
j.electacta.2013.03.143
.pdf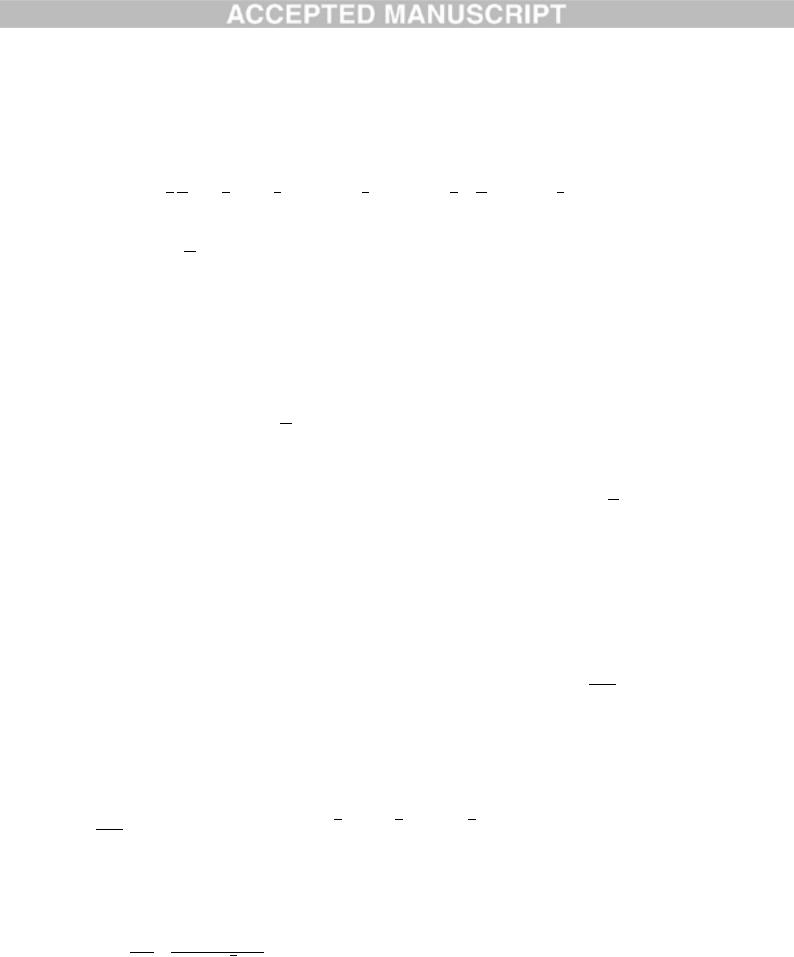
where
IU F a1k1CvL k2 a2 k3a3 ( )k4 a4 ( )k5 a5Ci0 ( )k7 a7 (17)
IL |
F b1k1CeL k2b2 k3b3 |
(18) |
|
I L F k |
|
(19) |
|
v |
1 |
|
|
|
|
|
|
I 0 |
F ( )k |
5 |
(20) |
i |
|
||
|
|
|
|
Here, it is assumed that U U U U Ue j t |
and we identify the four terms on the right |
side as arising from relaxations with respect to theManuscriptapplied potential V, the thickness of the
barrier layer with respect to the voltage at the bl/ol interface, U, cation vacancies, C L , and cation
interstitials, Ci0 , respectively. Note the absence of a term for the relaxation of oxygen vacancies,
Accepted |
|
|
||
because, again, the concentration of oxygen vacancies does not appear in the current [Equation |
||||
(1)], as a consequence of assuming Reaction (3), Figure 1, to be irreversible. |
|
|
||
Let us now calculate L |
, CvL |
and Ci0 . It is convenient to start with |
L |
. The rate of |
U |
U |
U |
U |
|
change of the thickness of the barrier layer is described by Equation (9). Accordingly, by taking the total differential, we have
d L j Le j t k |
3 |
k |
7 |
(k a U k b L) k a V |
(21) |
|||||||||
dt |
|
|
|
|
|
3 |
3 |
3 |
3 |
7 |
7 |
|
||
|
|
|
|
|
|
|
|
|
|
|
|
|
|
|
or |
|
|
|
|
|
|
|
|
|
|
|
|
|
|
LU |
L |
|
(k3a3 k7a7 ) |
|
|
|
|
|
|
|
(22) |
|||
U |
j k |
b |
|
|
|
|
|
|
|
|
||||
|
|
|
3 |
|
3 |
|
|
|
|
|
|
|
|
|
|
|
|
|
|
|
|
|
|
|
10 |
|
|
|
|
Page 10 of 57
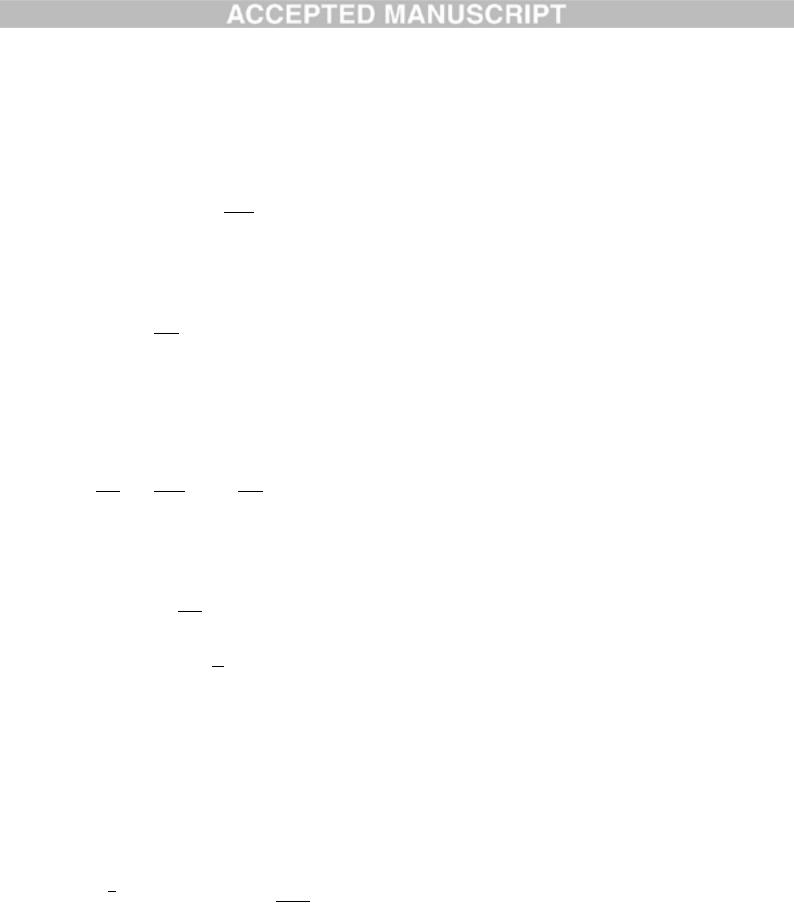
