

8. Thermochemistry of amines, nitroso, nitro compounds and related species 367
TABLE 7. |
Enthalpy of formation of nitrotoluenes (kJ mol 1) |
|
||
# |
Solid |
Liquid |
Gaseous |
Reference |
|
|
|
|
|
0 |
5.8 |
12.4 š 0.6 |
50.4 š 0.6 |
|
1 |
|
9.7 š 3.7 |
|
|
(o-) |
|
|
|
|
(m-) |
48.1 š 2.9 |
31.5 š 3.2 |
31.0 š 3.8 |
|
(p-) |
31.2 |
|
||
2 |
15.9 |
|
|
|
(2,3-) |
|
33.2 š 3.2 |
84 |
|
(2,4-) |
66.4 š 3.0 |
|
|
|
(2,4-) |
44.0 |
|
|
84 |
(2,5-) |
34.3 |
|
|
84 |
(2,6-) |
55.2 š 2.2 |
|
|
|
(2,6-) |
37.7 |
|
|
84 |
(3,4-) |
14.6 |
|
|
84 |
(3,5-) |
43.5 |
|
|
84 |
3 |
65.5 š 3.0 |
43.6 š 3.2 |
43.4 š 6.4 |
|
(2,4,6-) |
|
|||
(2,4,6-) |
35 |
|
|
84 |
(2,3,4-) |
15.1 |
|
|
84 |
(2,3,5-) |
23.0 |
|
|
84 |
(2,3,6-) |
16.7 |
|
|
84 |
(2,4,5-) |
15.5 |
|
|
84 |
(3,4,5-) |
5.0 |
|
|
84 |
TABLE 8. Calculated destabilization enthalpies of polynitrotoluenes (kJ mol 1)
|
|
|
Additivity enthalpy |
|
|
Calculated destabilization |
||
# |
|
solid |
liquid |
gaseous |
|
solid |
liquid |
gaseous |
|
|
|
|
|
|
|
|
|
1 |
32.3 |
24.1 |
35.3 |
|
|
14.4 |
|
|
|
(o-) |
|
|
|
|
|
||
|
(m-) |
|
|
|
|
7.4 |
|
|
|
(p-) |
|
|
15.8 |
7.1 |
4.3 |
||
2 |
70.4 |
|
20.2 |
54.5 |
|
|
||
|
(2,3-) |
|
|
|
|
13.0 |
||
|
(2,4-) |
|
|
|
4.0 |
|
||
|
(2,4-) |
|
|
|
26.4 |
|
|
|
|
(2,5-) |
|
|
|
36.1 |
|
|
|
|
(2,6-) |
|
|
|
19.3 |
|
|
|
|
(2,6-) |
|
|
|
32.7 |
|
|
|
|
(3,4-) |
|
|
|
55.8 |
|
|
|
|
(3,5-) |
|
97.1 |
|
26.9 |
|
|
|
3 |
108.5 |
5.1 |
43 |
53.5 |
38.3 |
|||
|
(2,4,6-) |
|
|
|
||||
|
(2,4,6-) |
|
|
|
73.5 |
|
|
|
|
(2,3,4-) |
|
|
|
123.6 |
|
|
|
|
(2,3,5-) |
|
|
|
85.5 |
|
|
|
|
(2,3,6-) |
|
|
|
91.8 |
|
|
|
|
(2,4,5-) |
|
|
|
93.0 |
|
|
|
|
(3,4,5-) |
|
|
|
103.5 |
|
|

368 Joel F. Liebman, Mary Stinecipher Campbell and Suzanne W. Slayden
There are no measured enthalpy-of-formation data for all three mononitrated toluenes in the same phase. However, combining the enthalpy of formation of solid 4-nitrotoluene with its fusion enthalpy results in an enthalpy of formation of the liquid of 31.3 kJ mol 1, a result within experimental error of the liquid 3-isomer. These species are stabilized by 7 kJ mol 1 by the DSE definition. In addition, liquid 2-nitrotoluene is ca 21 kJ mol 1 less stable than its isomers. Destabilization of the ortho isomer could be due to steric effects. Inductive electron-donation may stabilize the meta and para isomers. The solid 2,4- and 2,6-dinitrotoluenes each have two reported enthalpies of formation. The order of stability of the two isomers are contradictory, depending upon the source. The other isomers
exhibit decreasing stability in the order: 3,5- > 2,5- > 2,3- ¾ 3,4-. Again, depending
D
on the source of data, the 2,4- and 2,6-isomers are either more stable than the other isomers or they are of comparable stability to the 3,5- and 2,5-isomers. If the former, the overall stability order is very similar to the corresponding dinitroanilines. Relative to their calculated additivity values, the dinitrotoluenes are more destabilized than their dinitroaniline counterparts. No intramolecular hydrogen bonding can occur to explain the stabilities of the species. Thus, the comparable instability of the 2,3- and 3,4-isomers suggests that any important steric interaction is between the nitro groups. The reversal of stability order for the 2,5- and 3,5-dinitro substituted isomers in changing from the aniline parent to the toluene parent may be due to the lack of intramolecular hydrogen bonding in the 2,5-dinitrotoluene and the presumed greater lattice energy of the symmetrical 3,5-dinitrotoluene.
The trinitrotoluene isomers follow the same trends, invoking dinitro steric interactions and favorable solid lattice energies, as the dinitrotoluenes.
