is more or less cancelled out by changes in the orientation of substituent dipoles relative to the reaction centre and possibly steric inhibition of the resonance effect of the nitro group.
TABLE 5. Cumulative effect of two nitro groups on the strength of benzoic acid in water at 25 °C107
Isomer(NO2)2 |
pKa |
pKa (obs)a |
pKa(calc)a |
2,3 |
1.85 |
2.35 |
2.74 |
2,4 |
1.42 |
2.78 |
2.81 |
2,5 |
1.62 |
2.58 |
2.74 |
2,6 |
1.14 |
3.06 |
4.06 |
3,4 |
2.82 |
1.38 |
1.49 |
3,5 |
2.82 |
1.38 |
1.42 |
apKabenzoic acid, 4.20; pKa values: o-NO2, 2.03; m-NO2, 0.71; p-NO2, 0.78.
11. Electronic effects of nitro, nitroso, amino and related groups |
501 |
In phenol ionization, pKa values (water, 25 °C) for some p-X, o-X pairs are as follows: F, 9.91, 8.71; Cl, 9.42, 8.53; Br, 9.36, 8.44; CN, 7.95, 6.90; NO2, 7.15, 7.23; compared with 10.00 for phenol itself. (Various different values may be found in the literature107c for some of these substituted phenols. As far as possible the individual values quoted for each pair have been determined by the same authors.) The patterns for Hal and CN are similar, but that for NO2 is different, the isomeric phenols differing little in acidity. The increase in acidity when an electron-attracting group is moved from the para- to ortho-position in phenol is doubtless due to an increased transmission of the inductive effect to the reaction centre, which more than outweighs any unfavourable effect of change in orientation. The anomalously low acidity of o-nitrophenol is usually attributed to stabilization of the undissociated form by hydrogen-bonding between OH and ONO.
For the ionization of the anilinium ion, pKa values (water, 25 °C) for some p-X, o- X pairs are as follows: F, 4.65, 3.20; Cl, 4.05, 2.71; Br, 3.95, 2.55; CN, 1.75, 0.95; NO2, 1.13, 0.05; compared with 4.60 for the anilinium ion itself. Thus in every case a marked increase in acidity occurs when the substituent is moved from the para- to the ortho-position, although the changes for CN and for NO2 are somewhat smaller than for the Hal. It might have been expected that at least with o-NO2 hydrogen-bonding would play a part in stabilizing the protonated form relative to the neutral form, but there is no clear indication of this. Such an effect is apparent for the N,N-dimethylaniline system: p-NO2, 0.61; o-NO2, 2.92, compared with 5.15 for the N,N-dimethylanilinium ion itself. Here no internal hydrogen-bonding is possible in the unprotonated form and the stabilization of the protonated form by hydrogen-bonding between NHC and ONO leads to a very marked decrease in acidity.
C. The Reactions of ortho-Substituted Benzoic Acids with
Diazodiphenylmethane (DDM)
The point has already been made (Section IV.A) that discussion of the ortho-effect is frequently hampered by a lack of data for the wide range of ortho-substituted compounds necessary for satisfactory correlation analysis by means of the appropriate form of extended Hammett equation95. This situation was remedied some years ago by the present author and his colleagues143,144 for the reactions of ortho-substituted benzoic acids with DDM. Rate coefficients (l mol 1 min 1) at 30 °C were measured for the reactions of benzoic and 32 ortho-substituted benzoic acids in 11 alcohols (including 2-methoxyethanol) as solvents143. The reaction involves a rate-determining proton transfer from the carboxylic acid to the DDM to form a diphenylmethanediazonium carboxylate ion-pair. Subsequent fast product-governing stages have been variously formulated143. A more restricted study was carried out for reaction at 30 °C of the substituted benzoic acids in 7 aprotic solvents144, in which the proton transfer is believed to be rate-limiting rather than rate-determining.
