
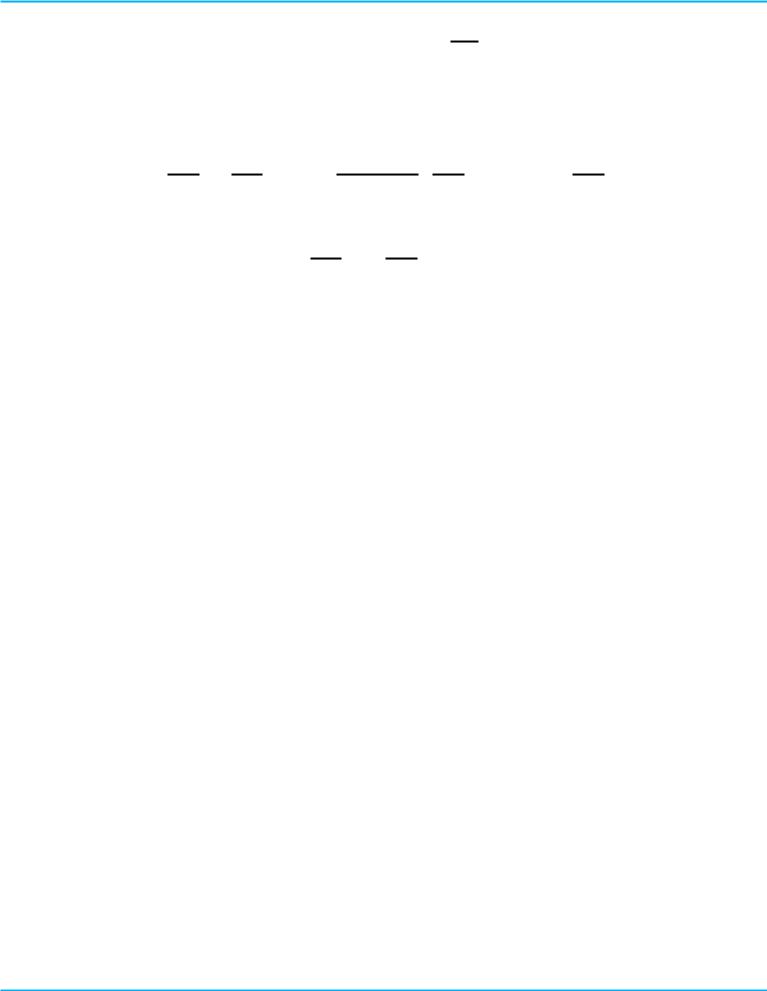
LABORATORY WORK #3
DETERMINATION OF ADIABATIC CONSTANT
PhysicVirtualLab Software Package
Substituting R= CP - CV and dividing by PV, we obtain
( )
Integrating this expression, we have
which is equivalent to Equation 8:
2.7 The Equipartition of Energy
We have found that predictions based on our model for molar specific heat agree quite well with the behavior of monatomic gases but not with the behavior of complex gases (see Table 1). The value predicted by the model for the quantity
, however, is the same for all gases. This is not surprising because this difference is the result of the work done on the gas, which is independent of its molecular structure.