VIII. NITRO COMPOUNDS AS EXPLOSIVES
A. Definitions
An explosive is a chemical compound which has both fuel (H and/or C) and oxidizer (here O on a nitro group) that reacts producing a sudden large change in volume accompanied by rapid release of energy. The high N2 bond strength and the fact that elemental nitrogen is a gas both contribute to the near-ubiquity of nitrogen in explosives. If enough of the explosive compound is compressed such that a chemical reaction can occur before it physically fragments, and a shock wave is formed inside the sample, the resulting reaction is called a detonation. If there is no shock wave, the reaction is called a deflagration. The major difference in the two reactions is the velocity of propagation of the reaction front. The deflagration has a velocity of the order of cm-s 1 instead of km-s 1 a difference of five orders of magnitude.
The maximum reaction enthalpy that can be produced by either process is the same because no additional oxygen is added, but experimentally the products are different because the temperature at which the products are formed is different. In detonation, this temperature is known as the freeze-out temperature and is between 1500 and 1700 K. In deflagration the temperature can be much higher and depends on the enthalpy produced. In detonation or deflagration, the maximum enthalpy of the reaction of the explosive is found from the difference between the enthalpies of formation of its most stable products and that of the compound itself, without the addition of oxygen or other elements. The determination of enthalpy of formation of the explosive is important for determining if there is enough enthalpy to drive the detonation. Explosives with higher enthalpy of detonation are more powerful and usually need less confinement to sustain a detonation. The most stable products of an explosive and their energetic priority for formation from a compound with elemental composition of C,H,N and O (most explosives contain only

8. Thermochemistry of amines, nitroso, nitro compounds and related species 369
TABLE 9. Experimental and calculated major detonation products formed at 1500 K (moles of product formed per mole TNT)
|
Maximum enthalpy |
Experimental |
Calculated |
Product |
mole product |
mole producta |
mole producta |
H2O |
2.5 |
1.60 |
1.06 |
CO2 |
1.75 |
1.25 |
2.19 |
CO |
0 |
1.98 |
0.56 |
C(s) |
5.25 |
3.65 |
3.57 |
N2 |
1.5 |
1.32 |
1.49 |
a See Reference 95.
these elements) are H2O, CO2, C(s) and N2. For example, the detonation equation producing the products with maximum enthalpy of detonation from the well-known explosive TNT (61) is:
C7H5N3O6 ! 2.5H2O C 1.75CO2 C 5.25C(s) C 1.5N2 |
62 |
||||
This reaction has a calculated Hdet of |
|
5.90 kJ g 1 ( 1340 kJ mol 1). The |
mea- |
||
sured experimental value99 was |
|
|
|
|
|
|
4.56 kJ g 1 ( 1036 kJ mol 1). Experimental values |
||||
|
|
|
|
|
are always less negative than the calculated maximum enthalpy because the products formed are not necessarily the most stable ones at standard temperature and pressure. The analyzed products and a composition that was calculated95 at a freeze-out temperature of 1500 K using the Tiger (thermodynamic equilibrium and explosive performance, detonation velocity and pressure) computer code96 are compared in Table 9. The most significant difference between yield of experimental and calculated products is in the carbon-containing products. The actual products include considerable carbon monoxide, the formation of which does not release as much enthalpy as does the formation of carbon dioxide97. The amount of water formed, with its relatively large negative enthalpy of formation, is also less experimentally than calculated. In the idealized detonation equation, 5.75 moles of gas are formed from a mole of solid TNT: the real detonation process produces 6.15 moles of gas. Other explosives that have less excess carbon (that is, more oxygen) produce relatively more moles of gas.
B. Experimental Determination of the Enthalpy of Formation of Explosives
The enthalpy of formation of an explosive compound is found in the same manner as any other compound except sensitive explosives are first tested with a very small sample or combined with standard benzoic acid for burning. Reviewing the protocol, a special Parr bomb98 is used that is built to withstand the high pressures possible on burning. The bomb is pressurized with 30 atm of oxygen and the explosive pellet is ignited with a hot wire to give the standard combustion products H2O, CO2, N2 and HNO3. The amount of nitric acid is found by titration and the enthalpy is corrected to the standard combustion products. The enthalpy at constant volume is corrected to enthalpy of combustion at constant pressure. From this latter enthalpy of combustion, the enthalpy of formation is found from the combustion reaction for the compound. Some high nitrogen content explosives leave carbonand nitrogen-containing residues which are not standard combustion products with well-defined stoichiometry and enthalpy. Such explosives are burned with a known amount of standard benzoic acid to complete combustion to the standard products. Table 10 shows the enthalpy of formation of some high-nitrogen heterocycles that have required this additional benzoic acid. The high nitrogen content compounds and salts
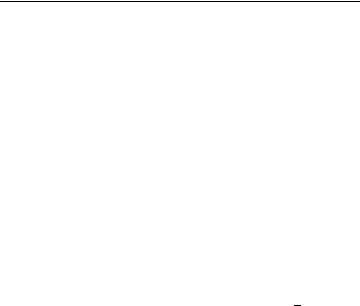
370 Joel F. Liebman, Mary Stinecipher Campbell and Suzanne W. Slayden
TABLE 10. Enthalpy of formation of some |
heterocyclic nitro |
compounds |
|
(kJ mol 1) |
|
|
|
Compound |
Hf(s) |
|
Reference |
|
|
|
|
3-Nitro-1,2,4-triazol-5-one (NTO) |
129.4 š 1.1 |
98 |
|
Ethylenediammonium salt of NTO (ENTO) |
467.8 š 5.9 |
99 |
|
triazole (ANTA) |
. |
. |
|
3-Amino-5-nitro-1,2,4- a |
91 2 š |
7 5 |
100 |
3,6-Bis(ANTA)tetrazine |
731.8 š 4.6 |
101 |
|
Ammonium salt of 5-nitrotetrazole (ANT) |
19.7 š 0.8 |
102 |
|
Ethylenediammonium salt of 5-nitrotetrazole |
233 š 12 |
102 |
a This compound is more properly named 3,6-bis[3-amino-5-nitro-1H-1,2,4-triazol-1-y1]- 1,2,4,5-tetrazine.