The correlation analysis employed the extended Hammett equation in the form of
equation 17: |
17 |
log k D ˛ I C ˇ R C ϕ C h |
(The symbols were defined in Section IV.B.) A full discussion of the ortho-effect as revealed in this work would be inappropriate here. We must restrict ourselves to the more limited task of indicating the role of o-NO2. We discuss first the work involving alcohols as solvents. To apply the extended Hammett equation, i.e. to determine the regression coefficients ˛, ˇ and ϕ and the intercept term h, it is first necessary to select a set of substituents which can be expected to be ‘well-behaved’. Particular problems for R andmay be caused by conformational effects, and internal hydrogen-bonding may occur
502 |
John Shorter |
as a further factor governing reactivity, for which parametrization is not included in equation 17.
Nine substituents (Set A: H, Me, But, F, Cl, Br, I, CF3 and CN) were selected as a basic set for the following qualities143: (i) symmetry and simplicity, (ii) freedom from conformational effects, (iii) lack of a large resonance effect, (iv) lack of any marked tendency to form hydrogen-bonds with the reaction centre. It proved possible to expand the list from 9 to 18 by making reasonable assumptions about the conformations of certain substituents, thus enabling them to be placed on the R and scales [Set B: Set A C Et, Pri, OMe, OEt, OPh, SMe, SO2Me, CH2Ph, (CH2)2Ph]. Correlations based on Set B turned out to be superior to those based on Set A.
The regression equations were established for data in 11 alcohols as solvents and were used to assess the peculiar behaviour of another 15 ortho-substituents in respect of conformational effect and intramolecular hydrogen-bonding143,145. Here we are concerned with assessing the situation for o-NO2. We first give as an example the regression for 2-methoxyethanol as solvent:
log k D 1.624 I |
C 0.964 R |
C 0.346 0.305 |
(18) |
(š0.074) |
(š0.082) |
(š0.060) |
|
n D 18, R D 0.990, s D 0.070
The regression coefficients are positive for the I and R terms because electron-attrac- ting groups accelerate the reaction and electron-releasing groups retard it. The positive regression coefficient for the term corresponds to the reaction being subject to steric acceleration by ortho-substituents through deconjugation of COOH with the benzene ring; cf Section IV.B.
For the nitro group there are two sets of substituent parameters: I D 0.67, R D 0.10 and D 1.39 for the group when coplanar with the benzene ring, and I D 0.67, R D 0.0 and D 0.35 for the group when orthogonal to the benzene ring. Thus the inductive effect is considered to be unaffected by twisting the nitro group, the resonance effect is eliminated by twisting to the orthogonal conformation and the steric effect is much greater for the coplanar conformation than for the orthogonal. We may now substitute these parameters in equation 18 and analogous equations for the reactions in the other solvents to obtain values of log k(calc, coplanar) and log k(calc, orthogonal), which may be compared with log k(obs). For equation 18, log k(calc, coplanar) D 1.360 and log k(calc, orthogonal) D 0.904. Log k(obs) D 0.994, i.e. between the values calculated for the two conformations and somewhat closer to the value for the orthogonal conformation. Without quoting the regression equations143,146, we give the results of similar calculations for the reactions in various alcohols [solvent, log k(calc, coplanar), log k(calc, orthogonal), log k(obs)]: methanol, 1.887, 1.321, 1.578; ethanol, 1.570, 1.006, 1.230; 2-methylbutan-2-ol, 1.056, 0.499, 0.734: benzyl alcohol, 2.410, 1.935, 2.170. In every case the observed value lies between the two extremes, indicating that an intermediate conformation of the nitro group is preferred. Thus both COOH and NO2 groups are somewhat twisted out of the plane of the benzene ring, as has been found for the configuration of this acid in the solid state147. The correlation analyses for the reactions in aprotic solvents also indicate an intermediate conformation for the nitro group144,146. All these results contrast with indications of an essentially orthogonal nitro group for the ionization of o-nitrobenzoic acid in aqueous solution (Section IV.B).
