
j.electacta.2013.03.143
.pdf
Figure 9. Comparison between the experimental and calculated steady-state data for (a) pH=8.15
(b) pH=10.
4.3. Determination of Iron Interstitials Diffusion Coefficient
field (ε) (i.e., migration). The diffusivity of iron interstitials through the passive layers was calculated according to Equation (47) [22] as a function of potential and is plotted in Figure 10.
Based upon the PDM, the metal interstitialsManuscriptgenerated at the m/bl interface move toward the bl/s interface and the driving force for this transport phenomenon is primarily the electric
Figure 10. Calculated diffusivity of iron interstitials as a function of potential, T = 22 oC
The diffusivity of iron interstitials (the presumed dominant point defect) is calculated to be in the range of 10-14 to 10-16 cm2/s and to be essentially independent of the applied potential, as predicted by PDM. The calculated diffusion coefficients for iron interstitials are in close agreement withAcceptedthose reported in the literature [44,57,58]. However, a small dependence on pH is found (Figure 10), where none should probably exist. The origin of this dependence is currently unknown, but it probably arises because of uncertainties in the values of fundamental parameters determined by the optimization. We note that the interstitial diffusivity is a “deeply buried” parameter, whose value is sensitive to the optimization at very low frequencies (down to 10-2 Hz), where the measured impedance data are the least accurate, but at which the impedance is not particularly sensitive to the value of Di. We will address this problem in future work by measuring the impedance down to a lower frequency of 10-3 Hz, where defect transport plays a more significant role in determining the impedance of the system than it does at the higher, minimum frequency of 10-2 Hz employed in the present work.
5. Summary and Conclusions
30
Page 30 of 57

The findings of this work on the nature of the passive state on iron can be summarized as follows:
Optimization of the impedance model derived from the PDM on the experimental EIS
data has resulted in determination of sets Manuscriptof parameters that can be used to predict the accumulation of general corrosion damage on iron in pH-neutral solutions.
Extension of the PDM to include a parallel electronic resistance and a Randles circuit representing a redox impedance for the redox reaction that accepts the electronic charge generated within the barrier layer by ionization of the defects, shows that, provided any redox species are at sufficiently low concentrations, the parallel impedance is sufficiently high (order of 3 × 109 Ω.cm2) that values for the PDM parameters can still be extracted by optimization of the impedance model on experimental EIS data. The parallel
electronic impedance was calculated theoretically using the defect concentrations in the barrierAcceptedlayer, which were derived from the parameter values (rate constants, transfer coefficients, etc.) obtained from optimization of the PDM on the experimental EIS data.
The thickness of the barrier oxide layer of the passive films that form anodically on iron within the passive range, as measured experimentally using spectroscopic ellipsometry is found to be in reasonable agreement with that calculated employing the PDM using values for various model parameters determined by optimizing the PDM on experimental EIS data. The passive current density for iron within the passive range, as measured experimentally, is found to be in good agreement with that calculated from the PDM using values for various model parameters determined by optimization. The thickness of the barrier layer was observed to increase linearly with applied voltage and the passive
31
Page 31 of 57

current density was found to be independent of voltage, as predicted by the PDM, for the
case where no change in cation oxidation state occurs upon barrier layer dissolution or
This represents powerful confirmation of the validity of the PDM, at
least in the case of iron.
6. Acknowledgments
The authors gratefully acknowledge the support of this work by the ONDRAF/NIRAS of
Belgium. |
Manuscript |
|
[1] M. Nagayama, M. Cohen, The Anodic Oxidation of Iron in a Neutral Solution: I . The Nature and Composition of the Passive Film, J. Electrochem. Soc. 109 (1962) 781.
[2] N. Sato, M. Cohen, The Kinetics of Anodic Oxidation of Iron in Neutral Solution: I . Steady
Growth Region, J. Electrochem. Soc. 111 (1964) 512.
[3] N. Sato, T.AcceptedNoda, K. Kudo, Thickness and structure of passive films on iron in acidic and basic solution, Electrochim. Acta 19 (1974) 471.
[4] P. Schmuki, S. Virtanen, A. Davenport, C. Vitus, In Situ X Ray Absorption Near Edge
Spectroscopic Study of the Cathodic Reduction of Artificial Iron Oxide Passive Films, J. Electrochem. Soc. 143 (1996) 574.
[5]E. Sikora, D. D. Macdonald, The Passivity of Iron in the Presence of Ethylenediaminetetraacetic Acid I. General Electrochemical Behavior, J. Electrochem. Soc. 147 (2000) 4087.
