
Principles and Applications of Asymmetric Synthesis
.pdf
372 ASYMMETRIC CATALYTIC HYDROGENATION AND OTHER REDUCTION REACTIONS
Scheme 6±43. Reprinted with permission by Elsevier Science Ltd., Ref. 94.
Scheme 6±44. R ˆ Ph, Bn, substituted Ph, i-Pr, i-Bu, and c-Hexyl.
In summary, many attempts have been made at achieving enantioselective reduction of ketones. Modi®ed lithium aluminum hydride as well as the oxazaborolidine approach have proved to be very successful. Asymmetric hydrogenation catalyzed by a chiral ligand-coordinated transition metal complex also gives good results. Figure 6±7 lists some of the most useful chiral compounds relevant to the enantioselective reduction of prochiral ketones, and interested readers may ®nd the corresponding applications in a number of review articles.77,96,97
Figure 6±7. Useful compounds for ketone reduction.
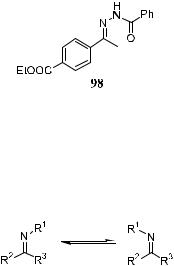
6.3 ASYMMETRIC REDUCTION OF IMINES |
373 |
6.3ASYMMETRIC REDUCTION OF IMINES
Preparation of enantiomerically pure secondary amines by catalytic asymmetric hydrogenation or hydrosilylation of imines is as important as the preparation of alcohols from ketones. However, asymmetric hydrogenation of prochiral CbN double bonds has received relatively less attention despite the obvious preparative potential of this process.98
It has been reported that the hydrogenation of imine ArC(Me)bNCH2Ph proceeds with enantioselectivity of up to 96% when Rh(I)-sulfonated BDPP is used in a two-phase system. However, the asymmetric reaction of CbN bonds with ruthenium(II) catalyst is rather rare.99 Willoughby and Buchwald100 demonstrated a titanocene catalyst that shows good to excellent enantioselectivity in the hydrogenation of imine.
Several successful results have been obtained in the asymmetric hydrogenation and asymmetric hydrosilylation of imines.101 An e½cient enantioselective hydrogenation of the CbN double bond was developed by Burk and Feaster,101a who used [Rh(COD)(DuPhos)]‡CF3SO3ÿ in the hydrogenation of N- aroylhydrazone 98.
One problem for the asymmetric hydrogenation of imine is (E )/(Z) isomerism of the substrate, which may have a signi®cant e¨ect on the enantioselectivity of the reaction. This problem was di½cult to address because of the rapid interconversion of the E and Z isomers of the imines under reaction conditions (Fig. 6±8).
Figure 6±8. Interconversion of (Z)/(E) isomers of an imine.
Becalski et al.98b found that the (E )/(Z) ratio of imines under rhodiumcatalyzed hydrogenation conditions stayed essentially constant during the course of the reaction. This observation was explained in terms of two possibilities: (1) the rates of hydrogenation of the two isomers were identical; or, more likely, (2) the rate of interconversion of the two isomers was faster than the rate of hydrogenation, leaving the (E )/(Z) ratio of the substrate constant.
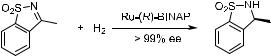
374 ASYMMETRIC CATALYTIC HYDROGENATION AND OTHER REDUCTION REACTIONS
Scheme 6±45
When the CbN bond is ®xed in a ring system in which no (E )/(Z) isomerization can take place, the asymmetric hydrogenation of the CbN bond can be highly enantioselective. Oppolzer et al.99 found that cyclic sulfonimide was hydrogenated with an Ru(BINAP) catalyst to give a product with essentially quantitative optical yield (Scheme 6±45).
Similar success was also achieved by Willoughby and Buchwald100a with a chiral titanocene catalyst. The high ee obtained by Burk and Feaster101a in the asymmetric hydrogenation of 98 was also consistent with the preferred coordination of one isomer forced by the bidentate chelation of the hydrazones.
To investigate the e¨ect of (E )/(Z) isomerization of CbN bonds on asymmetric hydrogenation, Chan et al.98g chose to study the asymmetric hydrogenation of (E ) and (Z) isomers of oximes. The advantage of oximes is that the (E ) and (Z) isomers may be isolated, separated, and unambiguously characterized. By choosing suitable substrates, the e¨ect of (E )/(Z) isomerism on enantioselectivity can be clearly established. A comparison of the asymmetric hydrogenation of (E )- and (Z)-1-acetophenone oxime showed a signi®cant (E )/ (Z) isomeric e¨ect. For example, with fRh(NBD)[(S)-BINAP]gBF4 used as a catalyst precursor, the product ee was 66% for the (Z) isomer and only 30% for the (E ) isomer. Both the (Z) and (E ) isomers gave predominantly the product with (S)-con®guration. When fRh(NBD)[…R;R†-SkewPhos]gBF4 was used, the (Z) isomer gave a product in 35% ee in (R)-con®guration, while the (E ) isomer gave 12% ee in the opposite con®guration. These results reveal the importance of (E )/(Z) isomerism in the asymmetric hydrogenation of CbN bonds.