It may be mentioned in passing that Chapman and coworkers148 determined rate coefficients for the reactions at 30 °C of phenylacetic acid and various meta- or para-substituted phenylacetic acids with DDM in 10 alcohols as solvents. The nitro acids were included. There were some interesting indications of solvent dependence for the apparent values of para-substituents, including NO2, for which values ranging from 0.69 to 0.77 were
11. Electronic effects of nitro, nitroso, amino and related groups |
503 |
obtained. In further work the reactions of ortho-substituted phenylacetic acids with DDM were studied149, although not so great a variety of substituents was used as in the later work with benzoic acids143,144. The results for the o-alkyl groups formed the basis of a separtion of polar and steric effects for o-hal and o-NO2. The essence of the treatment lay in considering the differential action of the solvent on the positive and the negative poles of the substituents in the various positions of the ring. The treatment was claimed to be reasonably successful in accounting for the substituent effects in alcohols of a wide range of polarity. However, the form of treatment has never been pursued further by the authors or apparently by anyone else.
V. SUBSTITUENT CONSTANTS OF THE NITRO GROUP FROM THE APPLICATION OF MODERN EXPERIMENTAL AND THEORETICAL TECHNIQUES
A. Experimental Techniques
The emphasis in the foregoing parts of this chapter has been deliberately ‘chemical’. We have tried to explore the role of substituent constants in relation to understanding the effect of structure on reaction rates and equilibria, with particular reference to the NO2 group as a substituent. This chemical emphasis will continue in the later parts of the chapter, for NO2 and for the other substituents with which we are concerned, but in the present section there will be a change. In Section II.B brief reference was made to the use of substituent constants in the correlation analysis of spectroscopic data, particularly 19F and 13C substituent chemical shifts and infrared frequencies and intensities. These matters must now be explored in greater detail.
Attempts were made to apply Hammett benzoic acid-based m and p constants to the correlation analysis of spectroscopic data. Some significant correlations were obtained, but many of the correlations were rather poor, trends rather than precise relationships. Success in this area was found to involve the separation of inductive and resonance effects and the application of the dual substituent-parameter (DSP) equation (Section II.B). Indeed the development of the DSP equation became closely connected with the correlation analysis of 19F NMR shielding of substituted fluorobenzenes at an early stage, around 1957106. I and R0 were applied extensively to 19F NMR data90, and within a few years the correlations were being used to investigate ‘the effect of solvent on the inductive order’96, and ‘the effect of structure and solvent on resonance effects’97. New I andR0 values were based on the correlations. What happened with 19F NMR set a pattern
which was followed by later work. Established I and R0 values for substituents which were expected to be ‘well-behaved’ were used to set up regression equations. In the very early days the established substituent constants were all based on chemical reactivity (rate constants or equilibrium constants). 19F NMR data for groups for which no appropriate substituent constants were available were then substituted into the regression equations to obtain ‘19F NMR-based values’ of the substituent constants. Further, for the substituents which had been used to establish the regression equations, back-calculation from the NMR data led to ‘19F NMR-based values’ for those substituents as well. Thus for many substituents both ‘reactivity-based’ and ‘19F NMR-based’ values of I and R0 became available. For certain substituents there was a proliferation of values based on reactivity under various conditions or on 19F NMR in different solvents. Slightly later, correlation analysis of infrared data led in particular to new R0 values and, to a lesser extent, new I values, which were described as ‘IR-based’100,101. Somewhat later the same development occurred in connection with 13C NMR, leading to ‘13C NMR-based’ values98,99.
504 John Shorter
The NO2 group played a part in these developments. Thus NO2 was characterized by Taft87,89 with a I value of 0.63, based on the appropriate Ł value in his analysis of reactivities in ester reactions. The application of 19F NMR found a I value for NO2 of 0.56 in ‘normal solvents’, 0.49 in dioxane, 0.60 in ‘weakly protonic solvents’ and 0.80 in trifluoroacetic acid96. The last-mentioned high value was attributed to the effect of hydrogen-bonding of NO2 to CF3COOH, but with this exception all the 19F NMR-based values lie considerably below the reactivity value of 0.63, which agrees moderately well with Charton’s reactivity value of 0.67 (Section III.A)109. ‘Normal solvents’ included cyclohexane, benzene, carbon tetrachloride and other more polar solvents, while the principal ‘weakly protonic solvents’ were methanol and formic acid. The above figures appear to give some indication of slight enhancement of the CI of NO2 by hydrogen-bonding to ‘weakly protonic solvents’. The very low value for I in dioxane finds a parallel in the behaviour of I for other electron-attracting groups (e.g. CN and COMe) and was attributed to Lewis acid-bonding of dioxane to the substituent98. A recent compilation of substituent constants gives a 19F NMR-based value for NO2 of 0.64 from a chemical shift determined in dilute solution in hydrocarbon solvent150; cf 0.56 above. The increase of 0.08 may be due to using a slightly different regression equation. (The application of this equation to the chemical shift data for ‘weakly protonic solvents’ would give a I value of 0.65, in good agreement with the reactivity-based values above.) The I value recommended in 1973 for NO2 by Ehrenson, Brownlee and Taft92 was 0.65, while a recent tabulation151 gives the equivalent F value (see below) also as 0.65.
