
Encyclopedia of SociologyVol._4
.pdf
SCIENCE
Kühlhorn, Eckart, and Jill Björ, eds. 1998 Svenska alkoholvanor i förändring (Changing Swedish Drinking Habits). Stockholm: Sober.
Martinussen, Willy 1988 Solidaritetens grenser (Limits of Solidarity). Oslo: Universitetsforlaget.
——— 1993 ‘‘A Snapshot of Present-Day Norwegian Sociology,’’ Acta Sociology, 36, 305–392.
Mathiesen, Thomas 1987 Kan fengsel forsvares? (Can Prisons Be Justified?) Oslo: PAX; sec. ed. 1995.
Mjøset, Lars ed. 1992 Contributions to the Comparative Study of Development. Oslo: ISF, Report 92:2.
Norström, Thor 1988 ‘‘Alcohol and Suicide in Scandinavia.’’ British Journal of Addiction 83:553–559.
Østerberg, Dag 1986 Fortolkende sosiologi (Interpretive Sociology). Oslo: Universitetsforlaget.
——— 1988 Metasociology. Oslo: Norwegian University Press.
Ramsøy, Natalie R. 1998 ‘‘Eilert Sundt.’’
Rokkan, Stein 1987 Stat, nasjon, klasse (State, Nation, Class). In B. Hagtvet, ed., Oslo: Universietsforlaget.
———, Angus Campbell, Per Torsvik, and Henry Valen 1970 Citizens, Elections, Parties. Oslo: Norwegian University Press.
———, and Seymour M. Lipset, eds. 1967 Party System and Voter Alignments. New York: Free Press.
Segerstedt, Torgny 1955 ‘‘Kriminalsociologi’’ (Sociology of Crime). In Ivar Agge, ed., Kriminologi (Criminology). Stockholm: Wahlström and Widstrand.
———, and Agne Lundqvist 1952 and 1955 Människan i industrisamhället. del I och II (Man in Industrial Society. Parts I and II). Stockholm: SNS.
Skog, Ole-Jørgen 1985 ‘‘The Collectivity of Drinking Cultures.’’ British Journal of Addiction 81:365–379.
Sundbärg, Gustav 1913 Emigrationsutredningen (Investigation on Emigration). Stockholm: Offentligt betänkande. Twenty appendices 1908–1912.
Svalastoga, Kaare 1959 Prestige, Class, and Mobility. Co-
penhagen: Gyldendal.
——— 1965 Social Differentiation. New York: McKay.
Swedberg, Richard 1990 Economics and Sociology. Prince-
ton: Princeton University Press.
Therborn, Göran 1986 Why Are Some People More Unemployed Than Others? London: Verso.
——— 1995 European Modernity and Beyond. Newbury Park, Calif.: Sage.
Vogel, Joachim 1988 Inequality in Sweden. Stockholm: SCB.
Westermarck, Edvard A. 1891 History of Human Marriage, 3 vols. London: Macmillan.
———1906–1908 The Origin and Development of Moral Ideas. London: Macmillan.
———1932 Ethical Relativity. New York: Harcourt; paperback ed. 1960 by Littlefield.
CARL-GUNNAR JANSON
SCIENCE
Sociologists of science study the social organization of science, the relationships between science and other social institutions, social influences on the content of scientific knowledge, and public policy regarding science. The definition of the term ‘‘science’’ is problematic. Science can refer to a changing body of shared knowledge about nature or to the methods used to obtain that knowledge; in that form, science has existed for millennia. Research on ‘‘indigenous scientific knowledge’’ is reviewed in Watson-Verran and Turnbull (1995). Sociologists of science are more likely to define science in institutional terms, and most research in that area studies those who work in differentiated social institutions. The ‘‘demarcation’’ problem of distinguishing between science and nonscience persists. Gieryn (1995, 1998) argues that scientists and their advocates continually engage in contested ‘‘boundary work’’ to demarcate science. He discusses the rhetorical and organizational devices used in those contests; thus, scientists are likely to emphasize the disinterested search for knowledge in their attempts to distinguish science from technology and stress the utility of scientific knowledge in their attempts to distinguish it from religion. Gieryn argues against the notion that there are ‘‘essential’’ features of science that determine the outcome of those contests; these ‘‘essential features’’ are instead ‘‘provisional and contextual results of successful boundary-work’’ (1995, p. 406).
Unless the production of knowledge about the empirical world is delegated to relatively autonomous specialists, knowledge accumulates at a slow pace. When beliefs about nature are closely linked to major social institutions, institutional rigidity tends to produce cognitive rigidity (Znaniecki 1940; Parsons 1951, pp. 326–348). There were communities of relatively autonomous specialists in several great civilizations in the past, but most failed to produce stable institutions. Modern science dates from seventeenth-century Europe. Europe-
2455
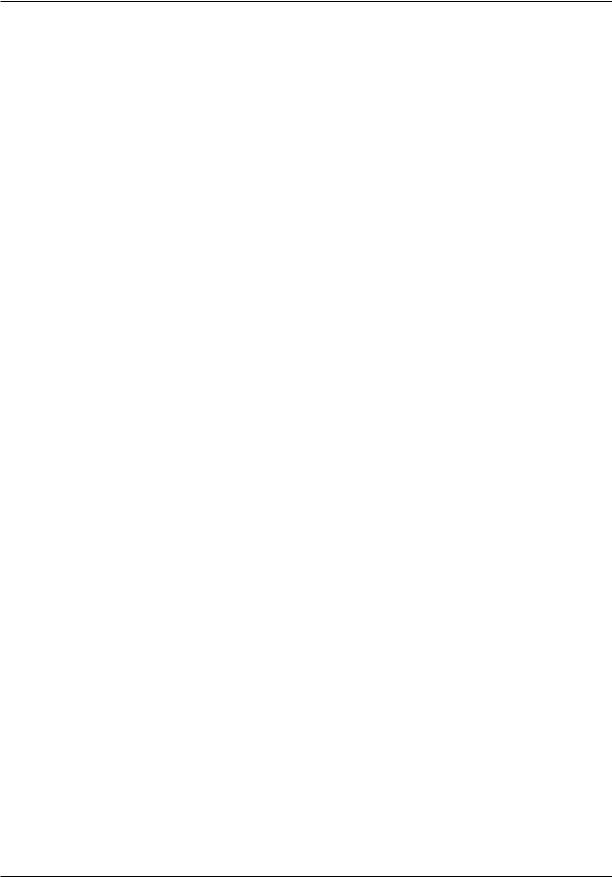
SCIENCE
ans at that time believed in a deity that gave laws to nature as well as to people and expected to discover those laws. Seventeenth-century Europeans could build on the basis of a science produced by the medieval schoolmen. With the rise of capitalism, intellectual elites developed a strong interest in using new knowledge to improve material conditions and enrich themselves. Merton, the leading founder of the sociology of science, argued in 1938 (1970) that in addition to these conditions, Puritanism contributed to the scientific ethos through its emphasis on work in the everyday world, rationalism and empiricism, openness to free inquiry, and desire to glorify God by describing His creation. (This still-controversial thesis is reviewed in a symposium in Isis 1988. For a general review of theories about the scientific revolution, see Cohen 1994.)