which is the result that we desire.
2.2. Calculation of |
Ci0 |
U |
|
The flux density of interstitials is |
Manuscript |
|
|
|
||
J |
D Ci D KC |
|
(23) |
i |
i x i |
i |
|
Here, Di is the diffusion coefficient of the cation interstitials, K = Fε/RT, where ε is the electric field strength inside the barrier layer, and T is the temperature. The continuity equation is
C |
i |
|
D |
2C |
i |
D K |
C |
i |
|
|
|
|
|
(24) |
||||||
t |
|
|
|
x |
|
|
|
|
|
|||||||||||
|
|
|
i x |
2 |
|
|
|
i |
|
|
|
|
|
|
|
|
||||
with the boundary conditions |
|
|
|
|
|
|||||||||||||||
k C |
|
|
D |
|
Accepted |
|
|
|||||||||||||
|
|
Ci D KC at |
x |
0 |
|
(25) |
||||||||||||||
5 i |
|
|
|
i |
|
x |
|
i |
|
|
i |
|
|
|
|
|
||||
Substitution |
|
C |
i |
C |
C |
e j t |
into |
Equations (23) |
to (25) |
and linearization of boundary |
||||||||||
|
|
|
|
|
|
|
|
i |
|
|
|
i |
|
|
|
|
|
|
||
conditions relative to ΔU and ΔL yields: |
|
|
||||||||||||||||||
j C |
|
|
|
2 C |
i |
D K |
C |
i |
|
|
|
(26) |
||||||||
|
|
x2 |
|
|
|
x |
|
|
|
|||||||||||
|
|
i |
|
|
|
|
|
i |
|
|
|
|
|
|
|
|
||||
or |
|
|
|
|
|
|
|
|
|
|
|
|
|
|
|
|
|
|
|
|
k5 (Ci |
|
|
|
|
|
|
|
|
|
|
|
C |
Di K Ci0 at |
x 0 |
|
|||||
0a5 U Ci0 ) Di |
|
i |
(27) |
|||||||||||||||||
|
|
|
|
|
|
|
|
|
|
|
|
|
|
|
|
|
x x 0 |
|
|
11
Page 11 of 57
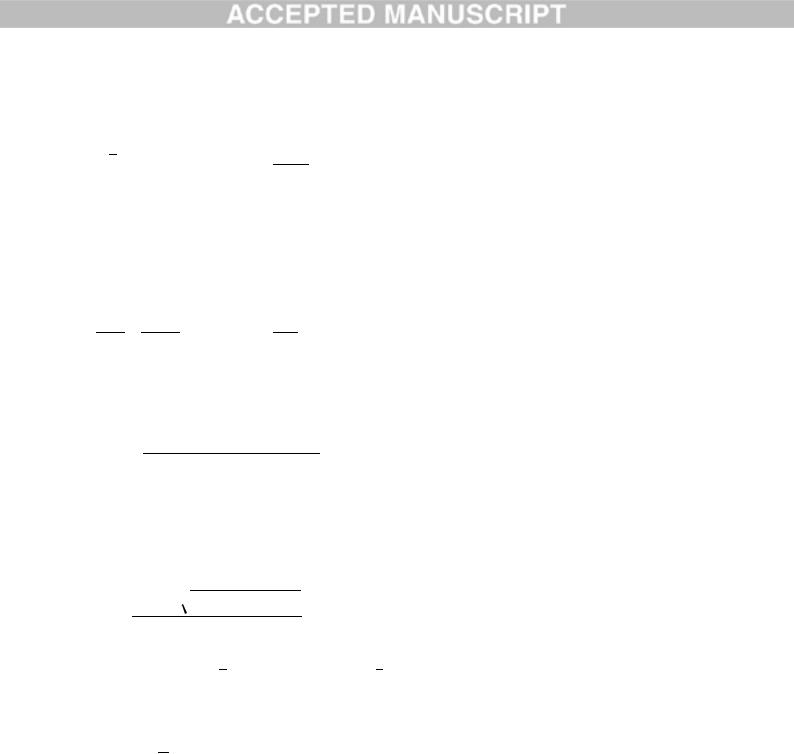
k |
|
( a |
U b |
C |
|
D |
K C L at x |
L |
(28) |
|
|
L ) D |
|
i |
|||||||
|
2 |
2 |
2 |
i |
x |
x L |
i |
i |
|
|
Analytical solution of the linear boundary problem (26) – (28) can be easily obtained and the