Hydrosilylation of imine compounds was also an e½cient method to prepare amines. The hydrosilylation product N-silylamines can readily be desilylated upon methanol or water treatment, yielding the corresponding amines. The amines can be converted to their corresponding amides by subsequent acyl anhydride treatment. The ®rst attempt to hydrogenate prochiral imines with Rh(I) chiral phosphine catalysts was made by Kagan102 and others. These catalysts exhibited low catalytic activity, and only moderate ee was obtained.
Particularly noteworthy is the discovery of a new type of the active catalyst 99,103,104 a crystalline, air-stable yellow-orange solid, which can serve as a highly enantioselective tool in the titanium-catalyzed hydrosilylation of imines. The reaction can be highly stereoselective for both acyclic and cyclic imines under a wide range of hydrogen pressures (Scheme 6±46).
Treatment of this precatalyst with phenylsilane yields a very active catalytic system that can be used for the hydrosilylation of imines. This discovery was initiated by the observed hydrosilylation of imines when phenylsilane was added to Cp2TiF2. It was presumed that it might be possible to break the strong

6.3 ASYMMETRIC REDUCTION OF IMINES |
375 |
Scheme 6±46. Titanocene-catalyzed asymmetric hydrosilylation of imines.
Ti±F bond and generate a Ti±H species when 99 was treated with phenylsilane. The chirality transfer may take place through imine insertion into the Ti±H bond, similar to that in the catalytic hydrogenation process.100c The reaction can be carried out by the subsequent addition of imines. The corresponding silylated amines can be obtained and further converted to enantiomerically enriched amines upon acid treatment. For example, in the presence of 99, N- methylimine 100 undergoes complete hydrosilylation within 12 hours at room temperature, with 97% ee and up to 5000 turnovers.103
The important feature of this reaction system is its experimental simplicity.105 Although hydrogenation requires elevated pressure and high temperature, this reaction can be carried out at room temperature in an inert atmosphere. The catalyst can be activated before the addition of the substrate or, even more conveniently, in the presence of the substrate. After completion of the reaction and the subsequent acidic workup, the secondary amine product can be obtained in high yield and purity as well as very high enantioselectivity.
The drawback of this reaction is its extremely high sensitivity to the steric bulk of the nitrogen substitutent. When the analogous N-benzylimine 101 was subjected to the standard hydrosilylation conditions, the reaction gave 55% conversion and 47% ee with prolonged reaction time (96 hours). A practical way to overcome this problem is to employ a nucleophilic promoter to convert the intermediate into a more reactive species. In this case, it has been found that primary amines have the most pronounced e¨ect on the reaction. For example,
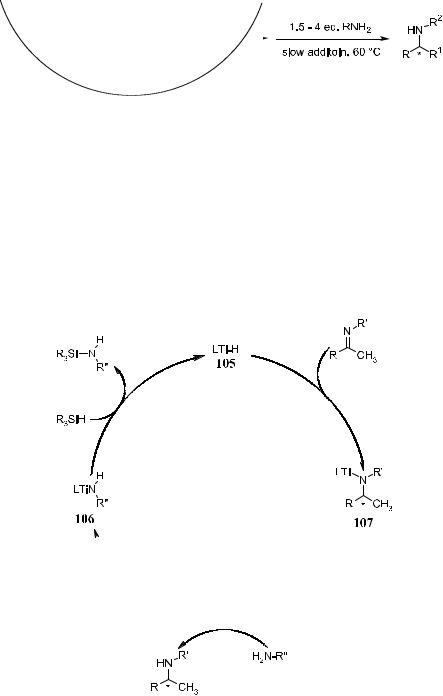
376 ASYMMETRIC CATALYTIC HYDROGENATION AND OTHER REDUCTION REACTIONS
Scheme 6±47
slow addition of four equivalents of n-hexylamine to the hydrosilylation reaction mixture at 60 C results in the complete reduction of 102 within 2 hours (85% ee, Scheme 6±47).
Generally, amine products can be obtained with 91±99% ee. It is noteworthy that the ee of the product does not correspond to the E:Z ratio of the starting imines. Imines 103 and 104 exist as 2.5:1 and 1.8:1 (E )/(Z) isomers, but titanocene-catalyzed reduction produces amines with 93% and 97% ee, respectively.105
A possible explanation for this e¨ect of added amine is shown in Figure 6±9.105
Figure 6±9. E¨ect of added amine on imine hydrosilylation.
