
Principles and Applications of Asymmetric Synthesis
.pdf332 ASYMMETRIC CATALYTIC HYDROGENATION AND OTHER REDUCTION REACTIONS
The ®rst successful examples of homogeneous asymmetric hydrogenation were reported independently by HoÈrner et al.1 and Knowles and Sabacky2 in 1968. The Wilkinson compound and related complexes modi®ed by the incorporation of a chiral tertiary phosphine, such as P(C6H5)(n-C3H7)(CH3), catalyzed the hydrogenation of certain hydrocarbon ole®ns with an optical yield of 3±15%. Although the ee values of the hydrogenated products were not very high, the results established a solid foundation for developing the concept of homogeneous asymmetric hydrogenation.
A breakthrough in this area came when Dang and Kagan3 synthesized DIOP, a C2 chiral diphosphine obtained from tartaric acid (Fig. 6±1). DIOP±Rh(I) complex catalyzed the enantioselective hydrogenation of a-(acylamino)acrylic acids and esters to produce the corresponding amino acid derivatives with up to 80% ee. These achievements stimulated research on a variety of bidentate chiral diphosphines, and numerous chiral ligands bearing C2 symmetry have been developed as a result (see Fig. 6±1 for examples).
Thirty years have passed, and bisphosphines containing chiral substituents have proved to be the most useful and versatile ligands in organotransition metal±catalyzed reactions. The mechanism of Rh complex±catalyzed asymmetric hydrogenation was elucidated in detail by Chan and Halpern8 and Brown et al.9 The successful commercial application of Rh-catalyzed hydrogenation of prochiral enamides in the synthesis of l-DOPA10 made asymmetric catalytic hydrogenation a popular subject of research.
6.1.1 Chiral Phosphine Ligands for Homogeneous Asymmetric Catalytic Hydrogenation
Asymmetric catalytic hydrogenation is one of the most e½cient and convenient methods for preparing a wide range of enantiomerically pure compounds, and Ru-BINAP±catalyzed asymmetric hydrogenation of 2-arylacrylic acids has attracted a great deal of attention,11 as the chiral 2-arylpropionic acid products constitute an important class of nonsteroidal antiin¯ammatory drugs.
Historically, the desire for practical routes to a-amino acids ultimately led to the development of e¨ective chiral diphosphine rhodium catalysts for the enantioselective hydrogenation of a-amidoacrylates (a-enamides) (see Scheme 6±1).12 It had been found that many ligands with C2 symmetry were e¨ective in asymmetric hydrogenation reactions. DIOP is a ligand with two sp3 asymmetric carbons and a C2-symmetric axis, while DIPAMP possesses two asymmetric phosphorous atoms. The aromatic BINAP ligand possesses C2 axial chirality and has shown great asymmetric induction potential. It has been suggested that the highly skewed position of the naphthyl rings in BINAP is the determining factor in its e¨ectiveness in asymmetric catalytic reactions.13 Indeed, in most of the phosphine ligands, the linkage between the chiral part and the phosphine part is a C±P bond, while in some of the promising ligands C±O±P or C±N±P linkages are also involved.
Design of such diphosphine ligands remains an active area of research, and

6.1 INTRODUCTION 333
Figure 6±1. Selected ligands for catalytic asymmetric hydrogenation.
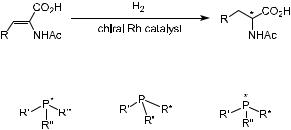
334 ASYMMETRIC CATALYTIC HYDROGENATION AND OTHER REDUCTION REACTIONS
Scheme 6±1
Figure 6±2. Possible types of chiral phosphine ligands.
the homogeneous asymmetric catalytic hydrogenation of prochiral CbX (X ˆ C, N, O, and so forth) double bonds is one of the most important applications of these enantioselective catalytic technologies. There are three ways to design a chiral phosphine. The chirality can be located on the P atom, on a side chain, or on both. Figure 6±2 depicts these three possible chiral phosphine ligands.