A distinctive normative ethos was institutionalized in modern science. Merton (1973, chap. 13) identified four salient norms: (1) ‘‘Universalism’’ requires that scientific contributions be evaluated according to general impersonal criteria without regard to ‘‘irrelevant’’ characteristics of the contributors such as their race, religion, and nationality. It also requires that scientists be rewarded according to their scientific contributions without regard for those irrelevant criteria. (2) ‘‘Communism’’ requires that knowledge be shared, not kept secret. Thus, the only way a scientist can claim a discovery as ‘‘his’’ or ‘‘hers’’ is to make it known to others. In this regard, modern scientists differ from Renaissance mathematicians and magicians, who were often quite secretive. (3) ‘‘Disinterestedness’’ refers to the injunction that the procedures and products of science not be appropriated for private gain. This need not imply altruism, although scientists often are driven to discover as an end in itself, but in addition, situations usually are structured so that it is in a scientist’s career interest to act in a disinterested manner. (4) ‘‘Organized skepticism’’ permits and encourages challenges to knowledge claims. Science tends to be unlike many other areas of social life, in which conformity in matters of belief is demanded as a sign of loyalty.
Merton’s essay on the normative ethos of science, first published in 1942, has drawn fruitful criticism. While Merton argued that the scientific ethos was functional for the advancement of knowledge, Mitroff (1974) argued that scientists could invoke ‘‘counter-norms,’’ for example, could fail
to be skeptical about their own theories, and this could be equally functional in some situations. Mulkay (1980) invoked ethnomethodological ideas to make an argument of general significance: ‘‘We should not assume that any norm can have a single literal meaning independent of the contexts in which it is applied. . . . Scientists must engage in inferential and interpretive work on norms. They are likely to do this after their actions, in order to construct acceptable accounts of their behavior. The norms don’t determine behavior.’’
Ambiguity involving the norm of universalism was present at the birth of modern science: Which characteristics of those who advance knowledge claims are relevant or irrelevant? Shapin (1994) argues that in England only the testimony of ‘‘gentlemen’’ was accepted as valid, and not all gentlemen; those who rejected the empiricism of men such as Francis Bacon and Robert Boyle and accepted the arguments from first principles of men such as Thomas Hobbes were excluded from the scientific community (Shapin and Shaffer 1985).
Scientists in the seventeenth and eighteenth centuries were usually amateurs, such as Robert Boyle and Benjamin Franklin, or the intellectual servants of amateurs. In the later eighteenth and nineteenth centuries, science was professionalized. Scientists received formal education in universities; found full-time employment, often in the universities; formed self-governing associations; and developed the modern scientific journal and other means of communication. A case study of the process for the newly emerging discipline of geology in the 1830s is presented in Rudwick (1985), where it is linked with the conduct of intense disputes about geological history; the ‘‘professionals’’ in those disputes got no special respect. The more general process of professionalization is described by Ben-David (1984, 1991), who notes the importance of national differences in the organization of science. Ben-David shows that there was more competition among universities in Germany and the United States in the late nineteenth and twentieth centuries than there was in those in Britain and France and claims that this partly accounted for the greater productivity of science in the first two countries. Other organizational characteristics of American science also help account for its superior productivity in the past half century: Science is not highly centralized, the competitive units are large enough and heterogeneous
2456
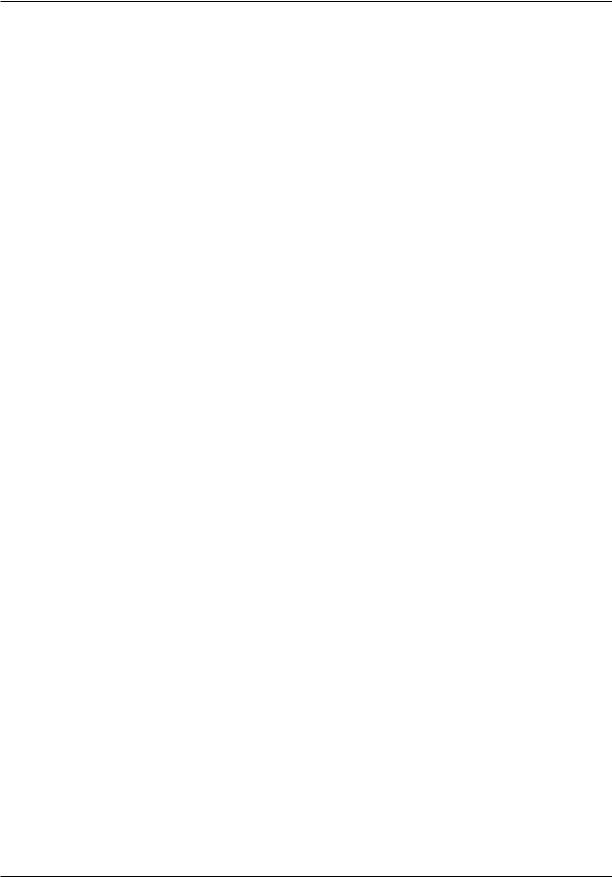
SCIENCE
enough to provide a critical mass of closely interacting scientists, and senior scientists tend to have less authority over younger scientists than they have elsewhere. (For statistics on national science productivity, see U.S. National Science Board 1996.)
SOCIAL STRATIFICATION IN SCIENCE
Competition remains intense among organizations that engage in basic research in the United States, particularly universities. Organizational prestige is central; as is usually true when it is difficult to measure organizational outputs directly, social comparisons become more important. Periodic surveys of faculty members have been used to rate the prestige of research departments. While outputs are difficult to measure, departments with high prestige are more successful in obtaining research resources and have higher rates of research productivity.