sought value C 0 |
/ U can be presented in the following bulky form: |
|||||||||||||||||
|
|
|
|
|
|
|
i |
|
|
|
|
|
|
|
|
|
|
|
C |
0 |
|
|
A B |
C0 C0 |
L |
|
|
|
(29) |
||||||||
|
i |
|
U |
U |
|
|
|
|||||||||||
U |
|
|
|
|
iU |
|
|
|
iL |
|
|
|
|
|||||
where |
|
|
|
|
|
|
|
|
|
|
|
|
|
|
|
|||
C0 |
b1U (a22 a21) b2U (a11 a12) |
|
(30) |
|||||||||||||||
|
iU |
|
|
|
|
a11a22 a12a21 |
|
|
|
|
||||||||
|
|
|
|
|
|
|
|
|
|
|
|
|||||||
C0 |
b2L (a11 a12) |
|
|
|
|
|
|
|
|
(31) |
||||||||
|
iL |
|
a11a22 a12a21 |
|
|
|
|
|
|
|
|
|||||||
|
|
|
|
|
|
|
|
|
|
|
Manuscript |
|||||||
|
|
|
K 2 K 2 4 j / D |
|
|
|||||||||||||
r1,2 |
|
|
|
|
|
|
|
|
|
|
|
|
i |
|
|
|
(32) |
|
|
|
|
|
|
|
2 |
|
|
|
|
|
|
|
|
||||
|
|
|
|
|
|
|
|
|
|
|
|
|
|
|
|
|
|
|
a11 (r1 K)Di |
k5 , |
|
|
a12 (r2 |
K)Di k5 , |
|
(33) |
|||||||||||
a |
|
(r K)D er1L , |
a |
|
|
(r K)D er2L |
|
|||||||||||
21 |
22 |
|
|
|||||||||||||||
|
|
|
|
1 |
|
i |
|
|
|
|
|
2 |
|
i |
|
|
||
b |
|
k |
5 |
a |
C 0 , |
b |
|
k |
a |
, |
b |
k b |
|
(34) |
||||
1U |
|
|
5 |
i |
2U |
|
2 |
|
2 |
|
2 L |
|
2 2 |
|
|
|||
|
|
|
|
The reader should note that the expressions given above for cation interstitials are exactly |
||||||||||||||
the same for oxygen vacancies, with the oxidation number, |
, being replaced by 2, Subscript 2 |
|||||||||||||||||
|
|
|
|
|
|
|
Accepted |
|
|
being replaced by Subscript 3, and Subscript 5 being replaced by Subscript 6, so as to identify the correct reactions in Figure 1.