Much e¨ort has been devoted to investigating chiral phosphine ligands for their synthesis and asymmetric catalytic hydrogenation potential, and such chiral phosphine ligands have been extensively used for catalytic asymmetric hydrogenation, both academically and industrially.14
Numerous practical advantages are associated with asymmetric catalytic processes that allow the conversion of prochiral substrates to valuable enantiopure products, and homogeneous asymmetric hydrogenation constitutes one of the most e½cient and versatile methods for making such conversions. A variety of chiral compounds can be obtained via the catalytic asymmetric hydrogenation of CbC, CbN, and CbO bonds with outstanding levels of e½ciency and enantioselectivity. The yield and the enantiomeric excess of the product are in¯uenced not only by chiral ligands but also by counterions, by substrate-to- catalyst ratio and solvent, as well as by reaction time and reaction temperature. The following sections present some examples to show the in¯uence of these factors.
6.1.2Asymmetric Catalytic Hydrogenation of CFC Bonds
6.1.2.1Reaction Mechanisms for the Asymmetric Hydrogenation of Enamides. Over the past three decades, use of chiral catalysts to synthesize highly enantiomerically pure compounds has been one of the most impressive achievements in the asymmetric hydrogenation of prochiral ole®nic substrates. Homogeneous asymmetric hydrogenation of enamides catalyzed by transition metal complexes has become one of the most powerful methods for synthesizing optically active organic compounds. High enantioselectivities have been obtained in the synthesis of amino acids and related compounds through Rh complex±catalyzed asymmetric hydrogenation. Representative asymmetric catalytic hydrogenation reactions are shown in the table of Scheme 6±215 in
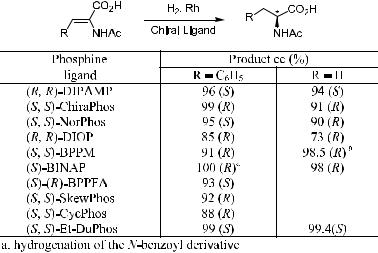
6.1 INTRODUCTION 335
Scheme 6±2. Catalytic hydrogenation of enamindes.
which the results for various Rh complex±catalyzed enantioselective hydrogenations of a-acylaminoacrylic acids to the corresponding amino acids are summarized.
The reaction mechanism in phosphine±Rh complex catalyzed hydrogenation was elucidated by Halpern16 and Brown and Maddox17 on the basis of NMR and X-ray crystallographic studies of the reaction intermediates, as well as detailed kinetic analyses. The well-recognized mechanism proposed by Halpern is presented in Figure 6±3.
First, solvent molecules, referred to as S in the catalyst precursor, are displaced by the ole®nic substrate to form a chelated Rh complex in which the ole®nic bond and the amide carbonyl oxygen interact with the Rh(I) center (rate constant k1). Hydrogen then oxidatively adds to the metal, forming the Rh(III) dihydride intermediate (rate constant k2). This is the rate-limiting step under normal conditions. One hydride on the metal is then transferred to the coordinated ole®nic bond to form a ®ve-membered chelated alkyl±Rh(III) intermediate (rate constant k3). Finally, reductive elimination of the product from the complex (rate constant k4) completes the catalytic cycle.
When an appropriate chiral phosphine ligand and proper reaction conditions are chosen, high enantioselectivity is achievable. If a diphosphine ligand with C2 symmetry is used, two diastereomers for the enamide-coordinated complex can be formed because the ole®n can interact with the metal from either the Reor Si-face. Therefore, enantioselectivity is determined by the relative concentrations and reactivities of the diastereomeric substrate±Rh complexes. It should be mentioned that in most cases it is not the preferred mode of initial binding of the prochiral ole®nic substrate to the catalyst that dictates the ®nal stereoselectivity of these catalyst systems. The determining factor is the di¨er-
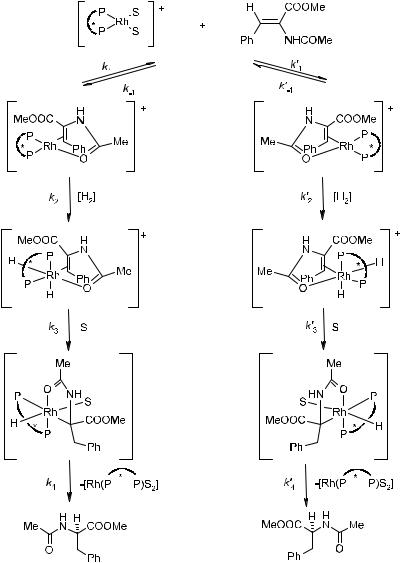
336 ASYMMETRIC CATALYTIC HYDROGENATION AND OTHER REDUCTION REACTIONS
Figure 6±3. Mechanism for the hydrogenation of a prochiral substrate methyl acet-
amidocinnamate (MAC) with a catalyst containing a chiral chelating diphosphine ligand
_
(P * P). S ˆ Methanol.