Competition is also intense among individual scientists, who compete for recognition from their peers for being the first to make valued discoveries (Merton 1973, chaps. 14 and 15; Hagstrom 1965, 1974). Competition may lead to secretive behavior and premature publication; it also may encourage scientists to move into new areas where there is less competition. A common consequence of competition is simultaneous or multiply independent discovery (see Zuckerman 1988 and the references cited there). The frequency of such events shows the extent to which science is a social product. When apparently simultaneous discoveries occur, those involved often engage in priority disputes; they are often ambivalent in those disputes, torn between a desire for the recognition due to originality and the demand for humility, the recognition of their dependence on the work of others.
There is a great degree of inequality in the research productivity of scientists. The chances that a scientist will publish as many as n papers is 1/n2; in other words, about 6 percent of all scientists produce 50 percent of all published papers (Price 1986). This inequality is even greater if one looks at the distribution of citations of the work of scientists. With some reservations, the number of citations can be taken as a measure of the quality of scientific work; frequently cited papers are usually those which other scientists have found useful in their own research. If c is the number of citations, the chances that the work of a scientist will have c
citations is proportional to 1/c³; that is, about 3 percent of all scientists receive 50 percent of all the citations of scientific papers.
Most of the variation in scientific productivity can be explained in terms of individual characteristics of scientists, such as years required to earn a doctorate, and characteristics of their employers, especially departmental prestige. While men have been more productive than women (the difference has been declining), that difference is almost entirely the result of differences in background and employer characteristics (Xie and Shauman 1998). In the United States (more than in most countries), there is considerable mobility of scientists among organizations. High research productivity predicts mobility to institutions of higher prestige and to a higher rank, but employment in a high-prestige organization in turn causes higher productivity (Allison and Long 1990). In general, American universities tend to conform to universalistic norms in making appointments and promotions (Cole and Cole 1973). There is an apparent exception to this in the early phases of careers, when productivity is difficult to assess; the prestige of a scientist’s doctoral department is strongly correlated with the prestige of the initial employer.
Inequality of productivity increases over the careers of scientists (Allison et al. 1982) as a manifestation of Merton’s (1973) ‘‘Matthew effect’’: ‘‘For unto every one that hath shall be given, and he shall have abundance: but from him that hath not shall be taken away even that which he hath.’’ Initially productive scientists obtain more and better resources for research, their work is more visible to others, and they are more likely to interact with other highly productive scientists.
WORK GROUPS, SPECIALTIES, AND
DISCIPLINES
Scientific research is a nonroutine activity; outcomes and problems cannot be predicted, and it is difficult to plan research. As organization theories lead one to expect in such situations, scientific work tends to be done in small groups with few hierarchical levels and a small degree of control by supervisors (Hagstrom 1976). Most basic research in universities is done by groups of four to nine graduate students and technicians led by one to a few professors. Over the course of time, faculty
2457
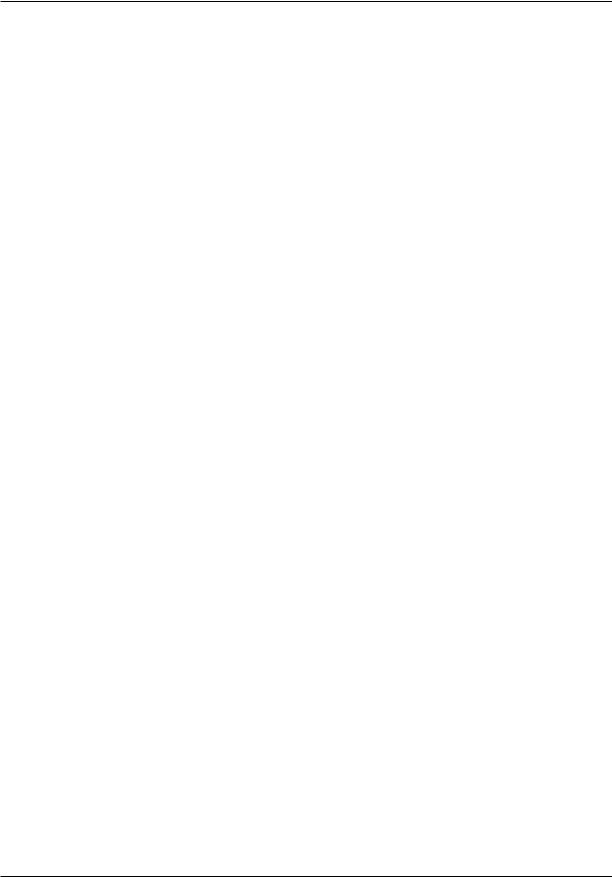
SCIENCE
members have found it increasingly desirable to collaborate with their peers, and most publications are multiply authored. Some aspects of research can be routinized, and the extent to which this can be done varies among disciplines; for example, work is more readily routinized in chemistry than it is in mathematics (Hargens 1975). Thus, work groups are smaller in mathematics than in chemistry. Chemists can delegate tasks to assistants, whereas mathematicians cannot; while the number of assistants does not explain much of the variation in the productivity of mathematicians, it does so in regard to the productivity of chemists. In other areas of science, major changes in research methods have led to what is called big science, which is epitomized by high-energy physics. Despite the use of labor-saving devices, the work groups at high-energy particle laboratories can be very large, with well over 150 scientists. Such groups have a greater division of labor, a broader span of supervisory control, and greater centralization of decision making.
These work groups ordinarily are embedded in larger organizations such as universities and governmental or industrial establishments. They also are likely to be linked informally with other groups working on the same or related research problems in other establishments. These loosely linked and loosely bounded sets of work groups can be called ‘‘specialties’’ or, more evocatively, ‘‘invisible colleges’’ (Price 1986). Groups in a specialty simultaneously compete with one another and make contributions to one another’s research. The number of groups in a specialty worldwide (there is a great deal of international collaboration) is ordinarily small, perhaps 50 on the average and seldom over 100, although specialties with over 500 groups exist. Scientists spend much of their time communicating with one another: writing papers, reviewing papers by others, attending meetings of scientific societies, and informally (Nelson and Pollock 1970).
The public nature of science tends to inhibit deviant behavior, but some deviance is to be expected. The extent of research fraud, such as forging and trimming data, is difficult to ascertain, as is the case in white-collar crime generally. Evidence and theories about such deviance are summarized in Zuckerman (1988). Fraud is most likely to occur when researchers are under pressure to get results (such as postdoctoral fellows and
nontenured faculty members) and when it is less likely to be detected (as in collaborative research with workers from different disciplines, where one is unable to evaluate the work of another); both conditions are especially likely to exist in experimental research in the biomedical sciences. Of courses, scientists with a high reputation also have engaged in research fraud; the case of the psychologist Cyril Burt is discussed in Gieryn (1998).