[6]J. Liu and D. D. Macdonald, The Passivity of Iron in the Presence of Ethylenediaminetetraacetic Acid. II. The Defect and Electronic Structures of the Barrier Layer, J. Electrochem. Soc. 148 (2001) B425.
32
Page 32 of 57

[7] M. Büchler, P. Schmuki and H. Böhni, Iron Passivity in Borate Buffer: Formation of a Deposit Layer and Its Influence on the Semiconducting Properties, J. Electrochem. Soc. 145 (1998) 609.
[8] T. Ohtsuka, H. Yamada, Effect of ferrous ion in solution on the formation of anodic oxide
film on iron, Corrosion Sci. 40 (1998) 1131. |
Manuscript |
|
[9] Alison J. Davenport, Lucy J. Oblonsky, Mary P. Ryan and Michael F. Toney, The Structure of the Passive Film That Forms on Iron in Aqueous Environments, J. Electrochemical Soc. 147 (2000) 2162.
[10] Michael F. Toney, Alison J. Davenport, Lucy J. Oblonsky, Mary P. Ryan, and Carissima M. Vitus, Atomic Structure of the Passive Oxide Film Formed on Iron, Phys. Rev. Lett 79(21) (1997) 4282.
[11] M Büchler, P Schmuki, H Böhni, T Stenberg, T Mäntylä, Comparison of the
Semiconductive Properties of Sputter Deposited Iron Oxides with the Passive Film on Iron, J.
[12]L. Young, Anodic Oxide Films, Academic Press, New York, 1961.
[13]D. D. Macdonald, Passivity the key to our metals-based civilization, Pure Appl. Chem. 71 (1999) 951.
[14]D.D. Macdonald, S.R. Biaggio, and H. Song, Steady State Passive Films: Interfacial Kinetic
Effects and Diagnostic Criteria, J. Electrochem. Soc. 139 (1992) 170.
[15]D. D. Macdonald and M. Urquidi-Macdonald, Theory of Steady State Passive Films, J.Accepted
Electrochem. Soc. 137 (1990) 2395.
[16] D. D. Macdonald, The Point Defect Model for the Passive State, J. Electrochem. Soc. 139 (1992) 3434.
33
Page 33 of 57

[17] D. D. Macdonald and G. R. Engelhardt, The Point Defect Model for Bi-Layer Passive Films, ECS Trans 28(24) (2010) 123.
[18] R. Storn and K. Price, Differential Evolution – A Simple and Efficient Heuristic for global Optimization over Continuous Spaces, Journal of Global Optimization 11 (1997) 341.
[19] Z. Lu, D. D. Macdonald, Transient growth andManuscriptthinning of the barrier oxide layer on iron measured by real-time spectroscopic ellipsometry, Electrochim. Acta 53 (2008) 7696.
[20] C. Y. Chao, L. F. Lin, and D. D. Macdonald, A Point Defect Model for Anodic Passive Films: I . Film Growth Kinetics, J. Electrochem. Soc. 128 (1981) 1187.
[21] L.F. Lin, C-Y. Cao, and D. D. Macdonald, A Point Defect Model for Anodic Passive Films: II . Chemical Breakdown and Pit Initiation, J. Electrochem. Soc. 128 (1981) 1194.
[22] C.Y. Chao, L.F. Lin, and D. D. Macdonald, A Point Defect Model for Anodic Passive Films: III . Impedance Response, J. Electrochem. Soc. 129 (1982) 1874.
[23] D. D. Macdonald, On the Existence of Our Metals-Based Civilization: I. Phase-Space Analysis, J. Electrochem. Soc. 153 (2006) B213.
[24] H. Song and D.D. Macdonald, Photoelectrochemical Impedance Spectroscopy: I .
Validation of the Transfer Function by Kramers Kronig Transformation, J. Electrochem. Soc.
138 (1991) 1408.
[25]D. D. Macdonald, E. Sikora, M. W. Balmas, and R. C. Alkire, The photo-inhibition of localized corrosion on stainless steel in neutral chloride solution, Corrosion Sci. 38 (1996) 97.
[26]M. Urquidi-Macdonald and D.D. Macdonald, Solute Vacancy Interaction Model and theAccepted
Effect of Minor Alloying Elements on the Initiation of Pitting Corrosion, J. Electrochem. Soc. 132 (1985) 555.
[27]L. Zhang and D. D. Macdonald, Segregation of alloying elements in passive systems—I. XPS studies on the Ni–W system, Electrochim. Acta 43 (1998) 2661.