with positive enthalpies of formation result in more nitrogen gas (with its definitionally zero enthalpy of formation) formed in their combustion products than is formed from compounds richer in carbon that burn to form carbon dioxide (with its large negative enthalpy of formation). What must be remembered for explosive performance is there must be enough enthalpy of detonation to drive the detonation, but not so much that the compound is sensitive to shock and other accidental stimuli.
C. Sensitivity of Explosives
The desirable sensitivity of an explosive is dependent on its use. Of course, it should not be so sensitive that it cannot be handled or stored for a few years without decomposition. Small amounts of a sensitive explosive are used to start the reaction in the detonator. With the modern initiation systems94 of flying plates or exploding bridge wires, even these detonator explosives can be less sensitive than those used in the past. Another use is as the booster explosive which requires moderate sensitivity. The largest use of explosives is in the main charge, which does the ‘work’. The least sensitive explosive that will do the work is used to prevent accidents during handling.
The sensitivity of an explosive is tested by its reaction to heat and shock. In the three families of nitrated aromatics discussed earlier (cf Sections VII.A D), the more highly nitrated compounds are explosives which have been tested for their sensitivity to impact. Many methods for testing sensitivity have been used. The figure of insensitiveness (FI, commonly used in numerous older studies) measured103 the proportion of total gas a compound produced on various levels of impact and compared this number with that of picric acid, which was arbitrarily given a value of 100. Table 11 shows the enthalpy of formation and FI for the isomers of trinitrotoluene, polynitroanilines and trinitrobenzene. The thermal and shock stabilities of the aromatic polynitro compounds are very good as long as the nitro groups are not in adjacent positions on the ring. Table 11 seems to show a relation between the destabilization enthalpy and one method of characterizing sensitivity.
More recently, explosives have been tested for impact sensitivity by an impact machine in which 40 mg of explosive on sandpaper are placed between an anvil and a steel cylinder. A 2.5 kg weight is dropped from different heights and the sound produced serves to indicate a ‘go’ or ‘no go’. The result from 25 ‘drops’ is calculated to give a height at which the probability of explosion is 50%. Results from a compilation104 are presented in Table 12 and show that the relative values of TNT and picric acid are reversed from the previous table. TNT is relatively more sensitive on the ‘Type 12 impact machine’ than in the FI impact test. TATB is so insensitive that it fails to explode at the maximum drop height of the machine.
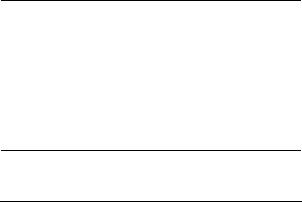
8. Thermochemistry of amines, nitroso, nitro compounds and related species 371
TABLE 11. Figure of insensitiveness (FI) compared to enthalpy of destabilization (DSE, kJ mol 1)
Compound |
DSE |
FIa |
Reference |
1,2,4-Trinitrobenzene |
56 |
103 |
84 |
1,3,5-Trinitrobenzene |
38 |
109 |
94 |
2,4,6-Trinitroaniline |
C22 |
111 |
94 |
2,3,4,6-Tetranitroaniline |
43 |
86 |
94 |
Pentanitroaniline |
339 |
36 |
94 |
2,3,4-Trinitrotoluene |
124 |
92 |
84 |
2,3,5-Trinitrotoluene |
86 |
101 |
84 |
2,4,6-Trinitrotoluene (TNT) |
74 |
114 |
84 |
3,4,5-Trinitrotoluene |
104 |
95 |
84 |
3,4,6-Trinitrotoluene |
93 |
102 |
84 |
a FI (picric acid) D 100 by definition.
TABLE 12. Impact and thermal sensitivity of explosives
|
Impact sensitivity |
Temperature |
|
Type 12 machine |
of onset of |
Compound |
Drop height (cm) |
exotherm (°C) |
HNB (55) |
15 |
165 |
TNT (61) |
148 |
250 |
Picric acid (60) |
191 |
260 |
DATB (56) |
320 |
300 |
TATB (57) |
>320 |
340 |
|
|
|
When heated, an explosive will begin to decompose exothermically. If a large enough sample is heated above its critical temperature, it will explode. The critical temperature is dependent on the shape and size of the explosive sample, its thermal conductivity and its kinetics of decomposition105. A small-scale test for comparison of the thermal stability of explosives is differential thermal analysis (DTA). A few milligrams of sample are placed in a capillary tube and separate thermocouples are placed in the sample and in an accompanying inert sample. The temperature is raised by 10 deg min 1 as the differential temperature of the thermocouples is monitored. As the explosive begins to exotherm, it is monitored on a plot of temperature vs differential temperature. Table 12 shows the thermal stability of some nitroaromatics103.