Scientific specialties usually exist within disciplines represented by their own university departments and scientific societies, but interdisciplinary research is common. The growth of an interdisciplinary area can lead to the differentiation of disciplines, and so the number of scientific disciplines has grown (Hagstrom 1965). The different scientific disciplines differ greatly in the degree of consensus about theories and methods; one indicator of this is variation in the rejection rates of manuscripts submitted to scientific journals, which is high in fields such as sociology and low in fields such as physics (Hargens 1975, 1988). Variations in consensus can affect the careers of scientists by affecting judgments of the merits of the work of individuals; it is easier to achieve early success in disciplines with a high degree of consensus. Disciplines also vary in the degree to which the work of scientists depends on and contributes to the work of others in their disciplines. This interdependence is related to Durkheim’s concept of ‘‘organic solidarity.’’ It is lower in mathematics than it is in the empirical sciences, as is indicated by fewer references in and citations of papers written by mathematicians, and it can be experienced as a problem by mathematicians (Hagstrom 1965).
THE SOCIOLOGY OF SCIENTIFIC
KNOWLEDGE
The Structure of Scientific Revolutions by the historian Kuhn (1970), first published in 1962, strongly influenced the sociology of science. Kuhn made a distinction between normal and revolutionary science. Normal science is a puzzle-solving activity governed by paradigms. A paradigm consists of shared commitments by a group of scientists to a set of values, presuppositions about nature, methods of research, symbolic generalizations such as Newton’s laws, and exemplars such as particular experiments. In normal science, researchers force nature into agreement with the paradigm; appar-
2458
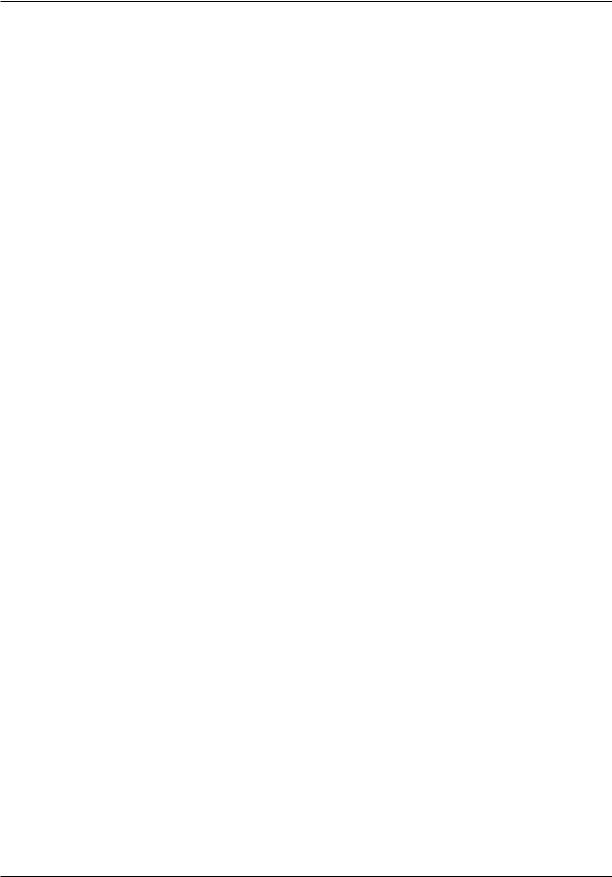
SCIENCE
ently disconfirming evidence does not weaken commitment to the paradigm. Normally scientists are successful in explaining away apparently disconfirming evidence, but persistent critical anomalies can trigger a scientific revolution. In a successful revolution, one paradigm is succeeded by another paradigm with quite different presuppositions and exemplars. Kuhn (1970) argued that the contending paradigms in revolutionary situations are ‘‘incommensurable’’; the choice between them is not and cannot be determined by evidence and formal decision rules alone. Kuhn illustrated his argument with evidence from major revolutions ranging from the Copernican Revolution of the sixteenth century to the revolutions that overthrew Newtonian physics in the twentieth century as well as smaller revolutions that affected the work of smaller sets of scientists.
The sociologists who developed the sociology of scientific knowledge, initially largely British, advanced radical arguments far beyond those of Kuhn. Not only are paradigms, or theories, ‘‘underdetermined’’ by data, theories are largely or entirely socially constructed. In Harry Collins’s words, ‘‘the natural world has a small or nonexistent role in the construction of scientific knowledge. . . . [N]othing outside the courses of linguistics, conceptual and social behaviour can affect the outcome of these arguments’’ (quoted in Gieryn 1982). The constructivists have done a number of detailed case studies of changes in the content of science to support their claims. Their early work is summarized in Collins (1983), who shows how ‘‘data’’ were insufficient for resolving conflicts about an allegedly new type of laser. Others have studied cases such as disputes about gravity waves, the construction of quarks, and the history of statistics and genetics in the early twentieth century. In an ethnographic study of a laboratory that investigated neurohormones, Latour and Woolgar (1979) describe how facts were socially constructed. For example, initial claims constitute conjectures, and lower-order factual statements are qualified by the names of those making the claims. However, when they are successfully constructed, these qualifications are dropped and the facts are taken for granted, perhaps embedded in laboratory equipment or algorithms. Related work by other sociologists has involved detailed analyses of scientific discourse. Gilbert and Mulkay (1984) studied biochemists who did research on the process of
oxidative phosphorylation. Those authors showed that the sober proseof the scientific papers, where evidence and argument lead to conclusions, was contradicted by the informal discourse of the same scientists, who were partly aware that evidence and argument would be insufficient to persuade their opponents.
The constructivist position naturally leads to a relativistic position: If theories are social constructs, they could equally well be different. From his detailed study of the ways in which physicists constructed quarks in the period 1964–1974, Andrew Pickering (1984) concluded that ‘‘there is no obligation upon anyone framing a view of the world to take account of what twentieth century physics has to say. The particle physicists of the late nineteen-seventies were themselves quite happy to abandon most of the phenomenal world and much of the explanatory framework which they had constructed in the previous decade. There is no reason for outsiders to show the present HEP worldview any more respect.’’ This relativism leads constructivists to challenge the conventional demarcation between science and nonscience or pseudoscience. Thus, an article reporting a study of parapsychologists was titled ‘‘The Construction of the Paranormal: Nothing Unscientific Is Happening.’’