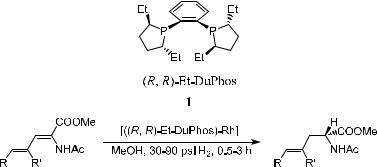
6.1 INTRODUCTION 337
Scheme 6±3
ence in the rate of the subsequent reactions of the diastereomeric catalyst± substrate adducts with hydrogen. Interestingly it is the minor diastereomer rather than the major one that determines the predominate chirality of the product because the minor diastereomer is much more reactive than the major one. The following are some examples of using catalytic asymmetric hydrogenation to synthesize optically active compounds.
Unsaturated amino acids are an important class of natural products that have shown interesting biological properties.18 Among them, g,d-unsaturated amino acids have been isolated from a variety of natural sources19 and served as intermediates in the synthesis of complex amino acids and peptides.20 This type of compound can be synthesized via the catalytic hydrogenation of the corresponding substrate. As shown in Scheme 6±3, the asymmetric hydrogenation of a,g-dienamide ester catalyzed by [Rh-…R;R†-Et-DuPhos]‡ a¨ords the corresponding g,d-unsaturated amino acid with excellent enantioselectivity.21
In this reaction, the Et-DuPhos-Rh catalyst is applied at a substrate-to- catalyst ratio S/C ˆ 500 under an initial hydrogen pressure ranging from 30 to 90 psi. Full conversion of the substrate can be obtained in 0.5 to 3 hours. In all cases, less than 2% over-reduction was detected, and the products were isolated with better than 95% yield. The amount of over-reduction product varied but could be minimized by careful control of the S/C ratio, reaction time, and, to a lesser degree, initial hydrogen pressure. In all cases, the hydrogenation of the enamide double bond preceded the reduction of the distal double bond. When …R;R†-Et-DuPhos was used as the chiral ligand, the product in (R)-con®guration was obtained, while product in (S)-con®guration was obtained when …S;S†- DuPhos was used.
a-Aminophosphinic acids, structural analogs of a-aminocarboxylic acids, have interesting biological properties and serve as active herbicides, bactericides, and antibiotics. The optically active a-aminophosphinic acids and their derivatives can also be synthesized via catalytic asymmetric hydrogenation of the corresponding phosphinic substrates. As shown in Scheme 6±4, optically active a-aminophosphinic acids are synthesized via asymmetric hydrogenation of the
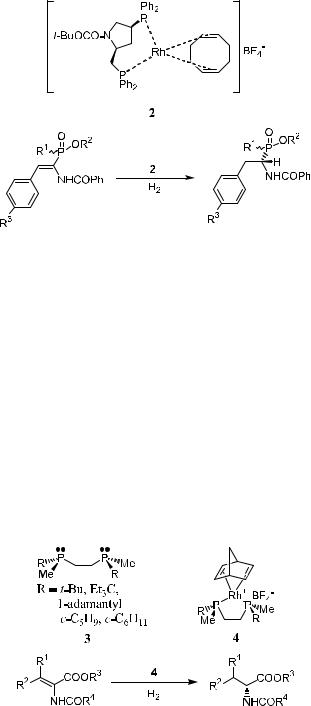
338 ASYMMETRIC CATALYTIC HYDROGENATION AND OTHER REDUCTION REACTIONS
Scheme 6±4
corresponding unsaturated precursor catalyzed by Rh(I) complex 2. Enantiomeric excess of 98% can be obtained when …S;S†-BPPM is used as the chiral ligand.22
Optically active phosphines can play an important role as chiral ligands in various metal-catalyzed asymmetric reactions, and numerous chiral phosphines have been designed and synthesized. Some P-chiral phosphines were landmark discoveries at an early stage in the history of asymmetric hydrogenation reactions. However, less attention has been paid to P-chiral phosphine ligands in asymmetric catalysis for two reasons. First, there is synthetic di½culty in getting highly enantiomerically enriched P-chiral phosphines; second, this class of chiral phosphines, especially the diarylphosphines and triarylphosphines, are con®gurationally unstable and gradually racemize at high temperatures.