12
Page 12 of 57
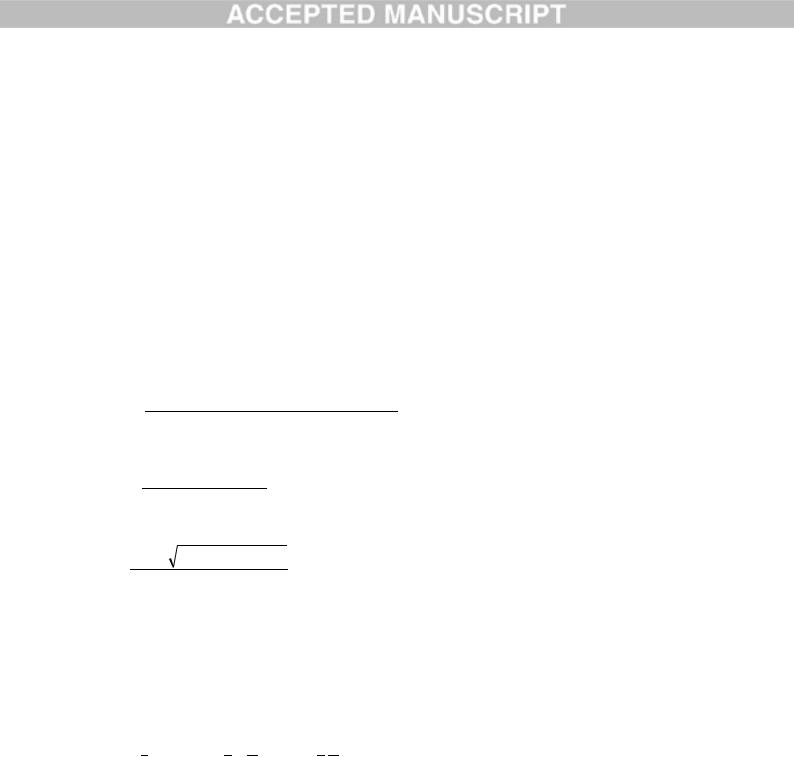
2.3. Calculation of |
CvL |
U |
By analogy it can be shown that:
CL |
|
Aer1L Ber2 L r2 L |
|
|
L |
L |
(35) |
||||||
|
|
U |
CL CL |
|
|
||||||||
U |
|
|
|
vV |
vL U |
|
|
|
|||||
where |
|
|
|
|
|
|
|
|
|
|
|
|
|
CvUL |
|
(b |
a |
|
b a )er1L |
(b a b |
a |
|
)er2L |
(36) |
|||
1U |
|
22 |
|
2U 12 |
2U 11 |
|
1U |
|
21 |
|
|||
|
|
|
|
|
|
a11a22 a12a21 |
|
|
|
|
|
|
|
CvLL |
|
b a er2L |
b a er1L |
|
|
|
|
|
|
(37) |
|||
2L |
11 |
|
|
2L 12 |
|
|
|
|
|
|
|||
|
|
a11a22 a12a21 |
|
|
|
|
|
|
|
|
|
K 2 K 2 4 j / D |
|
|
|
|
|
|||||
r1,2 |
|
|
|
|
v |
|
|
|
|
(38) |
||
|
|
|
2 |
|
|
|
|
|
||||
|
|
|
|
|
|
|
|
|
Manuscript |
|||
|
|
|
|
|
|
|
|
|
|
|||
where |
|
|
|
|
|
|
|
|
|
|
||
a11 (r1 K)Dv , a12 (r2 |
K)Dv , |
|
|
|
(39) |
|||||||
a |
[(r K)D k ]er1L , |
a |
[(r K)D k ]er2L |
|||||||||
|
||||||||||||
21 |
|
1 |
|
v 1 |
22 |
2 |
|
v |
1 |
|
||
b |
k |
a |
, b |
k a C L , |
b |
k C |
Lb |
|
|
(40) |
||
1U |
|
4 |
4 |
2U |
1 1 v |
2L |
1 v 1 |
|
|
|
||
|
|
|
|
Accepted |
|
|
|
By substituting Equations (22), (29) and Equation (35) into Equation (16) we have the final result
Y 0 |
I |
U |
I |
L |
I L ( C L |
C L |
L |
) I 0 |
( C 0 |
C 0 |
L ) |
(41) |
|
F |
|
|
L U |
v |
vU |
vL |
U |
i |
iU |
iL |
U |
|
If k1 = 0, we have
13
Page 13 of 57
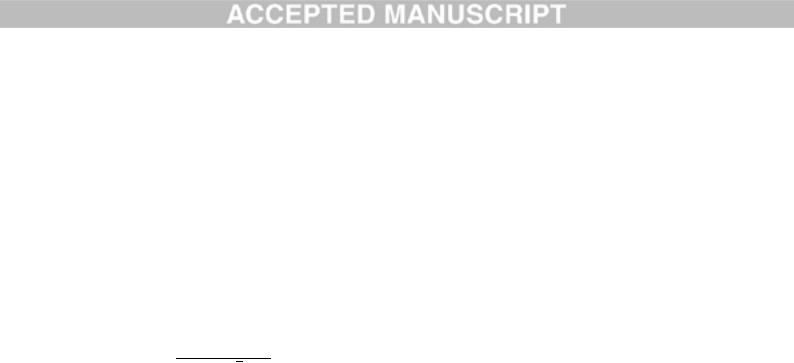
Y 0 |
I |
U |
I |
L |
I 0 |
( C 0 |
C 0 |
L ) |
(42) |
F |
|
|
L U |
i |
iU |
iL |
U |
|
If, in addition, we can neglect changes in the cation vacancy concentration (for example, at high frequencies) or χ = δ, we have
Y 0 |
I |
U |
I |
L |
(43) |
F |
|
|
L U |
|
where constants IU and IL are described by Equations (17) and (18). In the simplest case, when it is possible to neglect changes in the thickness of boundary layer (for example when L
is less than diameter of the iron atom), the impedance of the barrier layer reduces to the ohmic
resistance ZF0 1/YF0 IU .
Y 0 |
I |
I |
|
(k3a3 k7a7 ) |
(44) |
|
F |
U |
|
L |
j k b |
Manuscript |
|
|
|
|
|
3 |
3 |
|
|
|
|
|
|
|
The readerAcceptedwill again note that the expression for the current, Equation (1), does not contain the concentration of oxygen vacancies. This is, because Reaction (3), Figure 1, for the