IX. REFERENCES
1. See, for example, S. W. Slayden |
and J. F. Liebman, in Supplement E2: |
Chemistry of |
Hydroxyl, Ether and Peroxide Groups |
(Ed. S. Patai), Wiley, Chichester, 1993; |
J. F. Liebman, |
K.S. K. Crawford and S. W. Slayden, in Supplement S: The Chemistry of Sulphur-containing Functional Groups (Eds. S. Patai and Z. Rappoport), Wiley, Chichester, 1993; S. W. Slayden,
J.F. Liebman and G. W. Mallard, in Supplement D: The Chemistry of Halides, Pseudo-halides and Azides, Vol. 2 (Eds. S. Patai and Z. Rappoport), Wiley, Chichester, 1995.
2.The primary source of information for enthalpies of formation is J. B. Pedley, R. D. Naylor and
S.P. Kirby, Thermochemical Data of Organic Compounds (2nd Ed.), Chapman & Hall, New York, 1986. The successor to this volume, J. B. Pedley, Thermochemical Data and Structures of Organic Compounds, Vol. I, Thermodynamics Research Center, College Station, Texas, USA, 1994, has recently been published. We have used values from this latter source when the compounds were not included in the earlier edition. In any case, no reference citation is given except for occasionally referring to either volume as ‘Pedley’ or merely ‘archival’. Where data were

372 Joel F. Liebman, Mary Stinecipher Campbell and Suzanne W. Slayden
obtained from some other source, that reference is explicitly given. Some of our other preferred secondary sources include:
(a)M. S. Kharasch, Bur. Stand. J. Res., 2, 359 (1929).
(b)G. R. Handrick, Ind. Eng. Chem., 48, 1366 (1956) and the earlier Report on Study of Pure Explosive Compounds by G. R. Handrick and W. C. Lothrop, a document from Arthur D. Little Inc. for the Office of the Chief of Ordnance, Contract No. DA-19-020-ORD-47 C-58247, dated May 1, 1952. We heartily thank Dr. Handrick for making this study available to the authors.
(c)D. R. Stull, E. F. Westrum, Jr. and G. C. Sinke, The Chemical Thermodynamics of Organic Compounds, Wiley, New York, 1969.
(d)E. S. Domalski, J. Phys. Chem. Ref. Data, 1, 221 (1972).
(e)E. S. Domalski and E. D. Hearing, J. Phys. Chem. Ref. Data, 22, 805 (1993).
These sources will usually be referred to as ‘Kharasch’, ‘Handrick’, ‘Stull, Westrum and Sinke’; ‘Domalski’ and ‘Domalski and Hearing’.
3.For the reader interested in these data, we cite the compendia, E. S. Domalski, W. H. Evans and E. D. Hearing, ‘Heat Capacities and Entropies of Organic Compounds in the Condensed Phase’, J. Phys. Chem. Ref. Data, 13, Suppl. 1 (1984), and its supplement E. S. Domalski and
E.D. Hearing, J. Phys. Chem. Ref. Data, 19, 881 (1990).
4.Unless indicated to the contrary, all estimated enthalpies of vaporization use the approach in
J.S. Chickos, D. G. Hesse, J. F. Liebman and S. Y. Panshin, J. Org. Chem., 53, 3435 (1988).
5.Unless indicated to the contrary, all measured enthalpies of fusion are taken from the compendia by Domalski and coworkers3 and by W. E. Acree, Jr., Thermochim. Acta, 189, 37 (1991); 219, 97 (1993) and a still unpublished supplement (personal communication). We thank Dr William Acree for providing us with his unpublished data.
6.J. S. Chickos, in Molecular Structure and Energetics: Physical Measurements (Eds. J. F. Liebman and A. Greenberg), VCH, New York, 1987 and unpublished supplement (personal communication). We thank Dr James Chickos for providing us with his unpublished study.
7.J. F. Liebman and H. M. Perks, in The Chemistry of Enamines (Ed. Z. Rappoport), Wiley, Chichester, 1994.
8.L. Batt and G. N. Robinson, in Supplement F. Chemistry of Amino, Nitroso and Nitro Compounds and their Derivatives (Ed. S. Patai), Wiley, Chichester, 1982.
9.E. J. Prosen, W. H. Johnson and F. D. Rossini, J. Res. Natl. Bur. Stand., 37, 51 (1946).
10.J. D. Cox and G. Pilcher, Thermochemistry of Organic and Organometallic Compounds, Academic Press, New York, 1970.
11.P. Sellers, G. Stridh and S. Sunner, J. Chem. Eng. Data, 23, 250 (1978).
12.The original statement for the relationship in equation 1 was given in Reference 9 as
H°f [Y (CH2)m H(g)] D A0 C Bm C
where ‘ is a term which has a small finite value for the lower members, being largest for m D 0, and becomes zero for the higher members beginning near m D 4’. In that study, Y was CH3 as well as other carbon-containing substituent groups. Subsequent analysis in Reference 10 showed that only the first part of the quoted phrase is true. The values do not necessarily decrease and the enthalpy-of-formation deviation for an ethyl-substituted compound may sometimes be smaller than that for butyl.
13.R. L. Montgomery and F. D. Rossini, J. Chem. Thermodyn., 10, 471 (1978).
14.J. F. Liebman, J. A. Martinho Simoes˜ and S. W. Slayden, Struct. Chem., 6, 65 (1995).
15.J. F. Liebman, J. A. Martinho Simoes˜ and S. W. Slayden, in Lithium Chemistry: A Theoretical and Experimental Overview (Eds. A. -M. Sapse and P. von R. Schleyer), Wiley, New York, 1995.