To clarify the variations in CV and CP in gases more complex than monatomic gases, let us explore further the origin of molar specific heat. So far, we have assumed that the sole contribution to the internal energy of a gas is the translational kinetic energy of the molecules. However, the internal energy of a gas includes contributions from the translational, vibrational, and rotational motion of the molecules. The rotational and vibrational motions of molecules can be activated by collisions and therefore are “coupled” to the translational motion of the molecules. The branch of physics known as statistical mechanics has shown that, for a large number of particles obeying the laws of Newtonian mechanics, the available energy is, on the average, shared equally by each independent degree of freedom. The equipartition theorem
PhysicVirtualLabs Project Team, IITU
Copyright 2014

LABORATORY WORK #3
DETERMINATION OF ADIABATIC CONSTANT
PhysicVirtualLab Software Package
states that, at equilibrium, each degree of freedom contribute |
|
of energy per |
|
||
molecule. |
|
|
Table 1
Molar Specific Heats of Various Gases
Molar Specific Heat (J/mol × K)a
Gas |
|
|
|
⁄ |
|
|
|
|
|
|
|
|
|
|
|
Monatomic Gases |
He |
20.8 |
|
12.5 |
|
8.33 |
1.67 |
Ar |
20.8 |
|
12.5 |
|
8.33 |
1.67 |
Ne |
20.8 |
|
12.7 |
|
8.12 |
1.64 |
Kr |
20.8 |
|
12.3 |
|
8.49 |
1.69 |
|
|
Diatomic Gases |
|
|
||
H2 |
28.8 |
|
20.4 |
|
8.33 |
1.41 |
N2 |
29.1 |
|
20.8 |
|
8.33 |
1.40 |
O2 |
29.4 |
|
21.1 |
|
8.33 |
1.40 |
CO |
29.3 |
|
21.0 |
|
8.33 |
1.40 |
Cl2 |
34.7 |
|
25.7 |
|
8.96 |
1.35 |
|
|
Polyatomic Gases |
|
|||
CO2 |
37.0 |
|
28.5 |
|
8.50 |
1.30 |
SO2 |
40.4 |
|
31.4 |
|
9.00 |
1.29 |
H2O |
35.4 |
|
27.0 |
|
8.37 |
1.30 |
CH4 |
35.5 |
|
27.1 |
|
8.41 |
1.31 |
Let us consider a diatomic gas whose molecules have the shape of a dumbbell (Figure 7). In this model, the center of mass of the molecule can translate in the x, y, and z directions (Figure 7a). In addition, the molecule can rotate about three mutually perpendicular axes (Figure 7b). We can neglect the rotation about the y axis because
the molecule’s moment of inertia Iy and its rotational energy |
|
about this axis are |
|
negligible compared with those associated with the x and z axes. (If the two atoms are taken to be point masses, then Iy is identically zero.) Thus, there are five degrees of freedom for translation and rotation: three associated with the translational motion and two associated with the rotational motion. Because each degree of freedom
PhysicVirtualLabs Project Team, IITU
Copyright 2014

LABORATORY WORK #3
DETERMINATION OF ADIABATIC CONSTANT
|
|
|
|
|
|
|
|
|
PhysicVirtualLab Software Package |
||
|
|
|
|
|
|
|
|
|
|
|
|
contributes, on the average, |
|
|
of energy per molecule, the internal energy for a |
||||||||
|
|
||||||||||
system of N molecules, ignoring vibration for now, is |
|||||||||||
( |
|
|
) |
( |
|
) |
|
|
|
|
|
|
|
|
|
|
Figure 7 - Possible motions of a diatomic molecule: (a) translational motion of the center of mass, (b) rotational motion about the various axes, and (c) vibrational motion along the molecular axis [5.1]
We can use this result and Equation |
|
|
|
|
|
to find the molar specific heat |
||||||||||
|
|
|
|
|||||||||||||
at constant volume: |
|
|
|
|
|
|
||||||||||
|
|
|
|
|
|
|
|
( |
|
|
) |
|
|
|||
|
|
|
|
|
|
|
So,
PhysicVirtualLabs Project Team, IITU
Copyright 2014

LABORATORY WORK #3
DETERMINATION OF ADIABATIC CONSTANT
PhysicVirtualLab Software Package
These results agree quite well with most of the data for diatomic molecules given in Table 1. This is rather surprising because we have not yet accounted for the possible vibrations of the molecule.
In the model for vibration, the two atoms are joined by an imaginary spring (see Figure 7c). The vibrational motion adds two more degrees of freedom, which correspond to the kinetic energy and the potential energy associated with vibrations along the length of the molecule. Hence, classical physics and the equipartition theorem in a model that includes all three types of motion predict a total internal energy of
( |
|
) |
( |
|
) |
( |
|
) |
|
|
|
and a molar specific heat at constant volume of
( )
This value is inconsistent with experimental data for molecules such as H2 and N2 (see Table 1) and suggests a breakdown of our model based on classical physics.
It might seem that our model is a failure for predicting molar specific heats for diatomic gases. We can claim some success for our model, however, if measurements of molar specific heat are made over a wide temperature range, rather than at the single temperature that gives us the values in Table 1. Figure 8 shows the molar specific heat of hydrogen as a function of temperature. There are three plateaus in the curve. For low temperatures, the diatomic hydrogen gas behaves like a monatomic gas. As the temperature rises to room temperature, its molar specific heat rises to a value for a diatomic gas, consistent with the inclusion of rotation but not vibration. For high temperatures, the molar specific heat is consistent with a model including all types of motion.