generation of oxygen vacancies is considered to be irreversible and because Reaction (6) does not contribute to the electron current, as previously noted. However, physically, the transport of oxygen vacancies across the barrier layer still occurs, and hence is expected to impact the film growth rate at the m/bl interface and hence the current from that process, and may determine the total passive current density if the oxygen vacancy were found to be the dominant defect in the barrier layer. In the case of iron, the dominant defect is the metal interstitial. Importantly, it should be noted, as has already been done, that the equations describing the transport of oxygen vacancies are identical with those describing the transport of metal interstitials, except that the charge χ is replaced by 2. Thus, the contributions to the impedance of each of these defects are
14
Page 14 of 57

indistinguishable, even if oxygen vacancies appeared in the formal analysis. However, in this particular case, where δ = χ = 3, even the concentration of the interstitial does not enter the problem, because of Equation (1) and because rate of Reaction (2), Figure 1, is also considered
to be irreversible and hence does not depend on the interstitial concentration at the m/bl interface. Thus, the Faradaic impedance does not include a relaxation for the concentration of any defect, but transport clearly occurs. In order to overcome this artifact, we added a Warburg impedance for defect motion in accordance with Chao, Lin, and Macdonald [22].
Z |
W |
1/ 2 |
j 1/ 2 |
(45) |
|
|
|
|
where is the Warburg coefficient and is the angular frequency. As previously shown [22], the Warburg coefficient can be written as
D1/ 2 / 21/ 2 (1 )I |
ss |
(46) |
|
Manuscript |
|
|
|
barrier layer, D is the defect diffusivity, and is the polarizability of the barrier layer/solution interface. Parenthetically, the Warburg coefficient may be used to obtain the diffusivity of the defect within the barrier layer, by rearranging Equation (46) to yield:
where Iss |
is the steady-state passive current density, is the electric field strength within the |
|
Accepted |
D 2 2 1 2 Iss2 / 2 (47)
as is shown below.