Optically active trialkylphosphines are known to be con®gurationally stable toward racemization, and ligands of type 3 have been synthesized and applied in the asymmetric catalytic hydrogenation of amidoacrylic acids (Scheme 6±5).
Scheme 6±5

6.1 INTRODUCTION 339
This type of ligand forms a ®ve-membered chelate with transition metals, and this feature may lead to high enantioselectivity in asymmetric synthesis. Furthermore, as trialkylphosphines are electron-rich ligands, reactions catalyzed by complexes containing these chiral P-coordinated ligands are anticipated to show high e½ciency. A variety of substrates have been hydrogenated in the presence of chiral catalyst 4 (Scheme 6±5). Higher than 99.9% ee has been obtained in some speci®c reactions.23
6.1.2.2 Asymmetric Catalytic Hydrogenation of Acrylic Acids and Derivatives. Enantiomerically pure 2-substituted succinic acid derivatives have attracted great interest because of their utility as chiral building blocks for peptidomimetics in the design of pharmaceuticals, ¯avors, fragrances, and agrochemicals. Asymmetric catalytic hydrogenation of b-substituted itaconic acid derivatives o¨ers one of the most practical and convenient routes to these compounds.
Burk et al. reported an asymmetric hydrogenation catalyzed by [(EtDuPhos)Rh]‡ catalyst. Very high enantioselectivity was obtained. When R ˆ i-Pr, the minor enantiomer could not be detected by chiral GC methods. The results are shown in Scheme 6±6.24
Scheme 6±6. Reprinted with permission by Wiley-VCH Verlag GmbH, Ref. 24.
Generally, stereoselectivity can be enhanced by increasing the interactions between functional groups in a substrate and the chiral ligands. In light of this concept, Hayashi et al.25 designed chiral aminoalkylferrocenyl phosphine ligands in which an amino group was introduced. This type of ligand o¨ers high
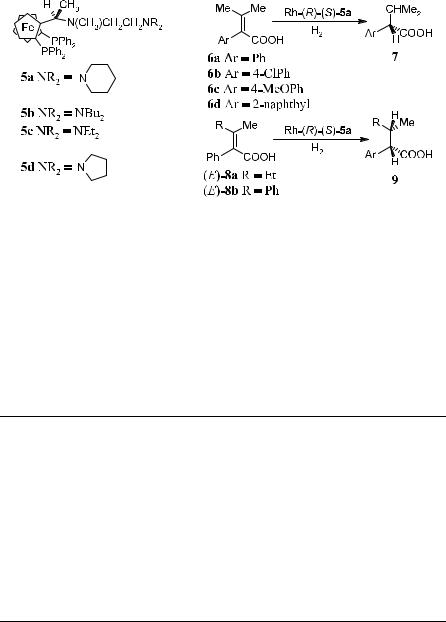
340 ASYMMETRIC CATALYTIC HYDROGENATION AND OTHER REDUCTION REACTIONS
stereoselectivity as well as reasonable catalytic activity in the hydrogenation of trisubstituted acrylic acids (tetrasubstituted ole®ns) (Scheme 6±7).
