
Solid Support Oligosaccharide Synthesis
.pdf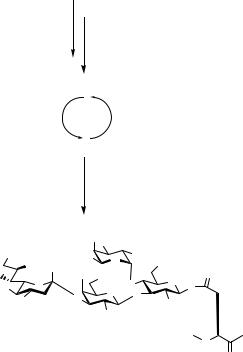
270 SOLID-PHASE SYNTHESIS OF BIOLOGICALLY IMPORTANT GLYCOPEPTIDES
synthesized starting with the coupling of galactosyl bromide 40 to a glucosamine azide 39 by activation with Hg(CN)2.
Neighboring-group participation at the 2 position of the galactosyl bromide selectively furnished the β-glycoside 41. Regioselective opening of the benzylidene group with NaCNBH3 in HCl/ether54 was followed by fucosylation to trisaccharide 43 employing ethylthiofucoside 42 as the donor and a variation of Lemieux’s in situ anomerization method described by Ogawa.55,56 After cleavage of the acetyl groups with NaOMe/MeOH, N-acetyl neuraminic acid was introduced in a regio/stereoselective (2→3) sialylation procedure furnishing sialyl Lewis A azide 45. Tetrasaccharide 45 was converted into the N-glycosylamino acid 47 by reduction with Raney nickel/H243 and subsequent coupling of the glycosylamine to the Fmoc-aspartic acid α-allylester 46. The C-terminal allyl ester was cleaved by Pd(0)-catalyzed allyl transfer to N-methyl aniline45 to give building block 47 ready for solid-phase glycopeptide synthesis. Octaglycopeptide 49 was synthesized using the HYCRON/TBTU methodology (Scheme 13.15).
Fmoc-Pro-HYCRON-bAla-TentaGel S
48
1.) morpholine, DMF;
Boc-Pro-OH, TBTU/HOBt, DIEA, sym-collidine, DMF; Ac2O, pyridine.
2.) TFA, DCM;
Fmoc-Glu(OtBu)-OH, TBTU/HOBt, DIEA, sym-collidine, DMF; Ac2O, pyridine.
1. morpholine/DMF 1:1
2. Fmoc-amino acid, TBTU/HOBt, DIEA, DMF
3. Ac2O, pyridine
Pd(PPh3)4, N-methylaniline, DMF/DMSO
|
|
BnOOBn |
|
|
|
|
|
|
OAc |
|
Me |
O |
OBn |
OBn |
|
|
|
|
OAc CO Me |
|
|
|
|
|||
|
|
|
|
|
|
|||
AcO |
2 |
OH OBn |
|
O |
H |
O |
|
|
O |
O |
|
||||||
AcHN |
|
O |
|
N |
|
|
||
AcO |
|
O |
O |
NHAc |
|
|
||
|
|
|
|
|
||||
|
|
|
OH |
|
|
|
||
|
|
|
|
|
|
|
|
|
|
Fmoc-Phe-Leu-Pro-Glu(OtBu) |
N |
Glu(OtBu)-Pro-Pro-OH |
|||||
|
|
|
|
|
|
|
|
|
|
|
|
|
49 |
|
|
H |
O |
|
|
|
|
|
|
|
Scheme 13.15 Solid-phase synthesis of a sequence from PSGL-1 carrying sialyl-Lewis A.
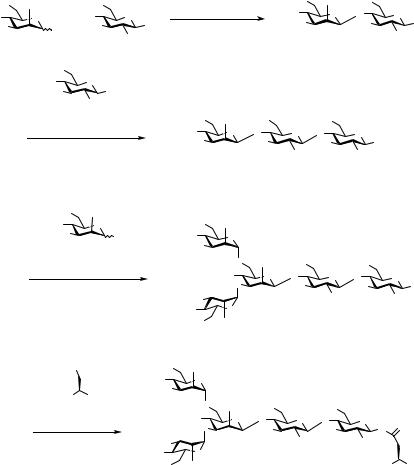
13.2 BUILDING-BLOCK APPROACH 271
13.2.2.2 A Glycopeptide Carrying an N-Linked Pentasaccharide Core CD52 is a GPI-anchored glycoprotein expressed on human lymphocytes. Monoclonal antibodies against CD52 are effectors of the complement-mediated lysis of cells. They have been used both in vivo and in vitro for the control of graft–host disease and for the prevention of bone marrow transplant rejection.57 CD52 is composed of a peptide containing only 12 amino acid residues and a N-glycosylated pentasaccharide chain.58 The synthesis of target compound 60 was performed using Fmoc amino acids and a carbohydrate building block 58 protected with benzyl ethers. Building block 58 was prepared starting from the monosaccharide building blocks 50 and 51 (Scheme 13.16).