It is important to note that the electronic defect structure of the barrier layer, and hence the electronic character (whether p-type or n-type) is defined by the distributions of the defects across the layer. This is because cation vacancies are electron acceptors, which dope the barrier
15
Page 15 of 57
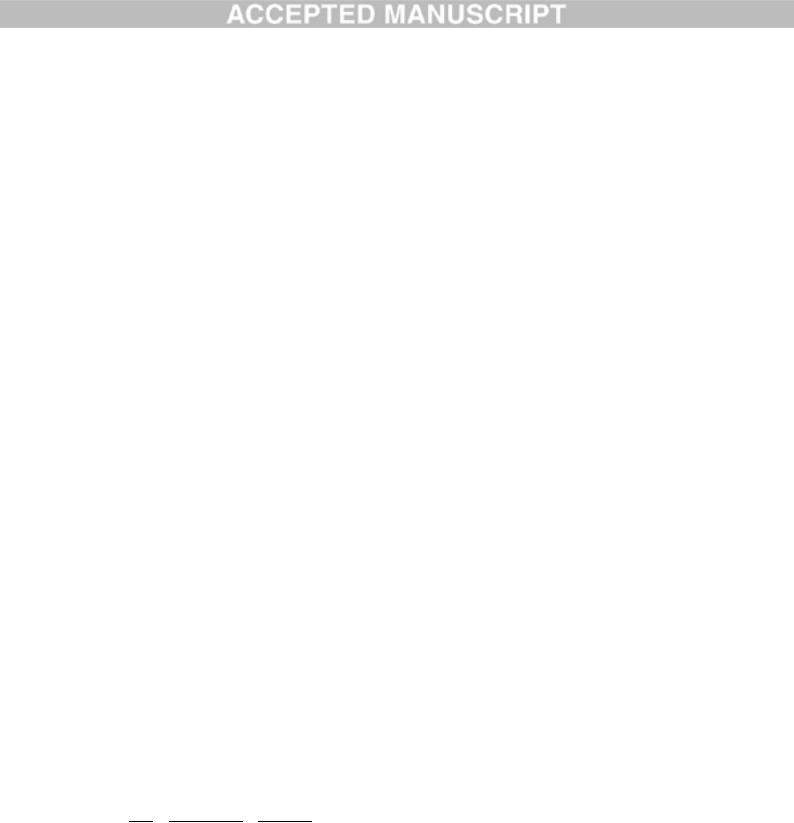
layer p-type, whereas cation interstitials and oxygen vacancies are electron donors, thereby doping the barrier layer n-type.
Estimation of defect distributions within the barrier layer in the steady state and in the general case can be performed via analytical solution of linear balance equations (see below). Accordingly, by knowing the kinetic parameters obtained from the EIS studies, it would be possible to calculate the concentrations of the defects in the barrier layer and hence to predict the electronic character.
The electrochemical reactions that occur atManuscriptthe metal/film and film/solution interfaces of the barrier layer (Figure 1) contribute to the impedance of a system. Therefore, the total
3. Impedance Model
impedance of the system, which comprises all of the contributory interfacial phenomena, including those at the metal/film/solution interphase plus the resistance of the solution can be represented by an electrical equivalent circuit. Figure 2 shows the equivalent circuit for the
metal/film/solution interphasial system that is proposed, in order to analyze the passivity of iron.
Figure 2 (Electrical equivalent circuit)
The total impedance of the system is expressed by Equation (48):
|
1 |
|
1 |
|
1 |
|
1 |
(48) |
Z |
|
Ze Zrandles |
|
|
Rs |
|||
Zcg |
|
|
Z f Z w |
|
|
|||
|
|
|
|
|
|
|
|
|
|
|
|
Accepted |
|
where Zf is the Faradaic impedance associated with the reactions occurring at the metal/film and film/solution interfaces. Zw is the Warburg impedance associated with the transport of defects through the barrier layer, and is expressed by Equations (45) to (47), Ze is the electronic
16
Page 16 of 57

impedance, due to the transport of electronic defects (electrons and/or holes), and Cg is the geometrical capacitance of the barrier layer. In the present analysis, we represent the geometrical capacitance with a constant phase element (CPE), because, on a polycrystalline
Note that Zrandles exists in series with Ze, because theManuscriptredox reaction, which it represents, accepts the electronic charge that by-passes the Faradaic impedance, Z (Figure 2).