Before addressing the reason for this mysterious behavior, let us make a brief remark about polyatomic gases. For molecules with more than two atoms, the vibrations are more complex than for diatomic molecules and the number of degrees of freedom is even larger. This results in an even higher predicted molar specific heat, which is in qualitative agreement with experiment. For the polyatomic gases shown in Table 1 we see that the molar specific heats are higher than those for diatomic gases.
The more degrees of freedom available to a molecule, the more “ways” there are to store energy, resulting in a higher molar specific heat.
PhysicVirtualLabs Project Team, IITU
Copyright 2014

LABORATORY WORK #3
DETERMINATION OF ADIABATIC CONSTANT
PhysicVirtualLab Software Package
Figure 8 - The molar specific heat of hydrogen as a function of temperature. The horizontal scale is logarithmic. Note that hydrogen liquefies at 20 K [5.1]
2.8 The Method of Clement and Desormes
The method of Clement-Dezormes to determine the specific heat ratio based on the measurement of gas pressure in the same vessel, which consecutively passes through three stages: from the first into the second gas becomes adiabatically, from the second to the third – isochorically (Figure 9).
Figure 9 - The dependence of the gas pressure P on the volume V to study the mass of gas
PhysicVirtualLabs Project Team, IITU
Copyright 2014
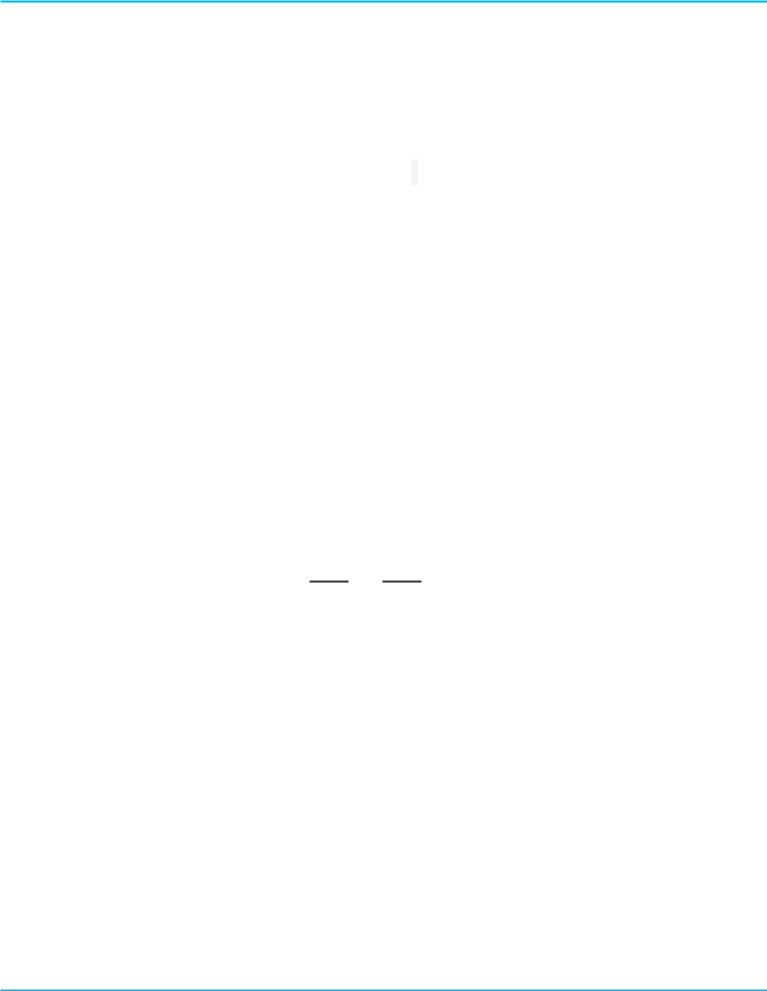
LABORATORY WORK #3
DETERMINATION OF ADIABATIC CONSTANT
PhysicVirtualLab Software Package
Let us consider this in more detail. In the glass bulb blown air to a pressure P1 which exceeds the atmospheric pressure P0 on the value of P', i.e.
P1 = P0 + P’ and P' ‹‹ P0
After the establishment of thermodynamic equilibrium with the surrounding medium temperature in the gas tank will be T1.
Thus, the initial state of the gas is determined by parameters P1, V1, T1. Then the balloon for a short time connected to theatmosphere, the pressure in the tank drops to a value of P2
equal to atmospheric pressure: P2 = Po.