[28]D. D. Macdonald and S. I. Smedley, An electrochemical impedance analysis of passive films on nickel(111) in phosphate buffer solutions, Electrochim. Acta, 35 (1990)1949.
34
Page 34 of 57

[29] D. D. Macdonald and M. Urquidi-Macdonald, Application of Kramers Kronig Transforms
in the Analysis of Electrochemical Systems: I . Polarization Resistance, J. Electrochem. Soc. 132 |
|
(1985) 2316. |
Manuscript |
|
[30] M. Urquidi-Macdonald, S Real and D. D. Macdonald, Application of Kramers Kronig
Transforms in the Analysis of Electrochemical Impedance Data: II . Transformations in the Complex Plane, J. Electrochem. Soc. 133 (1986) 2018.
[31] J. Ai, Y-Z. Chen, M. Urquidi-Macdonald, and D.D. Macdonald, Electrochemical Impedance Spectroscopic Study of Passive Zirconium: I. High-Temperature, Deaerated Aqueous Solutions ,
J. Electrochem. Soc. 154 (2007) C43.
[32] J. Ai, Y-Z. Chen, M. Urquidi-Macdonald, and D.D. Macdonald, Electrochemical Impedance Spectroscopic Study of Passive Zirconium: II. High-Temperature, Hydrogenated Aqueous
Solutions, J. Electrochem.AcceptedSoc. 154 (2007) C52.
[33] D. D. Macdonald and A. Sun, An electrochemical impedance spectroscopic study of the passive state on Alloy-22, Electrochim. Acta, 51 (2006) 1767.
[34] J. Geringer, M.L. Taylor, D.D Macdonald, Predicting the steady state thickness of passive films with the Point Defect model in fretting corrosion experiments, Electrochemical and SolidState Science, in progress.
[35]DataFit, Oakdale Engineering, www.oakdaleengr.com
[36]K. Levenberg, A Method for the Solution of Certain Problems in Least Squares, Quart. Appl. Math. 2 (1944) 164.
[37]“Ellis 2: Complex curve fitting for one independent variable | IgorExchange.” [Online]. Available: http://www.igorexchange.com/project/Ellis2. [Accessed: 07-Aug-2012].
[38]T. Bäck and H.-P. Schwefel, An Overview of Evolutionary Algorithms for Parameter Optimization,
Evolutionary Computation 1(1993) 1.
[39]O. Rosas-Camacho, M. Urquidi-Macdonald, and D. D. Macdonald, Deterministic Modeling of the Corrosion of Low-Carbon Steel by Dissolved Carbon Dioxide and the Effect of Acetic Acid. I-Effect of Carbon Dioxide, ECS Transactions 19(29) (2009) 143.
35
Page 35 of 57

[40] J. Ai, Y. Chen, M. Urquidi-Macdonald and D. D. Macdonald, Electrochemical impedance spectroscopic study of passive zirconium, Journal of Nuclear Materials 379 (2008) 162.
[41] D. D. Macdonald , J. Ai, Y. Chen, and M. Urquidi-Macdonald , Electrochemical Impedance Spectroscopic Study of Passive Zirconium in High Temperature De-aerated Aqueous Solutions,
ECS Transactions 2(17)(2007) 29. |
Manuscript |
|
[42] M. Bojinov, G. Fabricius, T. Laitinen, K. Makela, T.Saario and G. Sundholm, Coupling between ionic defect structure and electronic conduction in passive films on iron, chromium and iron–chromium alloys, Electrochim Acta 45 (2000) 2029.
[43] M. Bojinov, G. Fabricius, P. Kinnunen, T. Laitinen, K. Makela, T.Saario and G. Sundholm, Electrochemical study of the passive behaviour of Ni–Cr alloys in a borate solution—a mixedconduction model approach, J. Electroanal.Chem. 504 (2001) 29.
[44] M. Bojinov, G. T. Laitinen, K. Makela and T.Saario. and G. Sundholm, Conduction Mechanism of the Passive Film on Iron Based on Contact Electric Impedance and Resistance Measurements, J. Electrochem. Soc. 148 (2001) B243.
currents in large electric fields for discrete
[47] R. D. L. Kronig,AcceptedOn the theory of dispersion of x-rays, J. Opt. Soc. Am. 12 (1926) 547.
[48] H. A. Kramers, Zur Struktur der Multiplett-S-Zustände in zweiatomigen Molekülen. I, Z. Phys. 30 (1929) 521.