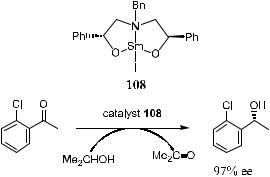
6.4 ASYMMETRIC TRANSFER HYDROGENATION |
377 |
The added primary amine may facilitate the cleavage of the Ti±N bond of the key intermediate 107 through the coordination to the titanium center followed by s-bond metathesis. Such an intramolecular exchange process is expected to be facile. The amine exchange product is 106, which can then be rapidly converted to 105 and the corresponding silylated amine to complete the catalytic cycle.
6.4ASYMMETRIC TRANSFER HYDROGENATION
The Meerwein-Ponndorf-Verley reaction is a classic method for ketone/ aldehyde carbonyl group reduction, which involves at least 1 equivalent of aluminum alkoxide as a promoter. In this reaction, the hydrogen is transferred from isopropanol to the ketone/aldehyde substrate, so the reaction can also be referred to as a transfer hydrogenation reaction.
Evans et al.106 report an asymmetric transfer hydrogenation of ketones using samarium(III) complex (108) as the catalyst at ambient temperature in 2- propanol. The products showed ee comparable with those obtained through enantioselective borane reduction (Scheme 6±48).
Scheme 6±48. Meerwein-Ponndorf-Verley reaction.
Increasing e¨ort has been applied to develope asymmetric transfer hydrogenations for reducing ketones to alcohols because the reaction is simple to perform and does not require the use of reactive metal hydrides or hydrogen. Ruthenium-catalyzed hydrogen transfer from 2-propanol to ketones is an e½cient method for the preparation of secondary alcohols.
Chowdhury and BaÈckvall107 showed that RuCl2(PPh3)3 was an e¨ective catalyst for transfer hydrogenation in the presence of 2% NaOH. This ®nding initiated the development of asymmetric processes that utilized chiral
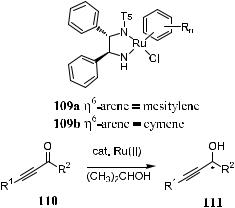
378 ASYMMETRIC CATALYTIC HYDROGENATION AND OTHER REDUCTION REACTIONS
ligand-modi®ed transition metal complexes as catalysts to achieve high stereoselectivity.108
Chiral propargylic alcohols are useful building blocks for the synthesis of various biologically active and structurally interesting compounds. These alcohols can be prepared by stoichiometric asymmetric reduction of the corresponding acetylenic ketones with chiral metal hydrides (Section 6.2) or through other asymmetric synthesis methods.109 Noyori and co-workers report that this conversion can also be realized via asymmetric transfer hydrogenation using chiral Ru(II) complex as the catalyst and 2-propanol as the hydrogen donor.110 Compound 109 bearing a chiral diamine derivative as ligand has been demonstrated to be an e½cient and highly enantioselective catalyst (Scheme 6±49).
Scheme 6±49
With 109a or 109b, the reaction proceeds smoothly to provide the corresponding alkynyl alcohol with consistently high ee (up to 99%). In the presence of bulky R2 substituents, the substrate can undergo transfer hydrogenation under neutral conditions at room temperature, with an S/C molar ratio of 100 to 200, or even higher. The ee values are very high regardless of the bulkiness of the R2 substituent, varying from a methyl to a t-butyl group.
Furthermore, asymmetric transfer hydrogenation proceeds equally well when there is a preexisting stereogenic center in the acetylenic ketone. The stereoselectivity is not in¯uenced by this preexisting stereogenic center. For example, transfer hydrogenation of compound (S)-112 catatyzed by …R;R†-109a gives …3S;4S†-113 with 99% ee and over 97% yield. Similarly, the …S;S†-109a± catalyzed reduction of (S)-112 gives over 97% yield of …3R;4S†-113 in more than 99% ee (Scheme 6±50). The adjacent nitrogen-substituted stereogenic center does not interfere with the stereoselectivity of the reaction.
Complex 109 can also be used for the asymmetric transfer hydrogenation
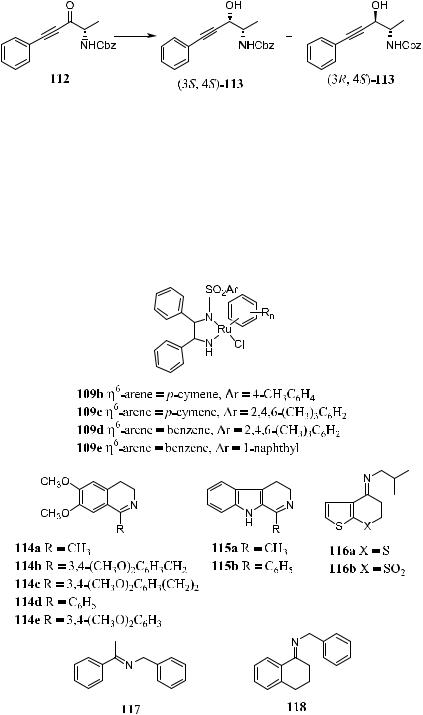
6.4 ASYMMETRIC TRANSFER HYDROGENATION |
379 |
||||||
|
|
|
|
|
|
|
|
|
|
|
|
|
|
|
|
|
|
|
|
|
|
|
|
Scheme 6±50
of imines, providing the corresponding chiral amines in high enantiomeric excess.111 The reaction can be carried out in various aprotic solvents such as DMF, DMSO, or CH2Cl2, but not in ethereal or alcoholic solvents. Reaction in neat formic acid/triethyl amine is very slow. Table 6±10 presents the results of the transfer hydrogenation of a variety of imines in the presence of an HCO2H±Et3N mixture.