The process of gas expansion is fast enough, so the heat exchange with the environment through the cylinder wall can be neglected and considered as an adiabatic process. The air in the balloon will change to state 2 with parameters P2, V2, T2, and V2> V1 and T2 <T1. During the adiabatic expansion some portion of the air will go into the atmosphere, so you must keep in mind that the V1 – is the amount of air in the state 1, a somewhat smaller volume of the cylinder. The relationship between the states 1 and 2 is determined by the Poisson equation:
(9)
on the other hand, the equation of state of ideal gas is:
(10)
Substituting the ratio of V1/V2 in (10) to (9), we obtain the relationship between pressure and temperature of the gas in these states:
|
( |
|
) |
( |
|
) |
(11) |
|
|
|
|
|
|||||
Later, after closing the valve, the air in the balloon isochoric heat to the ambient |
||||||||
temperature T1 and |
the pressure rises to |
a |
value of P3, which exceeds |
the |
||||
atmospheric P0 by |
a small amount P", |
i.e. the air moves to state 3 with |
the |
parameters P3, V2, T1, at this
P3 = P0 + P" and P"‹‹ P0
PhysicVirtualLabs Project Team, IITU
Copyright 2014

LABORATORY WORK #3
DETERMINATION OF ADIABATIC CONSTANT
PhysicVirtualLab Software Package
The relationship between states 2 and 3 we get from the equation of isochoric process:
(12)
From (12) and (11) taking into account the fact that P1 = P0 + P', and P3 = P0 + P'', we obtain
( |
|
) |
( |
|
) , |
|
|
( |
|
|
) |
( |
|
|
) . |
|
|
||||||
Since P'' ‹‹ P0 and P' |
‹‹ P0, |
, then, |
expanding both the binomial in a |
row and only terms of first order, we obtain
whence
(13)
Measuring the pressure p by water manometer and taking into consideration the fact that the pressure p of the liquid column is proportional to the fluid density ρ and height of the liquid in the manometer h, we conclude that P' – the pressure in the container before opening valve and the steady-state thermal equilibrium pressure P" – the pressure after closing the valve can be expressed as follows:
(14)
PhysicVirtualLabs Project Team, IITU
Copyright 2014

LABORATORY WORK #3
DETERMINATION OF ADIABATIC CONSTANT
PhysicVirtualLab Software Package
where g is acceleration of free fall. |
|
|
||||
Substituting equation (14) into equation (13), we |
obtain |
the calculation |
||||
formula for |
|
definitions: |
|
|
||
|
|
|
|
|
|
|
3. EXPERIMENTS DESCRIPTION
Experimental device
Experimental device (Figure 10) contains a large glass cylinder 1, which connected with pump due to tap 2. Pump is used to fill cylinder with air at open tap. U-formed manometer 3 is used to measure excess pressure. Tap 4 is used to discharge air from the cylinder and connect it with atmosphere.
Figure 10 - Experimental device
PROCEDURE
PhysicVirtualLabs Project Team, IITU
Copyright 2014
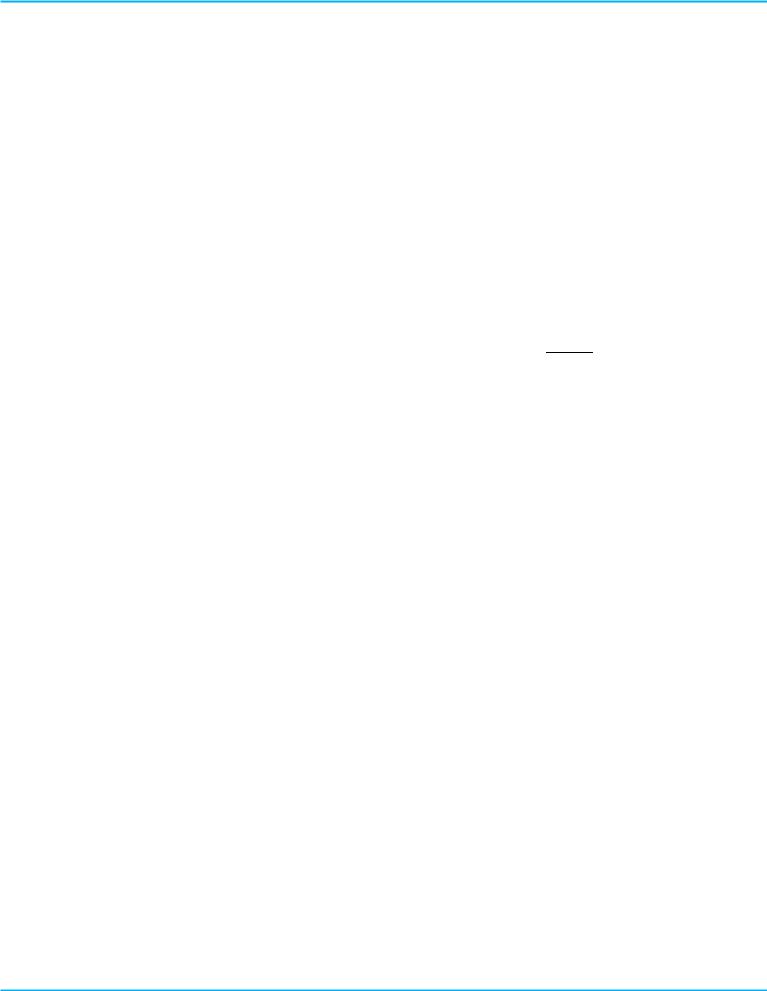
LABORATORY WORK #3
DETERMINATION OF ADIABATIC CONSTANT
PhysicVirtualLab Software Package