A R0 value of 0.15 was given by Ehrenson, Brownlee and Taft92. Simple subtraction of the recommended I value of 0.65 from the p0 value of 0.82 given by Taft152 would have yielded 0.17. A slightly lower value of p0 was favoured by Yukawa, Tsuno and Sawada153,
0.80 to 0.81. We may take 0.15 as a fair estimate of R0 based on the reactivity data available in the early 1970s. It is slightly higher than any value based on the assumption that for NO2 there is no valid distinction between p and p0 (see Section III.C), e.g. Charton’s value 0.10 for R, which was calculated by substracting I = 0.67 from p D 0.77109, 19F NMR studies shed no direct light on R0 for NO2, because cross-conjugation between F and NO2 in p-fluoronitrobenzene leads to enhancement of the CR effect of NO2 (Section II.A). It is only for R substituents that 19F NMR studies yield R0 values. The application of the 19F NMR method to CR substituents yields ‘apparent’ values, which may be designated N R56. For NO2 the value of NR is about 0.2 from measurements in a wide range of supposedly indifferent solvents97. As in the case of I, NR is increased by certain solvents. Thus in trifluoroacetic acid N R ³ 0.30. Presumably hydrogen-bonding enhances both CI and CR effects. Measurements of infrared intensities do, however, give information about R0 constants for CR substituents. Katritzky and colleagues154 give a R0 value of 0.174 for NO2 based on measurements with a solution of nitrobenzene in carbon tetrachloride. (Note: the sign of R0 is not actually given by the correlation of infrared intensities, because the square of R0 is involved. The sign can usually be inferred from other knowledge about the substituent.) The infrared value is thus somewhat higher than Charton’s reactivity-based value of 0.10109.
There is a continual tendency for the values of I and R0 (and other R-type constants) to be adjusted in the light of new measurements. Thus measurements in 1979155 of para13C substituent chemical shifts for a series of mono-substituted benzenes in very dilute solution in cyclohexane, carbon tetrachloride or deuteriochloroform were the basis for a redefinition of the R0 scale and some amendment of R0 values. However, the value for the nitro group was confirmed as 0.15.
11. Electronic effects of nitro, nitroso, amino and related groups |
505 |
Reynolds and coworkers156 based a similar operation on 13C substituent chemical shifts of meta- and para-substituted styrenes. Iterative multiple regression was used for the redefinition of the I and R0 scales. The authors also took the opportunity to replace the symbol I by F, having become convinced that the so-called inductive effect was entirely a field effect (see the present author’s discussion of this matter76). The authors presented an extensive table in which their values of the substituent parameters are compared with those obtained by other authors. Their R0 value of 0.144 for NO2 essentially confirmed the various values of about 0.15 already mentioned and their I value of 0.651 essentially confirmed the various values of about 0.65.
Happer157 determined 13C substituent chemical shifts for meta- and para-substituted styrenes in seven different solvents. Data for the side-chain carbons, and in the meta series for the ring carbon para to the substituent, were analyzed as a basis for assessing solvent effects on I, R0, R (BA) and R . The I values for NO2 varied from 0.60 in DMSO to 0.71 in CDCl3; in EtOH the value was 0.64. The R0 values for NO2 varied from 0.08 in CCl4 to 0.10 in benzene and in EtOH. Some doubts were, however, expressed regarding the reliability of the R0 values for CN, NO2 and CF3.