AllO |
OBn |
BnO |
Silver alumina-silicate |
AllO |
OBn |
BnO |
|
|||||
BnO |
O |
O |
O |
|||||||||
|
|
|
|
|||||||||
BnO |
O |
+ |
HO |
O |
|
|
|
|||||
|
|
|
AllO |
|
BnO |
F |
||||||
AllO |
|
Br |
BnO |
F |
|
|
|
|
|
|
NPhth |
|
|
|
|
NPhth |
|
|
|
|
|
|
|||
|
|
|
|
|
|
|
|
52 |
||||
|
50 |
|
51 |
|
|
|
|
|
||||
|
|
|
|
|
|
|
|
|||||
|
|
|
|
|
|
|
|
|
|
|||
|
|
BnO |
|
|
|
|
|
|
|
|
|
|
|
1. |
HO |
O |
|
|
|
|
|
|
|
|
|
|
BnO |
N |
|
|
|
|
|
|
|
|
||
|
|
|
3 |
|
|
|
|
|
|
|
|
|
|
|
53 |
NPhth |
|
|
|
|
|
|
|
|
|
|
|
|
|
HO |
OBn |
BnO |
|
BnO |
|
|
||
|
|
Cp2HfCl2, AgClO4 |
|
|
|
|
||||||
|
|
|
BnO |
O |
O |
O |
O |
O |
|
|||
|
|
|
|
|
HO |
|
BnO |
|
BnO |
N3 |
|
|
|
2. a.) [Ir(COD)(PMePh2)2]PF6 |
|
|
54 |
NPhth |
NPhth |
|
|||||
|
|
|
|
|
|
|||||||
|
|
b.) HgO/HgCl2 |
|
|
|
|
|
|
|
|
||
|
|
BnO |
OAc |
|
|
|
|
|
|
|
|
|
|
1. |
BnO |
O |
|
BnO |
OH |
|
|
|
|
|
|
|
|
BnO |
Cl |
|
BnO |
O |
|
|
|
|
|
|
|
|
|
55 |
|
BnO |
|
|
|
|
|
|
|
|
|
|
|
|
O |
OBn |
BnO |
|
|
|
||
|
|
AgOTf |
|
|
BnO |
|
||||||
|
|
|
|
BnO |
O |
O |
O |
|
|
|||
|
|
|
|
|
|
O |
O |
|||||
|
2. a.) NH2CH2CH2NH2, n-BuOH |
|
O |
|
BnO |
BnO |
N3 |
|||||
|
BnO |
|
|
56 |
NHAc |
|
NHAc |
|||||
|
|
b.) Ac2O/MeOH (1:2) |
|
|
|
|
|
|
||||
|
|
|
BnO |
O |
|
|
|
|
||||
|
|
|
|
|
BnO |
OH |
|
|
|
|
|
|
|
1.) Lindlar catalyst/H2 |
BnO |
|
|
|
|
|
|
|
|||
|
|
HOOC |
|
OH |
|
|
|
|
|
|
||
|
2.) |
|
BnO |
O |
|
|
|
|
|
|
||
|
|
|
|
|
|
|
|
|
|
|||
|
FmocHN |
COOtBu |
BnO |
|
|
|
|
|
|
|
||
|
|
O |
|
BnO |
|
|
|
|
||||
|
|
57 |
|
OBn |
|
BnO |
|
|
||||
|
|
|
BnO |
O |
O |
O |
O |
O H |
|
|||
|
|
DCC/HOBt |
|
O |
||||||||
|
|
|
O |
|
BnO |
|
BnO |
N |
||||
|
3.) TFA/CH2Cl2 (1:2) |
BnO |
|
|
|
NHAc |
NHAc |
|
||||
|
O |
|
|
|
|
|
|
|||||
|
|
|
|
BnO |
|
|
58 |
|
|
|
||
|
|
|
|
BnO |
OH |
|
|
|
FmocHN |
COOH |
||
|
|
|
|
|
|
|
|
Scheme 13.16 Synthesis of an Asn-Core pentasaccharide buiding block.