16.By contrast, we do not use ‘Benson group increments’, a generally powerful thermochemical technique summarized in the volume by S. W. Benson himself, Thermochemical Kinetics, 2nd edition, Wiley, New York, 1976, and used in many thermochemical chapters throughout the Patai series. For the classes of compounds discussed in the current chapter, we believe the necessary number of parameters (included to reflect electrostatic interactions, proximity effects, steric repulsions and ring corrections) is excessive.
17.The group additivity values were taken from ‘Revised Group Additivity Values of Enthalpies of Formation (at 298 K) of Carbon Hydrogen Compounds’, by N. Cohen, prepared for National Institute of Standards and Technology, 1994 as Aerospace Report No. ATR-94(7263)-1. The
8. Thermochemistry of amines, nitroso, nitro compounds and related species 373
revision of group additivity values to predict thermochemical properties by means of Benson’s group additivity method (Reference 16) is based on the experimental enthalpies of formation found in the compendium by Pedley. We thank Dr Norman Cohen for giving us a copy of his report.
18.For example, see A. Greenberg and T. A. Stevenson, in Molecular Structure and Energetics: Studies of Organic Molecules (Vol. 3) (Ed. J. F. Liebman and A. Greenberg), VCH, Deerfield Beach, 1986.
19.This was derived from the difference of the gas-phase enthalpies of formation of the archival
cycloheptane and hexahydroazepine, the latter quantity of 45.2 š 1.9 kJ mol 1 reported in
X. -W. An, Z. -L. Zhang, S. Wang, J. Yan, R. Hu and J. Hu, Huaxue Xuebao, 39, 485 (1980).
20.W. V. Steele, R. D. Chirico, A. Nguyen and S. E. Knipmeyer, J. Chem. Thermodyn., 26, 515 (1994).
21.James S. Chickos, personal communication to the authors.
22.If the semiempirical equations proposed in Reference 4 are correct, then the exothermicity of the reaction RXC R1Y ! RYC R1X would be identical in the gaseous and liquid phases. Experience shows the assumption generally results in much poorer agreement for all-solid reactions. No such semiempirical equations for enthalpy of sublimation and its estimation appear to be available, although some cancellation is expected.
23.From Reference 4, we derive that 4.7 kJ mol 1 is the estimated, and assumed, universal contribution of one carbon to enthalpies of vaporization for all monosubstituted hydrocarbons and 8.9 is the parameter for tertiary amines.
24.Strictly speaking, we should consider nicotine a bifunctional species and so use the analysis in J. S. Chickos, D. G. Hesse and J. F. Liebman, J. Org. Chem., 54, 5250 (1989) to estimate enthalpy of vaporization. However, in that the enthalpy of combustion data are old and the two substituents (tertiary amine and pyridine) are rather far apart and not involved in hydrogen bonding, we continue with our simplified analysis.
25.M. P. Kozina, L. P. Timofeeva, G. L. Gal’chenko, I. M. Skvortsov and I. V. Antipova, J. Gen. Chem. USSR, 51, 367 (1981).
26.υ4(prim/R) differs by 6 for R that is prim and sec and υ5sec/R,R1) differs by 15 for R and R1 both prim and both sec. Extrapolating to υ6(tert/R,R1,R2) where the Rs are all primary vs all secondary, the new difference quantity should be ca 70 kJ mol 1. Using the assumed
thermoneutrality of equation 8 as a guide, the difference quantity should be ca 66 kJ mol 1. 27. For example, consider the near-planar nitrogen in 1-azabicyclo[3.3.3]undecane (manxine),
N. J. Leonard, J. C. Coll, A. H. -J. Wang, R. J. Missavage and I. C. Paul, J. Amer. Chem. Soc., 93, 4639 (1971). Regrettably, we know of no enthalpy of formation measurements on either this heterocycle or the corresponding hydrocarbon, manxane.
28.See, for example, S. Bohm,¨ M. Decouzon, O. Exner, J. -F. Gal and P. -C. Maria, J. Org. Chem., 59, 8127 (1994).
29.This appears plausible to us having earlier done so for understanding the energetics of aryl halides, cf S. W. Slayden, J. F. Liebman and W. G. Mallard, in Supplement D2: The Chemistry of Organic Halides, Pseudohalides and Azides (Eds. S. Patai and Z. Rappoport), Wiley, Chichester, 1995: i-Pr and Ph have the substituent-bearing carbon bonded to two other carbons and t-Bu and Ph have the substituent-bearing carbon bonded just to carbon and not bonded to hydrogen or any additional univalent group.
30. |
(a) Both W. E. Garner and C. L. Abernathy, Proc. Roy. Soc. London, 99A, 213 (1921) and |
|
G. N. Vriens and A. C. Hill, Ind. Eng. Chem., 44, 2732 (1952) report nearly identical enthalpies |
|
of combustion of 16 of ca 974 kJ mol 1 from which an enthalpy of formation of the liquid of |
|
ca 32 kJ mol 1 is found. The latter authors also give Hv 16 D 53 kJ mol 1 and so the desired |
|
gas-phase enthalpy of formation is ca 85 kJ mol 1. |
|
(b) J. P. Guthrie, J. Barker, P. A. Cullimore, J. Lu and D. C. Pike, Can. J. Chem., 71, 2109 |
|
(1993) consider the above data as part of a reaction calorimetry study and suggest Hf(g, 38) D |
|
84 kJ mol 1. |
31. |
O. I. Kachurin, N. M. Matvienko and V. G. Chekhuta, Ukr. Khim. Zh., 45, 139 (1979) and |
|
N. M. Matvienko, O. I. Kachurin and V. G. Chekhuta, Ukr. Khim. Zh., 48, 1046 (1982) report |
a high-temperature acid-catalyzed transmethylation 2 16 14 C 15 with an accompanying reaction enthalpy of ca 4 kJ mol 1. From these data, we derive Hf(g, 16) D 96 kJ mol 1.