substrate, there is expected to exist a distribution of passive film thickness on the various grain faces exposed to the solution and hence it is expected that a distribution will exist in the capacitance of the film. Rs is the resistance of the electrolyte between the oxide layer and the tip of the Luggin probe, and Zrandles is the impedance associated with the redox reactions occurring at the barrier layer/solution interface and expressed by Equation (49)
Zrandles |
|
1 |
|
1 |
|
1 |
|
|
|
|
(49) |
||
|
Z Rct |
|
ZCdl |
|
reported by this group [39-41] an electronic resistance (Re,h) has been used instead of Zrandles in series with Ze. The role of Re,h is to short-circuit the Faradaic and defect Warburg impedances, as indicated by the electrical analog given in Figure 2. However, since free electrons do not exist in the solution, the electronic charge moving through the barrier layer must be accepted by some redox couple in the solution at the bl/solution interface. This redox couple could be H2/H+,
The electronicAcceptedimpedance, Ze, can be expressed as af complex number, the component values of which can be calculated from fundamental theory. In most of the previous studies
O2/H2O, or Fe3+/Fe2+, for example, depending upon the potential and the species present in the solution. This reaction possesses an impedance, which we describe here by a Randles equivalent electrical circuit, and which is included in Re,h. If Re,h is low, the majority of the current will
17
Page 17 of 57
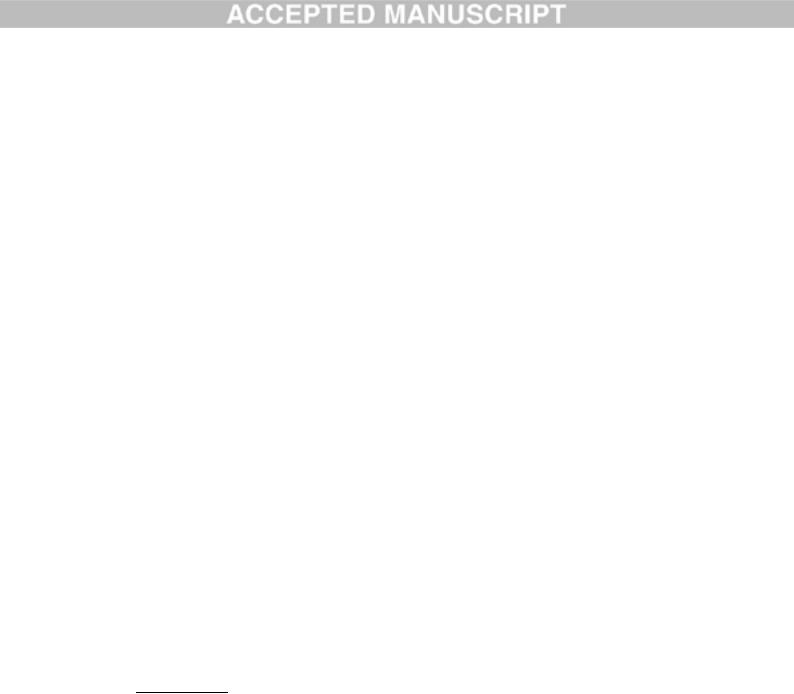
flow through this parallel path and not through Zf and Zw, with the consequence that the faradaic and defect transport impedances are “short circuited” and the analysis will become insensitive to the kinetic and transport processes involving the generation, annihilation, and transport of point
defects. This issue was recognized from the earliest development of the PDM [20-22]. Accordingly, our experiments over the past thirty two years have been carried out to render Re,h to be as large as possible, by excluding to the greatest extent possible all “tramp” redox species from the solution.