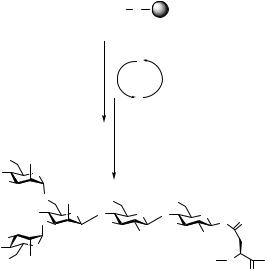
272 SOLID-PHASE SYNTHESIS OF BIOLOGICALLY IMPORTANT GLYCOPEPTIDES
For glycosylation of the glucosaminyl fluoride 51, the mannosyl bromide 50 was selectively activated by silver alumina–silicate. After purification, the disaccharide fluoride was directly used for a further glycosylation of a glucosamine azide 53 using Cp2HfCl2 and AgClO4 as the activating reagents. The trisaccharide was deallylated by an iridium-catalyzed isomerization59 and subsequent cleavage of the propenyl ether with HgO/HgCl2 to yield the β-mannosyl chitobiosamine derivative 54. α-Mannosylation of the two hydroxyl groups of 54 was achieved using mannosyl chloride 55 in the presence of silver triflate. Cleavage of the phthaloyl groups by ethylendiamine in n-butanol was followed by N-acetylation using Ac2O/MeOH (1:2). The azido function of 56 was reduced with H2 in the presence of Lindlar catalyst and the amine coupled to the Fmoc-amino acid 57 using DCC/HOBt as the condensing reagent. Cleavage of the tert-butyl ester gave building block 58 carrying exclusively benzyl protecting groups in the carbohydrate portion. Pentasaccharide asparagine
conjugate 58 was then used in the solid-phase synthesis of target structure 60 (Scheme 13.17).58c
Fmoc-Ser(tBu) O
59
1. 20% piperidine in NMP
2. Fmoc-amino acid, DCC, HOBt
1.95% aq. TFA, 2.5% HSCH2CH2SH
2.20% Pd(OH)2/C, H2, EtOH, H2O
HO |
OH |
|
|
|
|
|
|
HO |
O |
|
|
|
|
|
|
HO |
|
|
|
|
|
|
|
|
O |
OH |
HO |
HO |
|
|
|
|
HO |
O |
O |
O O |
O |
H |
O |
|
O |
|
HO |
HO |
|
N |
|
HO |
|
|
|
NHAc |
NHAc |
|
|
HO |
O |
|
60 |
|
|
|
|
HO |
OH |
|
|
H-GQ |
N |
DTSQTSSPS-OH |
|
|
|
|
|||||
|
|
|
|
|
|
H |
O |
|
|
|
|
|
|
|
Scheme 13.17 Solid-phase synthesis of CD52 glycopeptide.
The synthesis was performed on a 4-hydroxymethyl-phenoxymethylcopolystyrene- 1% divinylbenzene resin. Deprotection of the amino acids was achieved using 20% piperidine in N-methylpyrrolidone. Condensation reactions were carried out using DCC/HOBt as coupling reagents. After stepwise synthesis, the glycopeptide was released from the resin with concomitant cleavage of the acid-labile amino acid side-chain protecting groups using 95% TFA. Final deprotection of the carbohydrate moiety by hydrogenolysis catalyzed by 20% Pd(OH)2/C gave target compound 60.
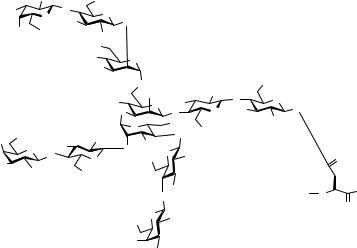
13.3 DIRECT SOLID-PHASE GLYCOSYLATION OF SOLID-SUPPORT-BOUND PEPTIDES 273
13.2.2.3 Synthesis of Glycopeptides Using Saccharide Units Isolated from Natural Sources Another approach to the synthesis of N-linked glycopeptides consists of the use of oligosaccharide building blocks that have been isolated from the natural sources fetuin and ribonuclease B by appropriate degradation and separation of the fragments.60 Such oligosaccharides can be obtained from the natural glycoproteins by a mild hydrazinolysis procedure that yields the unreduced forms of the carbohydrate moieties. Conversion of the carbohydrates to the β-glycosyl amines was achieved by treatment with a saturated solution of (NH4)HCO3, a method introduced by Kochetkov et al.61 Subsequent coupling to a suitable protected aspartic acid derivative gave the desired glycosyl asparagine conjugates. After carboxy deprotection, these building blocks could be used in solid-phase synthesis without protection of the carbohydrate hydroxyl functions to give glycopeptide structures such as 61 (Scheme 13.18). This approach enables an access to complex carbohydrate structures that still are not easily available by purely synthetic means.
|
OH |
O |
OH |
|
|
|
|
|
|
|
|
HO |
O |
O |
|
|
|
|
|
|
|
|
|
|
HO |
|
O |
|
|
|
|
|
|
|
|
OH OH |
|
NHAc |
|
|
|
|
|
|
|
||
|
|
|
|
|
|
|
|
|
|
||
|
|
|
HO |
|
|
|
|
|
|
|
|
|
|
|
HO |
O |
|
|
|
|
|
|
|
|
|
|
HO |
|
|
|
|
|
|
|
|
|
|
|
|
O |
|
NHAc |
OH |
|
|
||
|
|
|
|
|
OH |
|
|
|
|
||
|
|
|
HO |
|
O HO |
|
O |
O |
|
|
|
|
|
|
O |
|
O |
O |
HO |
NH |
|
|
|
|
|
|
|
|
NHAc |
|
|
||||
|
|
|
|
O |
|
OH |
OH |
|
|
|
|
HO OH |
|
|
NHAc |
|
O |
|
|
|
|
||
|
|
|
OH |
|
|
|
|
|
|||
HO |
O |
|
|
|
|
|
|
||||
O |
|
O |
NHAc |
|
|
|
|
||||
O |
|
O |
HO |
|
|
|
O |
||||
HO |
|
|
|
|
|
|
|||||
|
|
|
|
|
|
|
|
||||
|
|
|
|
|
|
|
|
|
|
|
|
OH |
|
OH |
|
|
|
|
|
|
|
|
|
|
|
|
|
|
|
OH |
H2N-Val-IleThr-Ala-Phe |
N |
Glu-Gly-Leu-Lys-OH |
||
|
|
|
|
|
|
O |
|
||||
|
|
|
|
|
|
|
|
|
H |
O |
|
|
|
|
|
|
|
|
|
|
|
||
|
|
|
|
|
O |
|
|
|
|
|
|
|
|
|
|
HO |
OH |
|
61 |
|
|
|
|
|
|
|
|
|
|
|
|
|
HO
OH
Scheme 13.18 N-linked glycopeptide with a triantennary structure.