[49] D. D. Macdonald , M. Urquidi-Macdonald, Kramers Kronig Transformation of Constant
Phase Impedances, J. Electrochem. Soc. 137 (1990) 515.
[50]M. Urquidi-Macdonald, S. Real, and D. D. Macdonald, Applications of Kramers—Kronig transforms in the analysis of electrochemical impedance data—III. Stability and linearity,
Electrochim. Acta 35 (1990) 1559.
[51]S.N. Sivanandam and S.N. Deepa, Introduction to Genetic Algorithms, Springer Verlag, (2007).
[52]M. Gulsen, A. Smith and D. Tate, A genetic algorithm approach to curve fitting, Int. J Prod. Res. 33 (1995) 1911.
36
Page 36 of 57

[53] T.VanderNoot and I. Abrahams, The use of genetic algorithms in the non-linear regression of immittance data, Journal of Electroanalytical Chemistry 448(1) (1998) 17.
[54] “GenCurvefit | IgorExchange.” [Online]. Available: http://www.igorexchange.com/project/gencurvefit. [Accessed: 07-Aug-2012].
[55] B. M. Marx, Exploring the Mechanisms of ManuscriptPassivity on Iron: Experimental Methods for
Characterizing and Developing Models to Describe Nano-Oxide Growth, The Pennsylvania State University, University Park, USA (2006).
[56] M. Büchler, P. Schmuki and H. Böhni, Formation and Dissolution of the Passive Film on Iron Studied by a Light Reflectance Technique, J. Electrochem. Soc. 144 (1997) 2307.
[57] Y.F. Cheng, C. Yang, J.L. Luo, Determination of the diffusivity of point defects in passive films on carbon steel, Thin Solid films 416 (2002) 169.
[58] X. Zhang, J.C. Wren, I. Betova, M. Bojinov, Estimation of kinetic parameters of the passive state of carbon steel in mildly alkaline solutions from electrochemical impedance spectroscopic and X-ray photoelectron spectroscopic data, Electrochim. Acta 56 (2011) 5910.
Accepted
Tables
37
Page 37 of 57
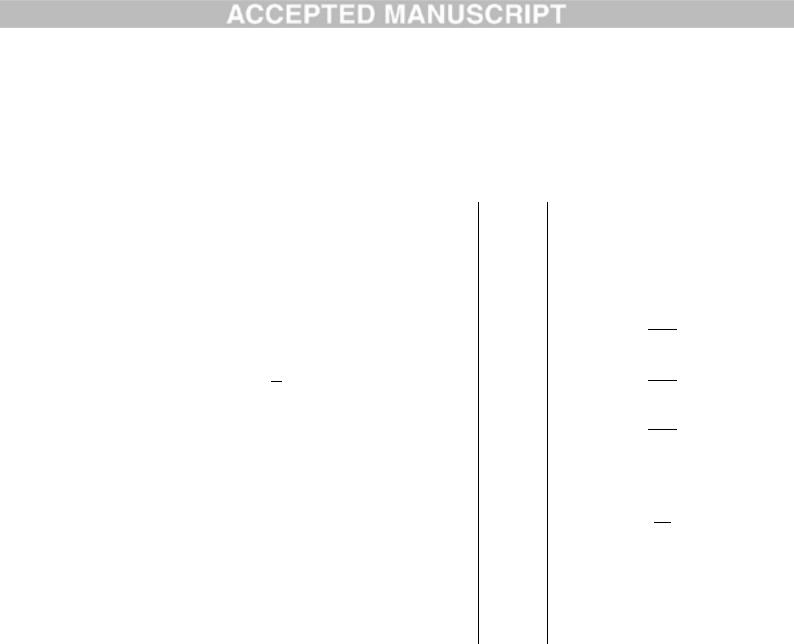
Table 1: Coefficients for the rate constants for the reactions that generate and annihilate point defects at the m/bl interface [Reactions (1) – (3)] and at the bl/s interface [Reactions (4) – (6)],
Figure 1, and for dissolution of the film [19-21]. |
ki ki0eaiV ebi Leci pH |
|
|
|
|
|
||||||||||||||||||
|
|
|
|
|
Reaction |
|
|
|
|
|
|
|
ai (V 1) |
bi (cm 1) |
ci |
Units of |
||||||||
|
|
|
|
|
|
|
|
|
|
|
|
ki0 |
|
|||||||||||
|
|
|
|
|
|