In series with the redox impedance, but in parallel with the Faradaic impedance (Zf) and
the defect Warburg impedance (ZW), Figure 2, is the electronic impedance of the film, due to the
ionization of electrons from donors (metal interstitials and oxygen vacancies) into the conduction
band and the generation of electron holes in the valence band by the acceptance of electrons from
the valence band by cation vacancies. Below we estimate the electronic impedance of the barrier |
||||||
|
|
|
|
|
|
Manuscript |
layer, Ze , on the basis of Bojinov et al.’s model [42-44]. In turn, and in accordance with this |
||||||
model, Ze is calculated by using the Young relation [45]: |
||||||
L |
|
|
dx |
|
|
|
Ze |
|
|
|
|
(50) |
|
e |
(x) j ˆ |
ˆ |
|
|||
0 |
|
0 |
|
|
|
|
where e (x) |
is the electronic conductivity profile in the barrier layer, ω is angular frequency, ˆ |
|||||
is the dielectric constant of the film, and ˆ0 |
is the dielectric permeability of the vacuum. The |
|||||
|
|
|
Accepted |
|
electronic conductivity of the barrier layer is proportional to the concentration of electronic charge carriers, Ce, i.e.
e e FCe |
(51) |
18
Page 18 of 57
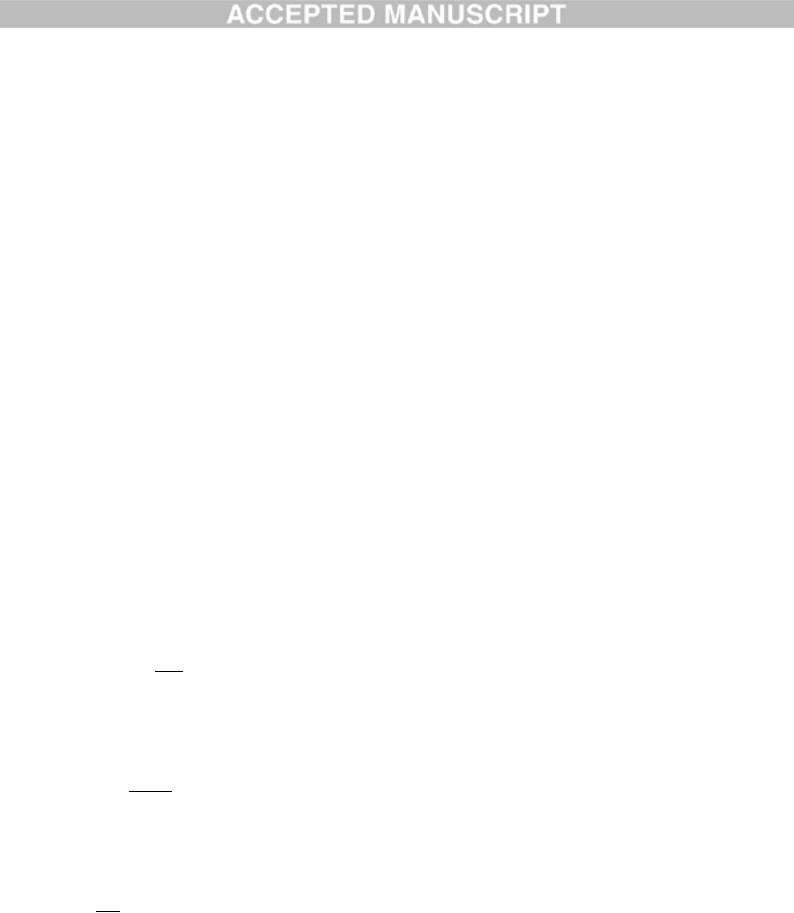
where e FDe / RT is the mobility of the electronic charge carriers, which is assumed to be
constant, and De is diffusion coefficient of electrons.
We will adopt the following approximation for Ce:
which simply states that the concentration of conducting electrons in the conduction band is directly determined by the concentration of electron donors in the barrier layer with each oxygen
vacancy and metal interstitial contributing 2 and electrons, respectively, to the conduction band.
In References [42-44], the concentrations of defects were calculated in accordance with the Fromhold and Cook equations [45]. Here, we will estimate Ci and CO on the basis of the
Manuscript(52)
|
|
|
|
|
Accepted |
|
||
PDM. In doing so, it is convenient to present flux densities of the ionic defect, Jk, in the film in |
||||||||
following form: |
|
|
|
|||||
J |
|
D |
dCk |
U |
C |
|
(53) |
|
|
k |
|
k |
dx |
k |
|
k |
|
where |
|
|
|
|
|
|||
Uk |
zk FDk |
|
|
|
(54) |
|||
|
|
|
RT |
|
|
|
|
|
has the dimension of velocity. Under steady-state conditions |
|
|||||||
dJk |
0 |
|
|
|
|
(55) |
||
dx |
|
|
|
|
|
|
and Equation (55) has the following solution
19
Page 19 of 57