13.3 DIRECT SOLID-PHASE GLYCOSYLATION OF SOLID-SUPPORT-BOUND PEPTIDES
A convergent method for the synthesis of glycopeptides consists of the direct coupling of oligosaccharides to preformed peptides. A solution-based synthesis of N-linked glycopeptides by acylation of β-glycosyl amines with partially protected aspartic acid–containing peptides has previously been reported.62 More recently, the direct O-glycosylation of peptides on solid phase has been published.63 Four different bisglycosylated O-glycopeptides were obtained by glycosylation of a set of peptide

274 SOLID-PHASE SYNTHESIS OF BIOLOGICALLY IMPORTANT GLYCOPEPTIDES
structures with different side-chain protection patterns bound to POEPOP (polyoxyethylenpolyoxypropylen)—a polyethylenglycol crosslinked resin— carrying the hydroxymethylbenzoic amide (HMBA) linker (Scheme 13.19).
Glycosylation of the free hydroxyl-functions of the polymer-bound peptides 62–65 was achieved by the trichloroacetimidate glycosylation procedure.13 After glycosylation using the first donor, the perbenzoyl-D-galactosyltrichloroacetimidate 66, the remaining tert-butyl ethers were cleaved and the hydroxyl functions thus set free were now glycosylated with the peracetyl-L-fucopyranosyl trichloroacetimidate 67. The four glycopeptides were detached from the resin by treatment with
OR1 OR2
Bz-Ile Ser-Gly-Ile-Ser-Gly-Ile-Gly
1.BzO OBz
O
BzO
BzOO NH
66
CCl3
TMS-OTf
2. TFA/H2O
HOOH
OH
H3C O
O
Bz-Ile Ser-Gly-Ile-Ser-Gly-Ile-Gly-OMe
H3C |
O |
O |
68 |
|
OH |
|
HOOH
|
HN |
62 R1 = H, R2 |
= H |
||
|
|
63 R1 |
= H, R2 |
= tBu |
|
O |
O |
64 R1 |
= tBu, R2 |
= H |
|
|
|
65 R1 |
= tBu, R2 |
= tBu |
|
|
HMBA |
POEPOP |
|
|
|
3.AcOOAc
H3C O OAc
O NH
67
TMS-OTf CCl3
4. NaOMe/MeOH
HOOH
OH
H3C O
O
Bz-Ile Ser-Gly-Ile-Ser-Gly-Ile-Gly-OMe
OH
HO |
O |
O |
69 |
|
|
||
|
|
|
|
HO OH |
|
|
|
|
HO OH |
|
HO OH |
|
||
|
|
HO |
O |
|
HO |
O |
O |
|
|
|
O |
|
|
||
|
|
|
OH |
|
|
OH |
|
|
Bz-Ile Ser-Gly-Ile-Ser-Gly-Ile-Gly-OMe |
|
Bz-Ile Ser-Gly-Ile-Ser-Gly-Ile-Gly-OMe |
||||
|
O |
O |
|
|
OH |
|
|
H3C |
70 |
HO |
O |
|
71 |
||
|
OH |
|
|||||
|
|
|
|
|
O |
|
|
HOOH HO OH
Scheme 13.19 Direct glycosylation of preformed peptides on solid phase.

13.4 CONVERGENT GLYCOPEPTIDE SYNTHESIS 275
NaOMe/MeOH with concomitant cleavage of the acetyl and benzoyl groups to furnish structures 68–71. This method takes advantage of the fact that the C-terminal amino acid is glycine. It offers the possibility of synthesizing glycopeptide libraries by means of solid-phase combinatorial chemistry. The optimal choice of the solid support is important. Previous attempts of direct solid-phase glycosylations of peptides bound to resins such as PEGA, TentaGel, Polyhipe, and Macrosorb resins via a rink linker or on polystyrene via the Wang linker failed.15
13.4 CONVERGENT GLYCOPEPTIDE SYNTHESIS WITH POLYMER-BOUND CARBOHYDRATES
The convergent synthesis of glycopeptides in which the carbohydrate moiety is attached to the solid support proved an efficient alternative methodology.64 The carbohydrate structure itself was synthesized on solid support via glycal assembly and anomeric amine synthesis (Scheme 13.20).