These extreme claims have elicited much controversy. Representative criticisms by sociologists can be found in Gieryn (1982) and Amsterdamska (1990). Some natural scientists have argued that constructivism, along with several other ‘‘postmodern’’ schools of thought in the social sciences and humanities, represents a dangerous form of antiscientism; see Gieryn (1998) for a discussion of these ‘‘science wars.’’ Nevertheless, persuasive evidence has been produced about the importance of social factors in changing scientific knowledge. Stewart (1990) studied the recent revolution most widely known to the general public: plate tectonics in the 1950s and 1960s. He found strong resistance to the revolution. Earth scientists who had invested heavily in earlier perspectives were most likely to resist plate tectonics. Usually conversion to the new paradigm was gradual, sealed when scientists saw the relevance of the paradigm for their own research, but Stewart found some whose acceptance of plate tectonics came as the kind of ‘‘gestalt switch’’ described by Kuhn (1970).
2459

SCIENCE
In the conflicts accompanying the revolution, scientists on both sides deviated from conventional norms and used coercive methods to advance their positions and resist their opponents. Such intense conflict does not always accompany revolutions; in the one in physics that produced quarks, there was little acrimony or duress (Pickering 1984). In the earth sciences and physics, interests internal to the scientific disciplines affected the reception of theories. External interests also can have significant effects. Desmond (1989) shows how the interests of social classes interacted with religion in affecting the reception of Lamarckian ideas about evolution in England in the 1840s; the participants in the disputes were aware of the ideological implications of biological theories. Feminist sociologists of science have shown how gender interests have influenced perceptions of nature and the formulation of biological theories. See Keller (1995) for a review of some examples.
APPLIED RESEARCH AND DEVELOPMENT
The preceding discussion has concerned mostly basic research oriented primarily toward the advancement of knowledge. However, most research is done to advance other goals: corporate profits, weaponry, health, and human welfare. Of the 2 to 4 percent of their gross national products that advanced industrial countries devote to research and development (R&D), less than 10 percent is devoted to basic research (U.S. National Science Board 1996). Of the remainder, much is devoted to defense, particularly in the United States, where a substantial majority of federal R&D expenditures are devoted to that use.
Independent inventors are still an important source of innovations, but most applied scientists and engineers are salaried employees of corporations and mission-oriented government agencies. Such employees lack most of the autonomy of basic scientists. University-trained scientists are likely to chafe under this loss of autonomy, but successful applied research organizations have developed procedures for harmonizing their scientists’ desires for autonomy with an organization’s desire for useful knowledge (Kornhauser 1962). Engineers are important in translating knowledge into products and processes. Engineers are more pragmatic than scientists and are committed less
to paradigms and more to physical objects (when a scientist moves, he or she is likely to pack his or her journals first; when an engineer moves, she or he packs her or his catalogues). While scientists tend to seek autonomy in organizations, engineers tend to seek power; it is usually necessary to control organizational resources to do successful engineering work.
One of the conflicts that can occur between scientists and their industrial employers concerns communications. Scientists want to communicate their discoveries to their colleagues to gain recognition; their employers want to profit from the discoveries, and that may require keeping them secret. The patent system can provide an accommodative mechanism: Discoveries are made public, but those who wish to use the discoveries for practical purposes must pay royalties to the patent holder. The patent system represents one aspect of the commodification of knowledge. Marxist theories imply that in capitalist social formations, goods and services are produced for sale as commodities, not for use, and this is increasingly the case for scientific knowledge. Kloppenburg (1988) has applied Marxist thought effectively in his history of plant breeding. There were and are inherent problems in making seeds into a commodity, since seeds tend to reproduce themselves; they can be both objects of consumption and part of the means of production. Until recently, seeds seldom were produced as commodities; new varieties were exchanged among farmers or distributed to them by government agencies at little cost, and the farmers would then grow their own seeds. This changed first with the development of hybrid corn, where farmers could not use the corn they produced as seed and instead bought new seed from the seed companies each season. This process has since been extended to other crops. In addition, consistent with Marxist thought, the seed industry has become increasingly centralized and concentrated, with fewer and larger firms dominating it. Those firms also expand into world markets, acquiring germ plasm in third world countries and selling seeds as commodities in those countries. The development of biotechnology has increasingly taken this form. Rapid developments in this area blur the distinction between basic and applied research. The emerging pattern seems to be one in which research that cannot be used to generate a profit is done in universities and gov-
2460
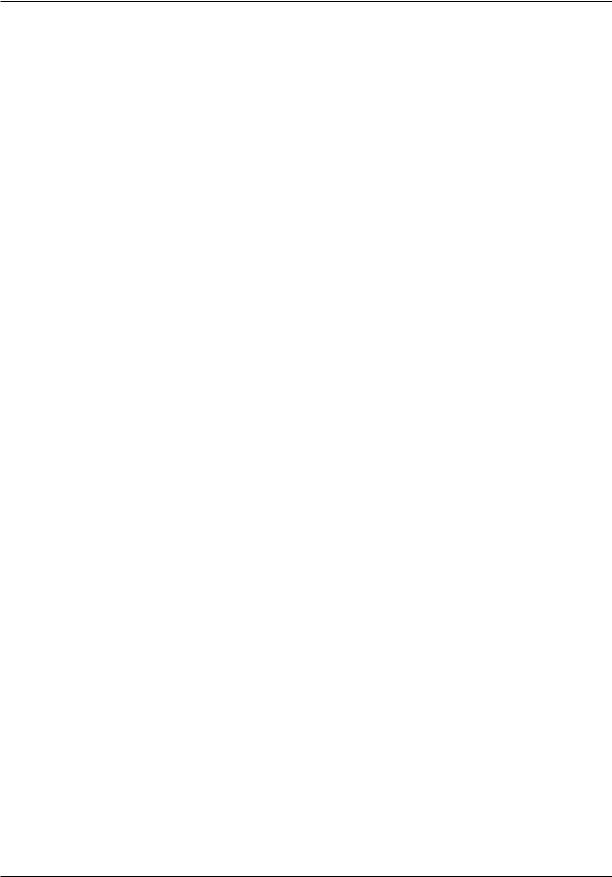
SCIENCE
ernmental agencies, usually at public expense, while research that can be used for profit is done in corporations.