374Joel F. Liebman, Mary Stinecipher Campbell and Suzanne W. Slayden
32.The enthalpies of formation for the naphthols are from Pedley; for the methylnaphthalenes, from R. Sabbah, R. Chastel and M. Laffitte, Thermochim. Acta, 10, 353 (1974); and for the bromonaphthalenes, from M. A. V. Ribeiro da Silva, M. L. C. C. H. Ferrao and A. J. M. Lopes,
J.Chem. Thermodyn., 25, 229 (1993).
33.See Ribeiro da Silva and coworkers in Reference 32.
34.Our success is at a price. After all, we have three occasionally conflicting prejudices with regard to choice of data. The first is to use recommended archival values. The second is to use the most recent piece of data. The third is to use an alternative but selected source, e.g. the same reference for two isomers. We have acted according to our last, but weakest, prejudice here. We admit that given the well-established carcinogenicity of ˇ-naphthylamine, there is no way in clear conscience to recommend remeasurement of the quantities of interest.
35.There is a 30 kJ mol 1 difference in the recommended enthalpies of formation of solid 2- aminobiphenyl in the two volumes by Pedley, cf the new measurements reported in W. F. Steele,
R.D. Chirico, S. E. Knipmeyer and A. Nguyen, J. Chem. Thermodyn., 23, 957 (1991). This difference attests to the difficulty of making enthalpy-of-formation measurements on amines, a conclusion already intimated in the discussion of the naphthylamines.
36.The three methylamines have comparable enthalpies of formation (to within 5 kJ mol 1) and the
enthalpy of formation of the parent NH3 is some 20 kJ mol 1 more negative than that of any of the amines.
37. The necessary enthalpy of formation of gaseous diphenylmethane is from W. V. Steele,
R.D. Chirico and N. K. Smith, J. Chem. Thermodyn., 17, 671 (1995).
38.A. J. Kirby, The Anomeric Effect and Related Stereoelectronic Effects at Oxygen, Springer-Verlag, Berlin, 1983; P. Delongchanps, Stereoelectronic Effects in Organic Chemistry, Wiley, New York, 1983.
39.We neglect here any effect of intramolecular hydrogen bonding because we doubt its presence. The necessary NÐ Ð ÐHÐ Ð ÐN angle would be ca 90° instead of the proper 180° found in the geometry that would allow for optimum hydrogen bonding.
40.The corresponding reactions 2EtX ! XCH2CH2X C CH3CH3 of ethyl haldies to form the ethylene dihalide are endothermic by 14 kJ mol 1, endo by 2 and exo by 2 for Cl, Br and I, respectively. This suggests some hydrogen bonding stabilization exists for the diamino case, even if the NÐ Ð ÐHÐ Ð ÐN angle is manifestly non-optimum.
41.The enthalpy of formation of the solid amine is 141.0 š 1.7 kJ mol 1 and is from D. R. Stull, Bull. Chem. Thermodyn., 2, 5 (1958) accompanied by an enthalpy of sublimation of 75.7 kJ mol 1 from Drs Sarah Hosseini and James Chickos, unpublished results.
42.The thermochemical consequences of interactions of acetylenes with amines is unexplored save
the studies of the enthalpies of formation of species 25, HC CCH2NMe2 and the unsubstituted HC CCH2NH2, and (HC CCH2)3 n Prn N, with n D 0, 1 and 2. A probe for the accuracy of their enthalpies of formation is the formal enthalpy of hydrogenation of HC CCH2NH2. The enthalpy of vaporization of this amine is predicted to be essentially the same as its saturated analog. Equivalently, the enthalpy of hydrogenation of the pure liquid should equal that of the gas, and both equal the phase appropriate difference of the enthalpy of formation of the unsaturated and saturated amines. In the absence of any substituent effects, this propargylamine
hydrogenation enthalpy would be the same as that of propyne. Should steric demands of NH2 and Me be equated, then the propargylamine hydrogenation enthalpy will equal that of 1-butyne. For the amine, the enthalpy of hydrogenation equals 307.2š1.0 kJ mol 1. By contrast, for propyne, 1-butyne(g) and 1-butyne(lq), the hydrogenation enthalpies equal 289.6 š 1.0, 290.8 š 1.2 and 288.5 š 1.2 kJ mol 1, respectively. It is hard to imagine how propargylamine differs so much from the parent, all-hydrocarbon, alkynes. This apparent incongruity makes us suspect the enthalpy-of-formation results for all of the acetylenic amines. But disregarding them is a pyrrhic victory because the thermochemical data base on polyamines has been meaningfully decreased.
43.O. P. Kuznetsova, E. A. Miroshnichenko, A. N. Zelenetskii, G. V. Rakova, Y. A. Lebedev and
N.S. Enikolopyan, Proc. Natl. Acad. Sci. U.S.S.R., 226, 147 (1976), citing A. G. Tomilov and
K.S. Smirnov, Adiponitrile and Hexamethylenediamine (in Russian), Khimya, Moscow, 1974.