The galactosyl glycal 72 was bound to solid phase via a silyl ether linker. Epoxidation of the glycal using 2,2-dimethyldioxirane and subsequent electrophilic activation of the epoxide resulted in the glycosylation of 3,4-di-O-benzyl glucal 73. After acetylation of the product, the polymer-linked trisaccharide 74 was obtained.
O O
O O
O O
OH
O
O
O
72
|
OH |
|
|
|
|
|
|
|
|
|
|
BnO |
O |
|
O |
O |
|
|
|
|
|
|
BnO |
|
|
O |
O |
|
|
|
|
|
|
73 |
|
|
O |
|
|
|
|
||
|
|
|
|
|
|
|
|
|||
|
|
|
|
O |
|
|
1. I(coll)2ClO4, THF |
|||
|
1. DMDO/CH2Cl2 |
|
|
|
|
|||||
|
|
|
OAc |
|
|
|||||
|
|
|
|
|
AnthSO2NH2 |
|
||||
|
2. ZnCl2/THF |
|
|
O |
|
|
||||
|
|
|
|
|
|
O |
O |
|
2. Bu4NN3, THF |
|
O |
3. Ac2O, coll, THF |
|
|
|
||||||
|
|
O |
|
|||||||
|
DMAP (cat) |
|
|
|
|
|
OAc |
O 3. Ac2O, DMAP, THF |
||
|
|
|
|
|
|
|
|
|
|
|
|
|
|
|
|
|
74 |
BnO |
|
O |
|
|
|
|
|
|
|
|
BnO |
|
|
|
O |
O O |
|
|
|
|
|
O O |
|
|
|
O |
O |
|
|
|
O |
|
|
|||
|
O |
HS |
SH |
|
O |
|
|
|||
|
|
O |
|
|
||||||
|
OAc |
|
O |
|
||||||
|
|
|
|
|
|
|
|
|||
|
|
|
|
|
|
|
OAc |
|
||
|
|
|
|
|
|
|
|
|
||
|
|
O |
|
DIEA, DMF |
|
|
|
|
|
|
|
O |
|
|
|
|
O |
|
|
||
|
|
O |
|
|
|
|
|
|
||
|
|
O |
|
|
|
|
O |
O |
|
|
|
|
O |
|
|
|
|
|
|||
|
|
|
|
|
|
O |
O |
|||
|
|
|
OAc |
|
|
|
|
|
||
|
|
|
|
|
|
|
|
|
||
|
|
|
|
|
|
|
|
OAc |
|
|
|
|
|
BnO |
O |
|
|
|
|
|
|
|
|
|
|
|
|
|
BnO |
O |
||
|
|
|
BnO |
N3 |
|
|
|
76 |
||
|
|
75 |
|
AcNSO2Anth |
|
|
|
BnO |
NH2 |
|
|
|
|
|
|
|
|
|
NHAc |
||
|
|
|
|
|
|
|
|
|
Scheme 13.20 Solid-phase glycal assembly and anomeric amine synthesis.

276 SOLID-PHASE SYNTHESIS OF BIOLOGICALLY IMPORTANT GLYCOPEPTIDES
O |
O O |
|
|
|
|
|
|
O |
O O |
|
|
|
||
O |
O |
|
|
|
|
|
|
O |
O |
|
|
|||
|
|
|
Cbz-Ala-Leu-Asp-Leu-Ser(OBn)-OAll |
|
|
|||||||||
|
|
O |
|
O |
|
|
||||||||
|
|
|
|
|
|
|
||||||||
|
|
|
OAc |
|
|
77 |
|
|
|
|
OAc |
|
|
|
|
|
|
O |
|
|
|
|
|
|
|
|
O |
|
|
|
|
|
|
|
|
|
|
|
|
|
|
|
||
|
|
|
O |
|
O |
IIDQ, CH2Cl2 |
|
|
O |
O |
O |
|
||
|
|
|
O |
|
|
O |
|
|
|
|
|
O |
||
|
|
|
|
|
OAc |
|
|
|
|
|
|
|
OAc |
|
|
|
|
76 |
BnO |
O |
|
|
|
|
78 |
BnO |
O |
||
|
|
|
|
BnO |
NH2 |
|
|
|
|
BnO |
NH |
|||
|
|
|
|
|
|
NHAc |
|
|
|
|
|
|
NHAc |
|
|
|
|
|
|
|
|
|
|
|
|
|
|
Cbz-Ala-Leu-Asn-Leu-Ser(OBn)-OAll |
|
|
|
|
|
|
|
O OH |
|
|
|
|
|
|
||
|
|
|
|
|
|
O |
O |
|
|
|
|
|
|
|
|
|
|
|
|
|
O |
O |
|
|
|
|
|
||
|
|
|
|
|
|
|
|
|
|
|
|
|||
|
|
|
|
|
|
|
|
|
|
|
|
|
|
|
|
|
|
|
|
|
|
|
OAc |
|
|
|
|
|
|
|
1. Pd(PPh3)4, DMBA |
|
O |
O |
|
|
|
|
|
|||||
|
|
O |
|
O |
|
|
|
|
||||||
|
|
|
|
|
|
|
|
|
O |
|
|
|
|
|
|
2. H2N-Asp(OPMB)-Leu-Thr(OBn)-OAll |
|
|
|
|
|
|
|||||||
|
|
|
OAc |
|
|
|
|
|||||||
|
|
|
79 |
|
|
|
|
|
|
BnO |
O |
|
|
|
|
|
|
IIDQ, CH2Cl2 |
|
|
|
|
|
|
|
||||
|
|
|
|
|
80 |
|
BnO |
NH |
|
|
||||
|
3. HF-pyr., anisole |
|
|
|
|
|
NHAc |
|
|
|
||||
|
|
|
|
|
|
|
|
|
|
|||||
|
|
CH2Cl2 |
|
|
|
|
|
|
Cbz-Ala-Leu-Asn-Leu-Ser(OBn)-Asp(OPMB)-Leu-Thr-(OBn)OAll |
Scheme 13.21 Solid-phase synthesis of carbohydrate bound glycopeptides.