Modern science has led to massive changes in the lives of people in all countries, and it has the potential for further changes. For example, it has made major contributions to economic growth (Mansfield 1991). However, not all these changes have been beneficial, and not all beneficial changes are allocated equitably. While polls show high support for science in general, there are intense public controversies in many areas, from the use of animals in biomedical research, to global warming, to military technologies (Nelkin 1995). Sometimes research and development efforts can achieve considerable autonomy. MacKenzie (1993) shows how those who developed the inertial navigation system for submarine-launched missiles successfully ‘‘black-boxed’’ their efforts so that political officials would not interfere. The navigation technology could have had seriously destabilizing effects in the cold war, without any deliberation by elected officials. The autonomy of engineers sometimes achieve does not imply autonomy for scientists. Thus, while oceanographers have made major discoveries in the past forty years, their expensive research has been driven largely by the interests of the U.S. Navy and their autonomy has been constrained by its interests (Mukerji 1990). Attempts have been made to develop more democratic means for developing science policy. Collingridge (1980) argues for an approach of ‘‘disjointed incrementalism’’: Since problems are rarely foreseen, policy making should be fragmented rather than centralized and will often be remedial; since it is not feasible to investigate all solutions, analysis and evaluation should be serial and incremental. Democratic governments have developed organizations to mediate between science and governmental institutions. These organizations can be nongovernmental, such as the National Academy of Sciences–National Research Council; part of the legislative branch, such as the Office of Technology Assessment of the U.S. Congress; or part of the executive branch, such as the Environmental Protection Agency. (For a description of these efforts in the United States and the difficulties they face, see Bimber and Guston 1995; Cozzens and Woodhouse 1995.) The growth and rapid change of science-based technologies present difficult problems in regard to support and
control. Knowledge about the organization of science and its relationships with other institutions can help in dealing with those problems.
REFERENCES
Allison, Paul D., and J. Scott Long 1990 ‘‘Departmental Effects on Scientific Productivity.’’ American Sociological Review 55:469–78.
———, J. Scott Long, and Tad K. Krauze 1982 ‘‘Cumulative Advantage and Inequality in Science.’’ American Sociological Review 47:615–625.
Amsterdamska, Olga 1990 ‘‘Review of Science in Action by Bruno Latour.’’ Science, Technology, and Human Values 15:495–504.
Ben-David, Joseph 1984 The Scientist’s Role in Society. Chicago: University of Chicago Press.
——— 1991 Scientific Growth: Essays on the Social Organization and Ethos of Science. Berkeley: University of California Press.
Bimber, Bruce, and David H. Guston 1995 ‘‘Politics by the Same Means: Government and Science in the United States.’’ In Sheila Jasanoff, G. Markle, J. C. Petersen, and T. Pinch eds., Handbook of Science and Technology Studies. Thousand Oaks, Calif. Sage.
Cohen, H. Floris 1994 The Scientific Revolution: A Historiographical Inquiry. Chicago: University of Chicago Press.
Cole, Jonathan R., and Stephen Cole 1973 Social Stratification in Science. Chicago: University of Chicago Press.
Collingridge, David 1980 The Social Control of Technology. London: Frances Pinter.
Collins, Harry M. 1983 ‘‘The Sociology of Scientific Knowledge: Studies of Contemporary Science.’’ In
Annual Review of Sociology, vol. 9. R. H. Turner and J. F. Short, Jr., eds. Palo Alto, Calif. Annual Review.
Cozzens, Susan E., and Edward J. Woodhouse 1995 ‘‘Science, Government, and the Politics of Knowledge.’’ In Sheila Jasanoff, G. Markle, J. C. Petersen, and T. Pinch, eds., Handbook of Science and Technology Studies. Thousand Oaks, Calif.: Sage.
Desmond, Adrian 1989 The Politics of Evolution. Chicago: University of Chicago Press.
Gieryn, Thomas F. 1982 ‘‘Relativist/Constructivist Programmes in the Sociology of Science: Redundance and Retreat.’’ Social Studies of Science 12:279–297.
———1995 ‘‘Boundaries of Science.’’ In Sheila Jasanoff, G. Markle, J. C. Petersen, and T. Pinch eds., Handbook of Science and Technology Studies. Thousand Oaks, Calif.: Sage.
2461
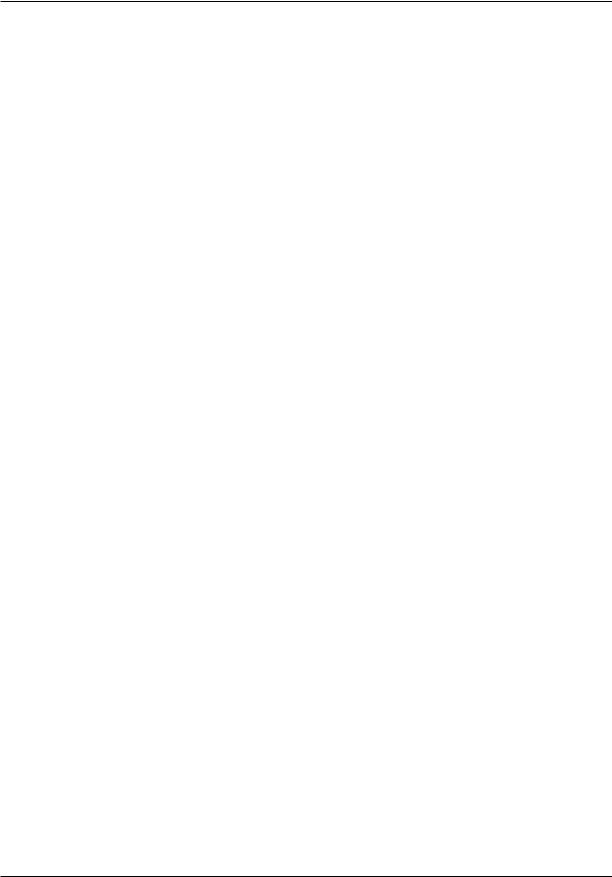
SCIENCE
——— 1998 Cultural Boundaries of Science. Chicago: University of Chicago Press.
Gilbert, G. Nigel, and Michael Mulkay 1984 Opening Pandora’s Box: A Sociological Analysis of Scientists’ Discourse. Cambridge, UK: Cambridge University Press.
Hagstrom, Warren O. 1965 The Scientific Community. New York: Basic Books.
———1974 ‘‘Competition in Science.’’ American Sociological Review. 39:1–18.
———1976 ‘‘The Production of Culture in Science.’’
American Behavioral Scientist 19:753–768.
Hargens, Lowell L. 1975 Patterns of Scientific Research: A Comparative Analysis of Research in Three Scientific Fields. Washington, D.C.: American Sociological Association.
——— 1988 ‘‘Scholarly Consensus and Journal Rejection Rates.’’ American Sociological Review 53:139–151.
Isis 1988 Symposium on the Fiftieth Anniversary of
Science, Technology and Society, vol. 79, pp. 571–605.