The influence of solvent on the inductive order of substituents was studied by Laurence and collaborators through infrared measurements on 4-substituted camphors158. From these Laurence159 has tabulated new F values applicable to solutions in carbon tetrachloride or other solvents of low dielectric constant, NO2 came out at 0.68.
Mention must also be made of the use of studies of chemical reactions in the gas phase as a means of determining substituent constants. The investigation of substituent effects and linear free-energy relationships in the gas phase has become an enormous subject with which we can deal only briefly. Part of this subject was established a long time ago and consists in the study of such reactions as the pyrolysis of esters by the techniques of gas kinetics (see the review by Smith and Kelly160). One purpose of such work is to see how far substituent constants based on processes in solution may be applied successfully in the gas phase. This leads to the possibility of determining substituent constants in the complete absence of solvent. Work of this nature continues today; see the recent review by Holbrook in this Series161, which updates the earlier review by Taylor162. However, the use of NO2 as a substituent in such gas-phase studies is fairly rare, presumably because the group often tends to get involved chemically in the reactions. CN is to be preferred as a strongly electron-attracting substituent.
The major activity in gas-phase studies now depends on the use of modern techniques such as ion cyclotron resonance (ICR). Thus, as already mentioned (Section III.D). Fujio, McIver and Taft131 measured the gas-phase acidities, relative to phenol, of 38 meta- or para-substituted phenols by the ICR equilibrium constant method, and their results for CR substituents led them to suggest that such substituents in aqueous solution exerted solvation-assisted resonance effects. It was later163 shown by comparison of gas-phase acidities of phenols with acidities of phenols in solution in DMSO that solvation-assisted resonance effects could also occur even when the solvent did not have hydrogen-bond donor properties. Indeed for p-NO2 and certain other substituents these effects appeared to be larger than in aqueous solution.
Taft and Topsom151 have fairly recently written an extensive review of the electronic effects of substituents in the gas phase. This article includes a tabulation of substituent inductive and resonance parameters. The inductive parameters (designated F) are based on measured spectroscopic properties in either the gas phase or in hydrocarbon or similar solvents. The resonance parameters were arrived at through the treatment of 38 gas-phase reactivity series by iterative multiple regression, using the R0 values of Bromilow and coworkers155 as the starting point. The F value for NO2 was found to be 0.65 (quoted
506 |
John Shorter |
above), while the resonance parameter is given as 0.18. The column heading in Taft and Topsom’s table is simply R, but inspection shows that the values must be regarded as being those of R0, when the distinction matters, i.e. with R substituents. The value of 0.18 for the resonance parameter of NO2 is slightly higher than any value previously suggested.
B. Theoretical Techniques
The application of ab initio molecular orbital theory to suitable model systems has led to theoretical scales of substituent parameters, which may be compared with the experimental scales. Calculations (3-21G or 4-31G level) of energies or electron populations were made by Marriott and Topsom in 1984164. The results are well correlated with F (i.e. I) for a small number of substituents whose F values on the various experimental scales (gas phase, non-polar solvents, polar solvents) are concordant. The nitro group is considered to be one of these, with values 0.65 in the gas phase, 0.65 in non-polar solvents and 0.67 in polar solvents. The regression equations are the basis of theoretical F values for about fifty substituents. The nitro group is well behaved and the derived theoretical value ofF is 0.66.
A theoretical scale of substituent resonance effects was based on calculations of electron populations in substituted ethylenes165. A suitable regression equation was again set up by using standard substituents, but in this case the quantum-mechanical quantity was correlated with infrared-based R0 values. The equation was the basis of theoretical R0 values for more than forty substituents. Here again it was possible to include the data for NO2 in the setting up of the regression equation. The theoretical value of R0 came out as 0.19, just slightly larger than the experimental value which had been used, namely 0.17. A further redefinition of the theoretical scale was made fairly recently166 as a result of a change of views as to the most suitable level of MO approximation. In the latest versionR0 comes out at 0.12, the literature value of 0.17 having again been used in setting up the regression equation. Thus the new theoretical value is rather closer to Happer’s values from 13C NMR and to Charton’s reactivity-based value (see Section V.A). Other recent theoretical papers by Topsom in which NO2 is featured are on a scale of variable -electron transfer167, the influence of water on substituent field effects168 (the theoretical F value for NO2 rises to 0.74), the influence of water on substituent resonance effects169 (the value of R0 for NO2 rises from 0.12 to 0.14), the acidities of ortho-substituted phenols170 and theoretical studies of the effects of hydration on organic equilibria171. There is also an extensive review of theoretical studies of electronic substituent effects172. It seems rash, however, to regard theoretical substituent parameters as in any way replacing those founded on experimental results.