The conversion into the polymer-bound N-acetylglucosamine 76 suitable for coupling with a preformed peptide was achieved after iodosulfonamidation and a nucleophilic sulfonamide rearrangement induced by reaction with tetrabutylammonium azide. After N-acetylation of the sulfonamide, the acetylated sulfonamide was cleaved by 1,3-propanedithiol and Hünig’s base. Under these conditions concomitant reduction of the anomeric azide took place and furnished 76. Glucosamine 76 was now coupled to preformed peptides containing an aspartic acid residue. Using this strategy, asparagine N-glycopeptides such as 78 have been successfully synthesized (Scheme 13.21).
The orthogonal protecting group pattern of 78 allowed extension of the peptide chain on the solid support by fragment condensation with further peptide fragments. After Pd(0)-catalyzed cleavage of the allyl ester,65 the solid-phase-bound acid was coupled to the N-terminally deblocked tripeptide H-Asp(OPMB)-Leu- Thr(OBn)-OAll 79 to form the polymer-bound trisaccharide octapeptide, which, after release from the resin by treatment with HF/pyridine, gave the free glycopeptide 80.
A similar approach was used in the synthesis of the sialyl Lewis X mimetics of type 85 (Scheme 13.22).66 Protein crystallization,67 conformational studies of sLex in solution68 and in bound form to E- and P-selectin69 as well as the study of structure–function relationships70,71 gave information about the functional groups of the sLex-epitope essential for the binding to the selectins. Synthesized mimetics must contain the three essential hydroxyl functions of the fucose. Sialic acid, galactose, and
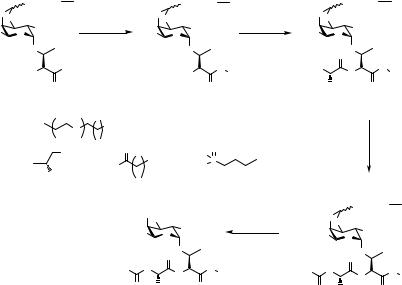
13.4 CONVERGENT GLYCOPEPTIDE SYNTHESIS 277
glucosamine were substituted by N- and C-terminal substitutions of threonine, which was itself bound to the fucose moiety by an α-glycosidic linkage. A fucosyl threonine building block was coupled to the solid support through its 3,4-cis-diol as an acetal. The combination of a p-(acyloxymethyl)benzylideneacetal anchor (p-AMBA) and a polyethylenglycol blockcopolymer (PEG-PS)72 proved efficient for the syntheses illustrated in Scheme 13.22.
Cleavage of the glycosylamino acid allyl ester 81 by Pd(0)-catalyzed allyl transfer to dimedone73 was followed by esterification or, alternatively, amidation of the carboxyl group. Further N-terminal deprotection and condensation steps resulted in the solid-phase-bound derivatives 84. Acid-catalyzed cleavage from the solid support and final hydrogenolysis of the benzyl protecting group resulted in a library of potential sLex mimetics 85.
The few examples outlined in this chapter illustrate the different methods and the efficiency of solid-phase glycopeptide synthesis. Despite these successful examples and those in the literature, the numerous and varying glycosylation patterns occurring in natural glycoproteins demand not only an optimal choice among the existing methods but also the further extension of the tool box for the synthesis of these complex biologically important structures. In other words, the synthesis of glycopeptides still remains a challenging task in organic synthesis.