Scheme 6±7
Rh complexes with ChiraPhos, PyrPhos, or ferrocenyl phosphines lacking amino alkyl side chains (such as BPPFA) are much less active toward tetrasubstituted ole®ns. Table 6±1 shows that in asymmetric hydrogenations catalyzed by 5a±d, the coordinated Rh complex exerts high selectivity on various substrates. It is postulated that the terminal amino group in the ligand forms an ammonium carboxylate with the ole®nic substrates and attracts the substrate to the coordination site of the catalyst to facilitate the hydrogenation.
TABLE 6±1. Asymmetric Hydrogenation of Trisubstituted Acrylic Acids Catalyzed by Chiral Ferrocenylphosphine±Rhodium Complexes
Entry |
Ole®n |
Ligand |
Solvent |
Time (h) |
Product |
ee (%, con®g.) |
|
|
|
|
|
|
|
1 |
6a |
5a |
THF/MeOH (90/10) |
30 |
7a |
98.4(S) |
2 |
6a |
5a |
THF/MeOH (80/20) |
20 |
7a |
97.6(S) |
3 |
6a |
5a |
i-PrOH |
20 |
7a |
97.0(S) |
4 |
6a |
5a |
MeOH |
5 |
7a |
95.8(S) |
5 |
6a |
5b |
THF/MeOH (80:20) |
20 |
7a |
97.9(S) |
6 |
6a |
5c |
THF/MeOH (80:20) |
30 |
7a |
98.1(S) |
7 |
6a |
5d |
THF/MeOH (80:20) |
30 |
7a |
98.2(S) |
8 |
6b |
5a |
THF/MeOH (80:20) |
40 |
7b |
97.4(S) |
9 |
6c |
5a |
THF/MeOH (80:20) |
40 |
7c |
96.7(S) |
10 |
6d |
5a |
THF/MeOH (80:20) |
65 |
7d |
97.3(S) |
11 |
(E )-8a |
5a |
i-PrOH |
100 |
9a |
97.3…2S;3S† |
12 |
(E )-8b |
5a |
THF/MeOH (80:20) |
100 |
9b |
92.1…2S;3R† |
ee ˆ Enantiomeric excess.
Reprinted with permission by Am. Chem. Soc., Ref. 25.

6.1 INTRODUCTION 341
Kang et al.6 reported a practical synthesis of an air-stable ferrocenyl bis- (phosphine) …pS;pS†-1,10-bis-(diphenylphosphino)-2,20-di-3-pentyl ferrocene (‰S;SŠ-FerroPhos, 10a) and its application in the rhodium(I)-catalyzed enantioselective hydrogenation of dehydroamino acid derivatives.
In the presence of a catalyst prepared in situ from [Rh(COD)2]BF4 and 10a, the hydrogenation of various dehydroamino acid derivatives proceeds smoothly under mild conditions (2 atm, 20 ±30 C) with high enantioselectivity (over 99.9% ee can be obtained). The enantioselectivity in the hydrogenation of a- acetamidocinnamic acid compares favorably with the reported ee from the reactions catalyzed by other air-stable triarylsubstituted ligands such as BINAP (84%)26 and 2,2-PhanePhos (98%).27 Due to the high enantioselectivity of this new ligand and its high stability toward air, it may have good potential for industrial application.
Several ferrocenyl phosphines have found industrial applications in the synthesis of chiral pharmaceuticals and agrochemicals. A process developed by Ciba-Geigy (now Novartis) for the production of a herbicide (S)-Metolachlor involves the asymmetric hydrogenation of an imine using an Ir complex of 10b as catalyst.28a Similar technologies involving an Rh catalyst±containing ligand 10c and an Ir catalyst±containing ligand 10d were developed by Lonza Fine Chemicals in partnership with Ciba-Geigy for the production of the vitamin (‡)-biotin and the cough medicine dextromethorphan, respectively.28b,c Firmenich developed a process for the production of (‡)-cis-Hedione8, a perfume ingredient, by using an Ru complex of 10e.28d The key steps of these processes are illustrated in Scheme 6±8.
Many attempts have been made to develop novel nonracemic ferrocenyl phosphine derivatives as asymmetric hydrogenation catalysts. Interested readers will ®nd the design and synthesis of these chiral ferrocenyl phosphine ligands in a recent review by Richards and Locke.28e