380
TABLE 6±10. Asymmetric Transfer Hydrogenation of a Variety of Imines
Entry |
Imine |
Catalyst |
S/C |
Solvent |
Time (h) |
Yield (%) |
ee (%) |
Con®g. |
|
|
|
|
|
|
|
|
|
1 |
114a |
(S,S)-109b |
200 |
CH3CN |
3 |
>99 |
95 |
R |
2 |
114b |
(R,R)-109c |
200 |
DMF |
7 |
90 |
95 |
S |
3 |
114c |
(R,R)-109c |
200 |
CH2Cl2 |
12 |
99 |
92 |
S |
4 |
114d |
(S,S)-109e |
200 |
CH2Cl2 |
8 |
99 |
84 |
R |
5 |
114e |
(R,R)-109e |
100 |
CH2Cl2 |
12 |
>99 |
84 |
S |
6 |
115a |
(S,S)-109b |
200 |
DMF |
5 |
86 |
97 |
R |
7 |
115b |
(S,S)-109b |
200 |
DMF |
5 |
83 |
96 |
R |
8 |
116a |
(S,S)-109e |
100 |
CH3CN |
12 |
82 |
85 |
S |
9 |
116b |
(S,S)-109e |
100 |
CH3CN |
5 |
84 |
88 |
S |
10 |
117 |
(S,S)-109d |
200 |
CH2Cl2 |
36 |
72 |
77 |
S |
11 |
118 |
(S,S)-109e |
100 |
CH2Cl2 |
6 |
90 |
89 |
S |
|
|
|
|
|
|
|
|
|
ee ˆ Enantiomeric excess.
Reprinted with permission by Am. Chem. Soc., Ref. 111.

|
|
6.4 ASYMMETRIC TRANSFER HYDROGENATION |
381 |
||||||||||
|
|
|
|
|
|
|
|
|
|
|
|
|
|
|
|
|
|
|
|
|
|
|
|
|
|
|
|
|
|
|
|
|
|
|
|
|
|
|
|
|
|
|
|
|
|
|
|
|
|
|
|
|
|
|
|
|
|
|
|
|
|
|
|
|
|
|
|
|
|
|
|
|
|
|
|
|
|
|
|
|
|
|
|
|
|
|
|
|
|
|
|
|
|
|
|
|
|
Scheme 6±51. Asymmetric transfer hydrogenation of acetophenone in the presence of 119. Reprinted with permission by Am. Chem. Soc., Ref. 112.
As transfer hydrogenation uses inexpensive reagents and is usually easy to perform, the asymmetric transfer hydrogenation using 2-propanol as the hydrogen source o¨ers an attractive method for reducing unfunctionalized ketones to chiral alcohols. The chiral ligand used by Noyori et al. contains an NH moiety that may promote a cyclic transition state through hydrogen bonding to a ketone substrate. Therefore, it is desirable to design a chiral ligand that can form a metal±ligand bifunctional catalyst to increase the a½nity of the substrate to the active site of the catalyst and thus to increase the stereoselectivity.
Sammakia and Stangeland112 reported another type of chiral ligand 119 bearing a chiral oxazoline moiety and tested its asymmetric induction capability in transfer hydrogenation. As shown in Scheme 6±51, 90% or higher ee values were observed in all cases, along with high conversion of the substrates.
It is well recognized that chiral tridentate ligands generally form a deeper chiral cavity around the metal center than a bidentate ligand. For example, as mentioned in previous chapters, the chiral tridentate ligand Pybox 120 has been used in asymmetric aldol reactions (see Section 3.4.3) and asymmetric DielsAlder reactions (see Section 5.7). The two substituents on the oxazoline rings of 120 form a highly enantioselective chiral environment that can e¨ectively differentiate the prochiral faces of many substrates.
Jiang et al.113 synthesized another tridentate ligand 121 for asymmetric transfer hydrogenation. Ru±121-catalyzed asymmetric transfer hydrogenation gives comparable enantioselectivity to Noyori's catalyst 109 but shows more