Keller, Evelyn Fox 1995 ‘‘The Origin, History, and Politics of the Subject Called ‘Gender and Science.’’’ In Sheila Jasanoff, G. Markle, J. C. Petersen, and T. Pinch eds., Handbook of Science and Technology Studies. Thousand Oaks, Calif.: Sage.
Kloppenburg, Jack R., Jr. 1988 First the Seed: The Political Economy of Plant Biotechnology 1492–2000. New York: Cambridge University Press.
Kornhauser, William 1962 Scientists in Industry: Conflict and Accommodation. Berkeley: University of California Press.
Kuhn, Thomas S. (1962) 1970 The Structure of Scientific Revolutions. Chicago: University of Chicago Press.
Latour, Bruno, and Steve Woolgar 1979. Laboratory Life: The Social Construction of Scientific Facts. Beverly Hills, Calif.: Sage.
MacKenzie, Donald A. 1993 Inventing Accuracy: A Historical Sociology of Nuclear Missile Guidance. Cambridge, Mass.: MIT Press.
Mansfield, Edwin 1991 ‘‘Academic Research and Industrial Innovation.’’ Research Policy 20:1–12.
Merton, Robert K. (1938) 1970. Science, Technology and Society in Seventeenth Century England. New York: Howard Fertig.
——— 1973 The Sociology of Science. Chicago: University of Chicago Press.
Mitroff, Ian 1974 ‘‘Norms and Counternorms in a Select Group of Scientists: A Case Study in the Ambivalence of Scientists.’’ American Sociological Review
39:579–595.
Mukerji, Chandra 1990 A Fragile Power: Scientists and the State. Princeton N.J.: Princeton University Press.
Mulkay, Michael J. 1980 ‘‘Interpretation and the Use of Rules: The Case of the Norms of Science.’’ In T. F. Gieryn, ed., Science and Social Structure: A Festschrift for Robert K. Merton. New York: New York Academy of Sciences.
Nelkin, Dorothy 1995 ‘‘Scientific Controversies: The Dynamics of Public Disputes in the United States.’’ In Sheila Jasanoff G. Markle, J. C. Petersen, and T. Pinch eds., Handbook of Science and Technology Studies. Thousand Oaks, Calif.: Sage.
Nelson, Carnot E., and Donald K. Pollock, eds. 1970
Communication among Scientists and Engineers. Lexington, Mass.: Heath.
Parsons, Talcott 1951 The Social System. Glencoe, Ill.:
Free Press.
Pickering, Andrew 1984 Constructing Quarks: A Sociological History of Particle Physics. Chicago: University of Chicago Press.
Price, Derek J. de S. (1963) 1986 Little Science, Big Science
. . . and Beyond. New York: Columbia University Press.
Rudwick, Martin J. S. 1985 The Great Devonian Controversy: The Shaping of Scientific Knowledge among Gentlemanly Specialists. Chicago: University of Chicago Press.
Shapin, Steven 1994 A Social History of Truth: Civility and Science in Seventeenth-Century England. Chicago: University of Chicago Press.
———, and Simon Shaffer 1985 Leviathan and the AirPump: Hobbes, Boyle, and the Experimental Life. Princeton N.J.: Princeton University Press.
Stewart, John A. 1990 Drifting Continents and Colliding Paradigms: Perspectives on the Geoscience Revolution. Bloomington: Indiana University Press.
U.S. National Science Board 1985 Science and Engineer-
ing Indicators. Washington, D.C.: National Science
Foundation.
———, 1996 Science and Engineering Indicators. Washington, D.C.: National Science Foundation.
Watson-Verran, Helen, and David Turnbull 1995 ‘‘Science and Other Indigenous Knowledge Systems.’’ In Sheila Jasanoff, G. Markle, J. C. Peterson, and T. Pinch eds., Handbook of Science and Technology Studies. Thousand Oaks, Calif.: Sage.
Xie, Yu, and Kimberlee A. Shauman 1998 ‘‘Sex Differences in Research Productivity.’’ American Sociological Review 63:847–870.
Znaniecki, Florian 1940 The Social Role of the Man of Knowledge. New York: Columbia University Press.
2462
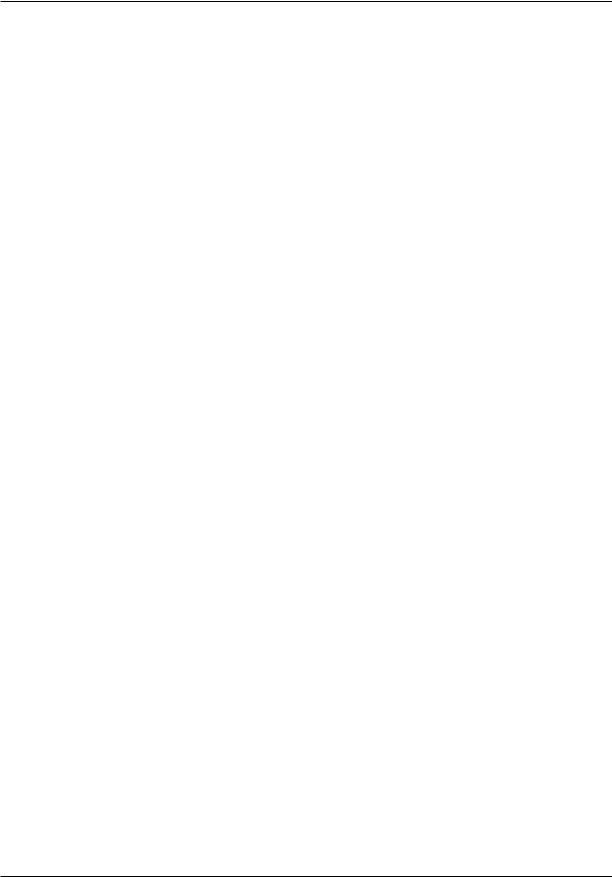
SCIENTIFIC EXPLANATION
Zuckerman, Harriet 1988. ‘‘The Sociology of Science.’’ In Neil J. Smelser, ed., Handbook of Sociology. Newbury Park, Calif.: Sage.
WARREN HAGSTROM
SCIENTIFIC EXPLANATION
Science and scientific knowledge achieved high status in twentieth-century Western societies, yet there continues to be disagreement among scientists and those who study science (historians, philosophers, and sociologists of science) about the meaning of scientific explanation. Indeed, the use of the word ‘‘explanation’’ has been the subject of heated debate (Keat and Urry 1982).