44.This value illustrates the virtue of not being thermochemical purists. It was obtained by averaging the enthalpy of formation of solid piperazine from Pedley and from An and coworkers (Reference 19) and summing the result with the enthalpy of sublimation from Chickos. In that the last quantity was measured at a mean temperature of 294 K instead of the idealized 298 K, errors are in fact minimal.
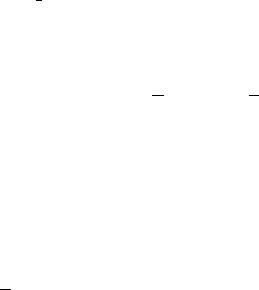
8. Thermochemistry of amines, nitroso, nitro compounds and related species 375
45.The enthalpy of formation of liquid and gaseous morpholine are 185.7 š 2.7 and 142.8 š
3.3 kJ mol 1, respectively: K. Pihlaja and P. Vainiotalo, unpublished results, cited by K. Pihlaja in Molecular Structure and Energetics: Physical Measurements (Eds. J. F. Liebman and A. Greenberg), VCH, New York, 1987. From the same source we find N-methylmorpholine is ca 21 kJ mol 1 less stable than its 1,3-counterpart parallelling the earlier conclusion that vic- diamines are less stable than the related gem-diamines.
46.R. H. Staley and J. L. Beauchamp, J. Am. Chem. Soc., 96, 1604 (1974).
47.On the other hand, this reaction is endothermic by almost 10 kJ mol 1 in the solid state. This is surprising because the monoamine has a nonzero dipole moment and a molecular structure that allows for formation of linear arrays of intermolecularly NÐ Ð ÐH C hydrogen-bonded molecules in the crystal, while the other species have zero dipoles and lack the possibility of such stabilization mechanisms in the solid.
48.We are being somewhat disingenuous here. If performed and interpreted correctly and with the appropriate ancillary phase-change enthalpy information, the enthalpy of formation of an arbitrary species by ion molecule reaction chemistry and by combustion calorimetry must be the same. That the ionization potential of quinuclidine is higher than 1,4-diazabicyclo[2.2.2]octane simply says that there is a stabilizing effect in the radical cation of the latter not found in the former. This information does not say that there is a stabilizing effect in the neutral molecular form of the latter not found in the former. After all, we trust the reader is not bothered by the fact that the ionization potential order of the cyclohexenes increases in the order: 1,3-diene < 1,4-diene < 1-ene < ‘1,3,5-triene’ (benzene).
49.This boat-chair difference is not immediately obtainable from experiment, e.g. the high-
temperature conformational isomerization and then quenching of cyclohexane does not result in a chair ! boat interconversion, but rather chair ! twist-boat; M. Squillacote, R. S. Sheridan, O. L. Chapman and F. A. L. Anet, J. Am. Chem. Soc., 97, 3244 (1975).
50. For the o-isomer, there is a new value of 39.1 š 5.7 kJ mol 1 [I. Contineanu, L. Wagner, L. Stanescu and D. I. Marchidan, Rev. Roum. Chim., 27, 205 (1982)] and for the m-isomer, there is a new value of 33.3 š 7.3 kJ mol 1 [I. B. Rabinovich, N. V. Karyakin, E. S. Dzharimova, S. A. Siling, I. L. Ponomarev and S. V. Vinogradova, Bull. Acad. Sci. USSR, Div. Chem. Sci.,
716 (1984)].
51.In Kharasch we find the enthalpies of formation of solid 2,40 - and 4,40 -diaminobiphenyl from an 1889 paper. The two values differ by ca 4 kJ mol 1 with the latter isomer more stable. Do we accept this ca 4 kJ mol 1 to be the difference of the enthalpies of formation of the two isomers? We now admit that there are two more recent, but still archival, measurements for the 4,40 -isomer
which differ from the earlier one by ca 20 and 24 kJ mol 1. (The new ones were reported in 1908 and 1944.)
52.It is frustrating that this result, and accordingly the enthalpy of formation of 54, is probably in
error. After all, it is often assumed that acetylated amines are easier to purify than the parent amine for example, we recall that samples of acetanilide are generally clean white solids while those of aniline are discolored liquids.
53. The |
original study is B. G. Gowenlock and |
K. E. Thomas, J. Chem. Soc. (B), 409 (1966). |
The |
value we have taken makes use of |
the ‘corrections’ suggested by S. W. Benson, |
F.R. Cruickshank, D. M. Golden, G. R. Haugen, H. E. O’Neal, A. S. Rodgers, R. Shaw and
R.Walsh, Chem. Rev., 69, 279 (1969).
54.R. A. L. Peerboom, S. Ingemann, N. M. M. Nibbering and J. F. Liebman, J. Chem. Soc., Perkin Trans. 2, 2, 1825 (1990).
55.There is a ca 70 kJ mol 1 uncertainty accompanying Hf(CH2NH), cf. B. J. Smith, J. A. Pople,
L.A. Curtiss and L. Radom, Aust. J. Chem., 45, 285 (1992). We will not attempt to resolve this issue in the current chapter.
56.K. B. Wiberg, D. Y. Nakaji and K. M. Morgan, J. Am. Chem. Soc., 115, 3527 (1993).
57.From the approach suggested in J. S. Chickos, D. G. Hesse and J. F. Liebman, J. Org. Chem., 55, 3833 (1990), we conclude that the errors of ignoring multiple substitution and a small multiple of RT will not ameliorate the seemingly disparate enthalpies of formation of the three triamines of interest.