Fairly recently there have been various other theoretical treatments of substituent effects, e.g. the correlation analysis of substituent effects on the acidity of benzoic acid by the AM1 method173 and direct prediction of linear free-energy substituent effects from 3D structures using comparative molecular field analysis, the relevant data set being 49 substituted benzoic acids174. Very recently Russian workers have presented a new model for the inductive effect, in an extremely detailed communication in three parts175. The approach appears to be very successful in rationalizing a large amount of relevant experimental data.
Although the term ‘theoretical techniques’ in relation to electronic effects may commonly be taken to refer to quantum-mechanical methods, it is appropriate also to mention the application of chemometric procedures to the analysis of large data matrices. This is in a way complementary to analysis through substituent constants based on taking certain systems as standards and applying simple or multiple linear regression. Chemometrics involves the analysis of suitable data matrices through elaborate statistical procedures,
11. Electronic effects of nitro, nitroso, amino and related groups |
507 |
such as principal component analysis and factor analysis. The parameters characterizing substituents and processes emerge from the data analysis. As an example of this kind of work, the recent contributions of the group of Ludwig and Pytela will be mentioned briefly. Reference has already been made to the earlier work of these authors in determining the dissociation constants of a large number of substituted benzoic acids in different solvents (Section III.B)120,121. The results were treated as a data matrix by the chemometric methods referred to above, and various sets of Hammett values were derived for the 35 substituents involved. For the nitro group and other electron-attracting substituents, the values derived by principal component analysis were lower than those derived by factor analysis by several units in the second place of decimals. More recently these authors have augmented their own experimental results with other information from the literature to construct a data matrix of 46 sets of measurements on substituted benzoic acids in various solvents; a total of 51 m- or p-substituents was involved176,177. ‘Optimized’ values were tabulated177, those for the nitro group being 0.717 for m and 0.780 for p.
Other papers in the series ‘Chemometrical Analysis of Substituent Effects’ are on additivity of substituent effects in dissociation of 3,4-178 or 3,5-179disubstituted benzoic acids in organic solvents and on the ortho-effect180. In the last-mentioned, data for the dissociation of ortho-substituted benzoic acids in 23 solvents are combined with data on the reactions with DDM (Section IV.C) and with other rate and equilibrium data bearing on the behaviour of ortho-substituents to form a matrix involving data for 69 processes and 29 substituents.
VI. ELECTRONIC EFFECTS OF NO2 ON VARIOUS SYSTEMS AND
PROCESSES A. Formation and Stabilization of Carbanions
1. Introduction
The Historical Introduction (Section I.B) showed how the activation of the hydrogen atom(s) on the carbon atom to which NO2 is attached was recognized at an early stage to be associated with incipient acidity. Reaction mechanisms essentially involving carbanions were proposed by Lapworth27 early in the 20th century and were gradually accepted by organic chemists over the next 30 to 40 years. By the 1930s some ‘stable’ carbanions, as opposed to highly reactive intermediates, were recognized, e.g. the behaviour of dinitromethane and nitroform as acids was known.