p-AMBA |
PEG-PS |
|
|
p-AMBA PEG-PS |
|
|
p-AMBA |
PEG-PS |
||||
OO |
|
a, b |
|
OO |
|
|
c, d |
OO |
|
|
|
|
Me O OBn |
|
|
|
|
|
|
|
OBn |
|
|||
|
|
Me |
O OBn |
Me |
O |
|
||||||
O |
|
|
|
|
O |
|
|
|
O O |
|
|
|
FmocHN |
OAll |
|
|
FmocHN |
|
X R |
FmocHN |
|
N |
|
X R |
|
|
|
|
|
|
|
|
1 |
|
|
H |
|
1 |
81 O |
|
|
|
|
|
82 O |
|
R2 |
O |
|
||
|
|
|
|
|
|
|
|
|||||
|
|
|
|
|
|
|
|
|
|
|
83 |
|
X = O, NH |
|
|
|
|
|
|
|
|
|
|
|
|
R1 = Et, |
O |
Me |
|
|
|
|
|
|
|
|
|
|
|
|
|
|
|
|
|
|
|
|
|
||
|
3 |
8 |
|
|
|
|
|
|
|
|
|
e, f |
|
|
O |
|
|
|
|
O |
|
|
|
|
|
|
OH |
|
|
|
HO |
|
|
|
|
|
||
R = |
, R3 |
= |
|
(n = 2,3), |
HO |
P |
|
|
|
|
|
|
2 |
|
HO |
|
|
|
|
|
|
|
|
|
|
OH |
|
|
|
|
|
|
|
|
|
|
|
|
|
|
|
n |
|
|
|
|
|
|
p-AMBA PEG-PS |
||
|
|
HOOH |
|
|
|
|
|
|
||||
|
|
|
|
|
|
O |
|
|
|
|||
|
|
|
|
|
OH |
|
g, h |
|
O |
|
|
|
|
|
Me O |
|
|
|
|
OBn |
|||||
|
|
|
|
Me |
O |
|||||||
|
|
|
|
|
|
|
|
|
|
|
|
|
|
|
|
|
O |
O |
|
|
|
|
O |
O |
|
|
|
|
H |
|
|
|
|
|
|
|||
|
|
|
|
|
|
|
H |
|
|
|||
|
|
|
|
|
|
|
|
|
|
|||
|
|
R3 |
N |
|
|
|
X |
R |
|
|
X |
|
|
|
|
N |
|
N |
|
N |
|||||
|
|
|
|
|
|
R1 |
3 |
|
|
R |
||
|
|
O |
|
R2 |
H |
O |
|
O |
R |
|
H |
1 |
|
|
|
|
|
|
O |
||||||
|
|
|
|
|
|
|
|
|
2 |
|
|
85 |
84 |
a.) Pd(PPh3)4, dimedone; b.) R1OH, 2,6-dichlorbenzoylchloride, pyridine or R1NH2, HBTU, HOBt, NMM; c.) morpholine/DMF; d.) FmocNHCHR2CO2H, HBTU, HOBt, NMM; e.) piperidine/DMF; f.) R3CO2H, HBTU, HOBt, NMM; g.) aqueous AcOH (80% + 2% TFA); h.) H2, 10% Pd/C.
Scheme 13.22 Parallel synthesis of fucopeptide library.
278 SOLID-PHASE SYNTHESIS OF BIOLOGICALLY IMPORTANT GLYCOPEPTIDES
ABBREVIATIONS
p-AMBA—p-(acyloxymethyl)benzylideneacetal AMPS—aminomethyl polystyrene Anth—anthracene
Boc—tert-butyloxycarbonyl DCC—dicyclohexylcarbodiimide Dhbt-OH—3-hydroxy-4-oxo-3,4-dihydro-1,2,3-benzotriazine DIC—diisopropylcarbodiimide; DIEA—diisopropylethylamine DMAP—4-dimethylaminopyridine DMBA—1,3-dimethylbarbituric acid DMDO—2,2-dimethyldioxirane
E-CAD1—epithelial Cadherin 1 HATU—O-(7-azabenzotriazol-1-yl)-N,N,N′,N′-tetramethyluronium hexafluorophosphate
HMBA—hydroxymethylbenzoic acid HOAt—1-hydroxy-7-azabenzotriazole HOBt—1-hydroxybenzotriazole HYCRON—(E)-17-hydroxy-4,7,10,13-tetraoxy-15-heptadecenoyl ICAM-1—intercellular adhesion molecule 1
LFA-1—leukocyte function associated antigen 1 MAG—multiple-antigen glycopeptide PEG-PS—polyethylenglycol polystyrene
PfPyU—N,N-N′,N′-bis(tetramethylene)-O-pentafluorophenyluronium hexafluorophosphat
Pmc—2,2,5,7,8-pentamethyl-chromane-6-sulfonyl SLea/x—sialyl Lewis A/X
TBTU— O-(1-benzotriazole-1-yl)-N,N,N′,N′-tetramethylammonium tetrafluoroborate TIS—triisopropylsilane
TMSOTf—trimethylsilyl trifluoromethanesulfonate Troc—trichloroethoxycarbonyl
Trt—trityl
REFERENCES
1.Varki, A., Glycobiology 3, 97–130 (1993).
2.Lis, H., and Sharon, N., Eur. J. Biochem. 218, 1–27 (1993).
3.Dwek, R. A., Chem. Rev. 96, 683–720 (1996).