58.R. M. Clay, S. Lorr, G. Keenan and W. V. Steele, J. Am. Chem. Soc., 105, 2070 (1983).
59. J. S. Chickos, D. G. Hesse, S. Y. Panshin, D. W. Rogers, M. Saunders, P. M. Uffer and
J. F. Liebman, J. Org. Chem., 57, 2897 (1992).
60.E. M. Engler, J. D. Andose and P. v. R. Schleyer, J. Am. Chem. Soc., 95, 8005 (1973).

376Joel F. Liebman, Mary Stinecipher Campbell and Suzanne W. Slayden
61.D. Van Vechten and J. F. Liebman, Isr. J. Chem., 21, 105 (1981).
62.It has been asserted that the choice of thermochemical reference states, including those for strain energy considerations, is inherently subjective and so this question cannot be objectively answered: see, J. F. Liebman and D. Van Vechten, in Molecular Structure and Energetics: Physical Measurements (Eds. J. F. Liebman and A. Greenberg), VCH, New York, 1987.
63.To calibrate our thinking, the resonance or extra stabilization found in benzene as opposed to a species with three isolated double bonds is ca 151 kJ mol 1, which we note is fortuitously nearly the identical value as found in the comparison of these nitrogenous heterocycles.
64.As such, a more thorough description of the energetics of nitroso compounds may well logically appear in a future Patai volume on functional groups devoted to carbon-containing double bonds such as monoalkenes, imines and ketones and aldehydes, since oximes would seem to belong with these functionalities.
65.As such, a more thorough description of the energetics of nitroso compounds may well logically appear in a future Patai volume on functional groups devoted to nitrogen nitrogen bonds such as hydrazo, azo and azoxy compounds, since azodioxy species would seem to belong with these functionalities. We additionally note that these azodioxy species can occur in both cis- and trans-form, and the relative stability seems dependent on the affixed hydrocarbyl moiety.
66.We refer to this as a thermochemical measurement when, in fact, the necessary equilibrium measurement techniques are usually found among the arsenal of the kineticist. A minor distinction to the reader perhaps, but it appears to the authors that except for Benson and his descendants, thermochemists and kineticists generally inadequately communicate.
67.W. E. Acree, Jr., S. A. Tucker, G. Pilcher, A. Chowdary. M. D. M. C. Ribeiro da Silva and M. J. S. Monte, J. Chem. Thermodyn., 25, 1252 (1993).
68.From Batt (Reference 8), we find values of 215 š 8, 249 š 13 and 203 š 4 kJ mol 1.
69.The enthalpy of formation of the gaseous tetramethylbenzene was obtained by summing Pedley’s value for the liquid and the enthalpy of vaporization from R. Sabbah, D. Tabet and S. Belaadi,´
Thermochim. Acta, 247, 193 (1994).
70.M. Colomina, C. Turrion,´ P. Jimenez,´ M. V. Roux and J. F. Liebman, Struct. Chem., 5, 141 (1994).
71.W. E. Acree, Jr., S. A. Tucker, G. Pilcher and G. Toole, J. Chem. Thermodyn., 26, 85 (1994).
72.We note now that Handrick cites some studies from the end of the last century and early in this. Admitting that our prejudices suggest using the values from Reference 71 and hence from this decade, it is nonetheless interesting that the most recent and the early values ‘merely’ differ by
ca 30 kJ mol 1. This difference is non-negligible, but is not particularly worse than the spread of values for the parent nitrosobenzene using much more contemporary techniques and analyses.
73.To be most consistent with the selection of the enthalpy of formation of 4- dimethylaminonitrosobenzene, we have decided to use in this section the enthalpy of formation of 4-dimethylaminonitrobenzene from Acree and coworkers in Reference 71; as well,
we acknowledge the merely 3 kJ mol 1 discrepant value found in J. Furukawa, S. Murata,
M. Sakiyami and S. Seki, Bull. Chem. Soc. Jpn., 57, 3058 (1984).
74.Kharasch gives us a nearly 90-year-old enthalpy of formation of the solid amine. However, no enthalpy of sublimation is seemingly available.
75.See R. Sabbah and M. Gouali, Aust. J. Chem., 47, 1651 (1994) and references cited therein.
76. This |
appears quite likely; cf. K. T. Finley and L. K. J. Tong, in The Chemistry of the Car- |
||
bon |
|
Nitrogen Double Bond (Ed. S. Patai), Wiley, London, 1970. Nonetheless, in that the question |
|
|
|||
of species ‘identification’ persists is |
suggestive that the two tautomers are relatively close in |
||
energy and so our analysis continues |
quite unfazed by this seeming ambiguity. |
77.We welcome a study of the tautomerically frozen species, some synthetically and calorimetrically suitable alkoxynitrosobenzenes, their p-benzoquinone O-alkyloxime isomers and the corresponding napthalene analogs.
78.Two recent reviews of hydrocarbon radical enthalpies of formation are J. Berkowitz, G. B. Ellison and D. Gutman, J. Phys. Chem., 98, 1744 (1994) and W. Tsang, in Energetics of Organic Free Radicals [Vol. 4 in the SEARCH Series (Eds. J. A. Martinho Simoes,˜ A. Greenberg and J. F. Liebman), Blackie Academic & Professional, an imprint of Chapman & Hall, London, in press]. The enthalpies of formation of hydrocarbyl radicals is still a subject of some dispute. Admitting our prejudices, the values chosen in the current study are those recommended by the latter reference.