In the last half-century the pKa values of thousands of carbon acids have been measured in a variety of solvents. In many such acids the ionization is stimulated by the NO2 group, sometimes in association with other CI, CR groups, such as CN, COR or COOR. There have also been many studies of the kinetic acidities of carbon acids and of the mechanisms of reactions involving carbanion intermediates; again, in many cases NO2 has played an important role. Clearly a comprehensive survey of NO2-promoted C H acidity is not feasible here, and it will be necessary to concentrate on outlining a few main topics. The role of NO2 in increasing C H equilibrium acidity will be illustrated with a few structural series, some studies of kinetic acidity will be outlined, and developments in the correlation analysis of C H acidity and in the understanding of the stabilization of carbanions by NO2 and related groups will be discussed. Reactions of aliphatic systems depending on the activation of C H bonds by NO2 were surveyed in great detail in a volume of this Series in 1970, including over five hundred references22. Later developments may be followed by means of the annual Series Organic Reaction Mechanisms181.
508 |
John Shorter |
2. Equilibrium acidities of nitrocarbon acids
Many pKa values for nitrocarbon acids are in the compilations of Palm and colleagues182. A comprehensive study of the ionization of many carbon acids in DMSO has been made by Bordwell and colleagues and many of them are nitro compounds183.
The pKa value of methane in water has been estimated as about 40 and that of CH3NO2 has been determined as 10.2. Further substitution of H by NO2 leads to pKa values of 3.6 for CH2(NO2)2 and 0.2 for CH(NO2)3. The values for the corresponding cyanocarbon acids are about 25, and 11.2 and 5.1 respectively. In the cyanocarbon series the effect of successive substitution shows an extraordinary degree of additivity, in spite of the mutual proximity of the cyano groups. This is probably connected with the small size of CN and the linearity of C C N. The larger and triangular nitro groups clearly interfere with each other, so that there is no additivity in the effect of successive substitution. In the result, whereas one nitro group is more acid-strengthening than one cyano group, three nitro groups are considerably less effective than three cyano groups.
The series RCH2NO2 presents some interesting results for the effect of different groups R on pKa: H, 10.2; Me, 8.6; Cl, 7.2; COOMe, 5.65; CN, 4.9; NO2, 3.6; Ph, 6.9. The position of the Me point with respect to the ‘parent’ H point seems anomalous, since the normal inductive effect of the methyl group would be expected to diminish rather than enhance acidity. This anomalous effect of ˛-methyl substitution continues with a further methyl group, for the pKa value of Me2CHNO2 is 7.75. The effects of lengthening and branching of an alkyl chain R in RCH2NO2 also show some irregularities: Et, 8.98; Pr, 8.86; Pri, 9.21; Bui, 8.56.
NO2 may also feature as a substituent influencing C H acidity from a more remote site. Thus for ring substitution in the series PhCH(NO2)2, the pKa values for the m- and p- nitro compounds are 2.82 and 2.63, respectively, compared with 3.89 for the unsubstituted compound (values in water, 20 °C). The value for this reaction series is about 1.7, and these results for m- and p-NO2 appear to indicate that ordinary Hammett values are applicable, whereas values might have been expected to be more relevant. Presumably this means that the delocalization of the negative charge in the carbanion is dominated by the (NO2)2 groups, so that delocalization into the ring plays a minor role.
There are many pKa data for the series RCH(NO2)2, which may be correlated with
I or Ł184 (Section II.B.). Certain R systems show deviations which may be discussed in terms of the occurrence of steric and other effects. Jones184 presents various other interesting structural series of nitrocarbons.
3. Kinetic acidities of nitrocarbon acids
Various types of reaction of carbon acids are believed to proceed via dehydronation (commonly referred to as deprotonation)185, notably halogenation in the presence of bases, and the rates of such processes are a measure of the kinetic acidities of the carbon acids. Extensive data of this nature exist, including some for nitrocarbon acids182. In recent years kinetic acidity has also been studied through the detritiation of appropriately tritiated compounds. The existence also of equilibrium acidity data under the same conditions permits rate-equilibrium correlations of the Brønsted type186, as well as Hammett treatments in appropriate cases. Studies of the rates of reaction of a given substrate with a series of bases also generate Brønsted correlations, and in appropriate cases also Hammett correlations.
Recent studies of kinetic acidity involving nitrocarbon acids have included the following: benzoate ion-promoted deprotonation of (3- or 4-nitrophenyl)nitromethane and (3,5-dinitrophenyl)nitromethane187; the deprotonation by various bases of a series of