4.(a) Kunz, H., and Schultz, M., in Glycopeptides and Related Compounds, Large, D. G., and Warren, C. D., (Eds.), Marcel Dekker, New York, 1997, p. 23; (b) Kunz, H., Pure Appl. Chem. 65, 1223–1232 (1993).
5.For a review, see Arsequell, G., and Valencia, G., Tetrahedron Asym. 8, 2839–2876 (1997).
REFERENCES 279
6.(a) Bhavanandan, V. P., Buddeke, E., Carubelli, P., and Gottschalk, A., Biochem. Biophys. Res. Commun. 16, 353 (1964); (b) Anderson, B., Seno, N., Sampson, P., Riley,
J.G., Hoffman, P., and Meyer, K., J. Biol. Chem. 239, 2716 (1964).
7.Roussel, P., and Lamblin, G., in Glycoproteins and Disease, Montreuil, J., Vliegenthart,
J.F. G., and Schachter, H. (Eds.), Elsevier, Amsterdam 1996, 351.
8.Carlstedt, I., and Davies, J. R., Biochem. Soc. Trans. 25, 214–219 (1997).
9.(a) Schultheiss-Reimann, P., and Kunz, H., Angew. Chem., Int. Ed. Engl. 22, 6263 (1983); (b) Kunz, H., and Birnbach, S., Angew. Chem., Int. Ed. Engl. 25, 360–362 (1986).
10.Lüning, B., Norberg, T., and Tejbrant, J., Glycoconj. J. 6, 5–19 (1989).
11.Meldal, M., and Jensen, K. J., J. Chem. Soc. Chem. Commun. 6, 483–485 (1990).
12.Mathieux, N., Paulsen, H., Meldal, M., and Bock, K., J. Chem. Soc. Perkin Trans. 1 16, 2359–2368 (1997).
13.Schmidt, R. R., and Kinzy, W., Adv. Carbohydr. Chem. Biochem. 50, 21–123 (1994).
14.Boullanger, P., Jouineau, M., Bouammali, B., Lafont, D., and Descotes, G.,Carbohydr. Res. 202, 151–164 (1990).
15.Paulsen, H., Schleyer, A., Mathieux, N., Meldal, M., and Bock, K.,J. Chem. Soc. Perkin Trans. 1 281–293 (1997).
16.Peters, S., Bielfeldt, T., Meldal, M., Bock, K., and Paulsen, H., J. Chem. Soc. Perkin Trans. 1 1163 (1992).
17.Wang, S.-S., J. Am. Chem. Soc. 95, 1328 (1973).
18.Knorr, R., Trzeciak, A., Bannwarth, W., and Gillessen, D., Tetrahedron Lett. 30, 1927 (1989).
19.Atherton, E., Holder, J. L., Meldal, M., Sheppard, R. C., and Valerio, R. M., J. Chem. Soc. Perkin Trans. 1 2887 (1998).
20.(a) Springer, G. F., Science 250, 1198 (1984); (b) Itzkowitz, S. H., Yuan, M., Montgomery, C. K., Kjeldsen, T., Takahashi, H. K., Bigbee, W. L., and Kim, Y. S., Cancer Res. 49, 197 (1989); (c) Hull, S. R., Bright, A., Carraway, K. L., Abe, M., Hayes,
D.F., and Kufe, D. W., Cancer Commun. 1, 261 (1989); (d) Brockhausen, I., Yang, J. M., Burchell, J., Whitehouse, C., and Taylor-Papadimitriou, J., Eur. J. Biochem. 233, 607 (1995); (e) Fukuda, M., Cancer Res. 56, 2237 (1996).
21.Brockhausen, I. in Glycoproteins, Montreuil, J., Schachter, H., and Vliegenthart, J. F. G., (Eds.), Elsevier, Amsterdam, 1995, 201.
22.Bay, S., Lo-Man, R., Osinaga, E., Nakada, H., Leclerc, C., and Cantacuzène, D., J. Peptide Res. 49, 620 (1997).
23.(a) Posnett, D. N., McGrath, H., and Tam, J. P., J. Biol. Chem. 263, 1719 (1988); (b) Tam, J. P., Proc. Natl. Acad. Sci. (USA) 85, 5409 (1988).
24.Leclerc, C., Deriaud, D., Mimic, V., and Van der Werf, S., J. Virol. 65, 711 (1991).
25.(a) Lemieux, R. U., and Ratcliffe, R. M., Can. J. Chem. 57, 1244 (1979); (b) Ferrari, B., and Pavia, A. A., Carbohydr. Res. 79, C1–C7 (1980).
26.Paulsen, H., and Adermann, K., Liebigs Ann. Chem. 751 (1989); Paulsen, H., and Hölck, J.-P., Carbohydr. Res. 109, 89 (1982).
27.(a) Habermann, J., and Kunz, H., Tetrahedron Lett. 39, 265 (1998); (b) Habermann, J., and Kunz, H., J. Prakt. Chem. 340, 233 (1998).
28.Takeichi, M., Science 251, 1451 (1991).