|
|
|
|
|
|
|
|
|
|
|
|
|
|
|
|
|
||
|
|
|
|
|
|
|
|
|
|
|
|
|
|
|
|
|
|
|
|
|
||||
|
|
|
|
|
k7 |
|
|
|
|
|
|
|
|
|
|
Manuscript |
mol |
|
||||||
|
(1) |
m VM |
' |
k |
M M |
vm e ' |
|
1 |
(1 ) |
1 |
K |
- 1β |
1 |
|
||||||||||
|
|
1 |
|
|
|
s |
|
|
||||||||||||||||
|
|
|
|
|
|
|
|
|
|
|
|
|
|
|
|
|
|
|
|
|
|
|
|
|
|
|
|
|
|
|
|
|
|
|
|
|
|
|
|
|
|
|
|
|
|
||||
|
|
(2) |
|
|
|
k2 |
|
|
vm e' |
|
|
2 |
(1 ) |
2 |
K |
- 2β |
mol |
|||||||
|
|
|
m Mi |
|
|
|
|
|
cm |
2 |
s |
|||||||||||||
|
|
|
|
|
|
|
|
|
|
|
|
|
|
|
|
|
|
|
|
|
|
|
||
|
|
|
|
|
|
|
|
|
|
|
|
|
|
|
|
|
|
|
|
|||||
|
|
(3) |
|
|
k3 |
|
|
|
|
|
.. |
|
|
3 |
(1 ) |
3 |
K |
- 3β |
mol |
|||||
|
|
|
m MM |
|
2 |
VO e' |
|
|
|
cm2 s |
||||||||||||||
|
|
|
|
|
|
|
|
|
|
|
|
|
|
|
|
|
|
|
|
|
||||
|
|
|
|
|
k |
|
|
|
|
|
|
|
|
|
|
|
|
|
|
mol |
||||
|
(4) |
M M 4 M |
|
|
( )e' |
|
4 |
|
|
4βδ |
cm2 s |
|||||||||||||
|
|
|
|
|
|
|
|
|
|
|
|
|
|
|
|
|
|
|
|
|
||||
|
(5) |
|
|
|
k |
|
|
|
|
( )e' |
|
5 |
|
|
5βδ |
cm |
||||||||
|
M i |
|
5 M |
|
|
|
|
|
s |
|
||||||||||||||
|
|
|
|
|
|
|
|
|
|
|
|
|
|
|
|
|
|
|
|
|
|
|
||
|
|
|
|
|
|
|
|
|
|
|
|
|
|
|
|
|
|
|
|
|
|
|
||
|
(6) |
VO H |
2O OO |
2H |
|
|
|
2 6 |
|
|
6βδ |
cm |
||||||||||||
|
|
|
|
.. |
|
|
|
|
k6 |
|
|
|
|
|
|
|
|
|
|
|
|
|
||
|
|
|
|
|
|
|
|
|
|
|
|
|
|
|
|
|
|
|
|
|
|
s |
|
|
|
|
|
|
|
|
|
|
|
|
|
|
|
|
|
|
|
|
|
|
|
|
|
|
|
|
|
|
|
|
|
|
(7) |
|
|
|
|
|
|
|
|
|
|
|
|
|
|
|
|
|
|
|
|
Accepted |
|
7 ( ) |
|
|
|
|
|
|
|||||||||||||
MO |
H M |
|
H2O ( ) |
|
|
|
7(δ- )β |
|
|
|
||||||||||||||
|
2 |
|
|
|
|
|
|
|
|
|
|
|
2 |
|
|
|
|
|
|
|
|
cm s |
38
Page 38 of 57

Table 2: Parameter values used to calculate the electronic impedance in parallel with the barrier layer.
Parameter |
|
Value/units |
Source |
|
|
|
|
|
|
De |
|
10-11/ cm2 s-1 |
[44] |
|
|
|
|
|
|
ˆ |
|
30 |
[18] |
Manuscript |
|
|
|||
|
|
|
|
|
C |
|
25×10-6 / F cm-2 |
Estimated as a typical value |
|
|
|
|
|
|
H2O |
|
0.5 |
Estimated by using OLI software [46] |
|
B |
|
3.92×10-11 / A cm-2 |
Estimated by using OLI software [46] |
|
|
|
|
|
|
Di |
|
1.4×10-14 / cm2 s-1 |
Preliminary optimization |
|
|
|
|
|
|
Do |
|
1.4×10-14 / cm2 s-1 |
Preliminary optimization |
|
|
|
|
|
|
|
Accepted |
|
||
|
|
|
|
39 |
Page 39 of 57