One way to make sense of science is to ‘‘reconstruct’’ the logic scientists use to produce scientific knowledge. The reconstructed logic of science differs from what scientists actually do when they engage in research. The research process is seldom as clear, logical, and straightforward as the reconstructed logic presented in this article makes it appear. For a long time, the most popular reconstruction of the logic of the scientific process was the ‘‘hypothetico-deductive’’ model. In this model, ‘‘the scientist, by a combination of careful observation, shrewd guesses, and scientific intuition arrives at a set of postulates governing the phenomena in which he is interested; from these he deduces observable consequences; he then tests these consequences by experiment, and so confirms or disconfirms the postulates, replacing them, where necessary, by others, and so continuing’’ (Kaplan 1964, pp. 9–10; see also Braithwaite 1968; Nagel 1961). The description of scientific explanation presented here is broadly consistent with this model as it is used in the social sciences.
Scientific explanations can be contrasted to other, nonscientific types of explanation (Babbie 1989; Kerlinger 1973; Cohen and Nagel 1934). Some explanations obtain their validity because they are offered by someone in authority, for example, a police officer, the president, or parents. Validity also may rest on tradition. For instance, the correct way to do a folk dance is the way it has always been danced, handed down over the generations. This knowledge is not obtained by going through textbooks or conducting experiments but is stored in the memories and beliefs of
individuals. Another way of knowing is a priori, or intuitive, knowledge. This knowledge is based on things that ‘‘stand to reason,’’ or seem to be obvious, but are not necessarily based on experience. People tend to cling strongly to intuitive knowledge even if the ‘‘facts’’ do not match their experience. Situations that contrast with strongly held beliefs are explained away as unique occurrences that will not happen again. For example, it ‘‘stands to reason’’ that if you are nice to other people, they will be nice to you.
The scientific method is a way of obtaining information, or knowledge, about the world. Theoretically, the same knowledge will be obtained by everybody who asks the same questions and uses the same investigative method. Scientific explanation uses theories, deductive and inductive logic, and empirical observation to determine what is true and what is false. Unlike authoritarian, traditional, or intuitive explanations, scientific knowledge is always supposed to be open to challenge and continual correction.
A theory is a hypothetical explanation for an observation or a question such as Why is the sky blue? or Why do victims of child abuse often grow up to be perpetrators? Scientists develop and test theories by using deductive logic, trying to show that empirical observations are instances of more general laws. Scientific theories are hypothetical explanations that state the possible relationships among scientific concepts. Theories consist of ‘‘a set of interrelated constructs (concepts), definitions, and propositions that present a systematic view of phenomena by specifying relations among variables, with the purpose of explaining and predicting the phenomena’’ (Kerlinger 1973, p. 9). Theories also are used by scientists to interpret, criticize, and bring together established laws, often modifying them to fit unanticipated data. They also guide the enterprise of making new and more powerful generalizations (Kaplan 1964, p. 295).
Scientific theories generally take the form of ‘‘If X happens, then Y will happen.’’ For instance, Karl Marx’s theory of surplus value suggests that as the level of surplus value in a capitalist society increases, so will inequality. This is an attempt to determine causal relations, so that theories not only predict what will happen in the world but also explain why it happens.
2463

SCIENTIFIC EXPLANATION
In general, scientific explanations are derived using nomothetic methods, which have the goal of making generalizations or of establishing universal laws. The experiment is perhaps the best known nomothetic method. Scientific theories try to generalize, or predict, beyond the specific data that support them to other similar situations. In contrast, some forms of the social sciences and humanities use idiographic methods, which are designed to provide knowledge of one particular event which may be unique. The best known idiographic method may be the case study. For example, both social scientists and historians investigate wars. A social scientist tries to explain what is common to all wars, possibly so that she or he can develop a general theory of intersocietal conflict. In contrast, a historian studies individual wars and tries to chronicle and explain the events and conditions that cause a specific war, and is generally not interested in a scientific theory of what may be common to all wars.
It seems that there is a paradox here: Scientific explanations are the best explanations that can be offered for an event, yet scientific theories are always open to correction by a better explanation or theory. What counts as a ‘‘better’’ explanation or theory has been the subject of debate in the philosophy of science. Some people believe that the better theories are those which can explain anomalies that previous theories could not. In other words, the new, ‘‘better’’ theory can explain everything the old theory could but also can explain some things that it left unexplained. There are many debates among philosophers of science about how to judge the ‘‘goodness’’ of a theory. They all admit that theories can never be confirmed definitively by any amount of observational material. The possibility always exists of finding an event that does not fit the theory, thus falsifying it. However, some theories have so much observational evidence on their side that they are said to be well confirmed, and the possibility of finding observations that falsify them is considered negligible.
However, the philosopher of science Popper said that while one can never absolutely confirm theories, one can definitively falsify them (1959). In other words, it is possible to find definite events that disconfirm, or falsify, a theory. However, other philosophers argue that this is not necessarily true, because it is always an open question whether it is the theory that is wrong or one of the
assumptions that is not tested when the theory is tested.
A famous example of this problem of falsification is provided by the philosopher of science Carl Hempel in his historical examination of the work of the Hungarian physician Semmelweiss (1966). Semmelweiss was concerned with the high rates of maternal mortality during childbirth. He theorized that those deaths resulted from blood poisoning, which was caused by infectious matter carried on the physician’s hands. Physicians were examining women right after performing dissections in the autopsy room. Semmelweiss’s hypothesis led him to believe that if the infectious matter was removed before the women were examined, the death rates would drop. To test this, he had doctors wash their hands in a solution of chlorinated lime after performing dissections and then examine women who had just given birth. As he predicted, the mortality rates fell as this procedure was practiced, providing evidence confirming his theory.
However, if the mortality rates had not fallen, that would not necessarily have meant that the theory was wrong. It could have meant that one of the unexamined assumptions, such as that chlorinated lime destroys infectious matter, was wrong. Thus, the theory would have been true but the experiment would not have provided evidence to confirm it because one of its untested assumptions was incorrect. Thus, falsification is a double-edged sword: When a theory is not confirmed, it is necessary to determine whether it is the thing that is being manipulated experimentally (the hand washing in chlorinated lime) that is the causal factor or whether one of the assumptions underlying the experiment is faulty (if it turned out that chlorinated lime did not kill infectious matter) (Hempel 1966, pp. 3–6). Scientists have to be careful not to give up on a theory too soon, even if early results appear to falsify it, because many major scientific achievements would not have occurred if they had been quickly abandoned (Swinburne 1964).
Whether philosophers of science hold to the confirmationist view or the falsificationist view of testing scientific theories, they agree on two things. The first is that scientific theories are universal statements about regular, contingent relationships in nature; the second is that the observations used to evaluate scientific theories provide an objective
2464