3.1.Close the cylinder (tap 4 is closed) and open tap 2. Pump air into cylinder until liquids difference in manometer get 300-350 mm.
3.2.Close the tap 2 and wait 3-5 minutes, until the temperature in cylinder equals with the room temperature (T of surrounding) and pressure will settle down. Measure height difference in the manometer h1.
3.3.Open the tap 4 quickly for short time and close again after the end of rushing of discharged air, which means that the pressure in the cylinder is equal to the atmospheric pressure.
3.4.Wait 3-5 minutes, air temperature in the cylinder is equal to the surrounding temperature. After that, measure the height difference in manometer h3.
3.5.Repeat the experiment 3-5 times by changing h1.
3.6.Fill the table with the readings from experiment.
3.7.Calculate the adiabatic constant of air by formula γ=
3.8.Estimate the absolute and relative error of measurements using Student’s method with α=0.95.
3.9.Write the results in the table 2.
Table # 2 |
|
|
|
|
|
|
|
|
|
|
|
|
|
|
|
|
|
|
Exp. |
|
|
|
|
|
|
|
|
N0 |
|
|
|
|
|
|
|
|
|
|
|
|
|
|
|
|
|
|
|
|
|
|
|
|
|
3.10. Compare the |
experimental γ with theoretical one. Theoretical γ can be |
||
calculated by |
|
|
. |
|
3.11.Discuss the results and draw the conclusion.
4.CONTROL QUESTIONS
4.1What is called: a) the heat capacity of the body, and b) the specific heat capacity of gas, and c) the molar heat capacity of gas? What units are they measured in?
4.2Is heat capacity a function of state or function of the process?
PhysicVirtualLabs Project Team, IITU
Copyright 2014

LABORATORY WORK #3
DETERMINATION OF ADIABATIC CONSTANT
PhysicVirtualLab Software Package
4.3What determines these heat capacities? (What is the difference between them?)
4.4What are the values of molar heat capacities Cp and Cv for air? What is the reason that Cp> Cv?
4.5What is the number of freedom degrees of the molecule?
4.6What is adiabatic process?
4.7What are the values of the adiabatic index γ: a) for a diatomic b) for triatomic and polyatomic ideal gas?
4.8What is the principle of Clement-Dezorma method for determining the adiabatic index?
4.9Write the first law of thermodynamics for an adiabatic process.
4.10What processes are described by the equations: PV = const; PVγ=const?
4.11How do the temperature and gas pressure change in the process of: a) the adiabatic expansion, and b) the adiabatic compression?
4.12What is the work of an adiabatic process?
4.13What are the values measured in the calculation of the adiabatic index?
4.14What expression is the value of the specific heat ratio γ determined by? a) Experimentally b) Theoretically?
4.15Derive the relation between Cp and Cv through the degrees of freedom.
4.16What are the causes of errors in this work?
4.17What is the internal energy of an ideal gas in terms of molecular-kinetic theory?
5.REFERENCES:
5.1Raymond A. Serway, John W. Jewett Physics for Scientists and Engineers, 2006.
5.2Irodov I.E. Fundamental laws of mechanics, 2001
PhysicVirtualLabs Project Team, IITU
Copyright 2014