
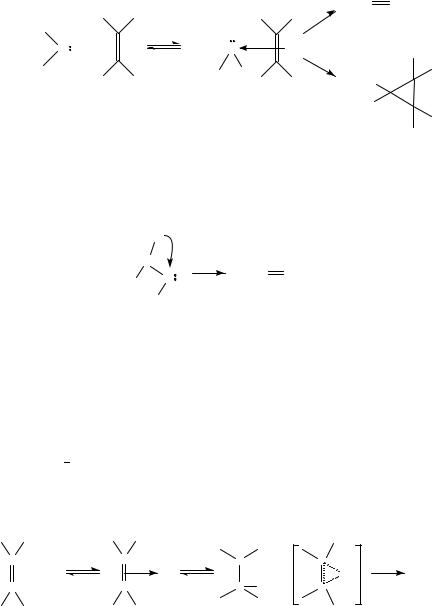
|
8. Complex formation involving double-bonded functional groups |
377 |
|
|
|
PhCH |
CHCl |
PhCH2 |
|
|
|
|
C + |
C |
|
Cl |
PhCH2 |
Cl |
|
|
|
|
PhCH2 |
(25) |
Cl |
|
SCHEME 9
a complex (in a potential energy minimum), or a contact pair in a solvent cage64,65, also depends on the structure of the partners.
Styrene derivatives may be obtained directly from the carbene, by internal hydrogen migration (reaction 3), or from the complex59 (25).
H |
|
|
|
PhC |
|
PhCH CHCl |
(3) |
|
C |
||
H |
|
||
|
|
||
|
|
|
|
Cl |
|
|
|
Theoretical studies65 on cycloaddition of halocarbenes (mainly CF2, CCl2) with ethylene suggest an alternative reaction pathway. This explains the high (negative) entropy value66 without the intervention of the intermediate complex reported by the multi-step mechanism of Scheme 967.
E. Complexes Between Olefins and Halogens: Introduction
Interactions between the CDC double bond and halogens deserve particular attention. The importance of these interactions arises from the fact they are the first step of the reactions of addition of halogens to alkenes (and, in general, of the electrophilic addition to the carbon carbon double bond68), which is a very useful functionalization reaction of the alkene double bond.
When mechanisms involving radicals can be discounted, the main and general reaction pathway of addition of halogens to alkenes is shown in Scheme 10, where X is mainly Cl and Br69,70.
|
C |
+ |
|
|
|
C |
C |
C |
|
|
|
+ X2 |
X2 |
X− |
or |
+ X |
Products |
C |
C |
C X |
C |
X− |
|
|
(26) |
(27) |
(28) |
|
|
|
|
SCHEME 10 |
|
|
|
The nature of the interaction between alkenes and halogens (complex 26 of Scheme 10) preceding the reactions of addition of the halogens to the CDC double bond is labelled according to Mulliken’s classification71 as a CT complex. The nature of the carbonium

378 |
Luciano Forlani |
ions 27 and 28 has been discussed, and has been the subject of several investigations. When X D Br, there are indications that bromination of alkenes includes the cyclic bromonium ion in the reaction pathway.
The first step of the reaction is the formation of a complex between the double bond and the halogen68,72 (26 in Scheme 10). This complex may be considered as a ‘charge transfer’ complex, but there are some doubts about the amount of charge which is transferred. When the amount of the transferred charge is small, the definition ‘van der Waals complex’ is more appropriate73.
The decrease of overall reaction rate as the temperature increases74 confirms the presence of aggregating processes forming complexes (mainly CT complexes) on the reaction pathway of bromination of olefins: the association of solutes is usually favoured by lowering the temperature.
When the olefin used is sterically hindered in relation to the nucleophilic part of the halogenating reagent or toward the solvent (if it is a nucleophilic solvent), the complex 26 of Scheme 10 is in rapid equilibrium with the free reagents and the halonium ion 28 (or the cation 27)75.
F. Complexes Between Olefins and Cl2, Br2, I2
The usual reaction between olefins and bromine or chlorine is illustrated in Scheme 11. Final products are di-brominated compounds 29. Theoretical studies76 agree with Scheme 11. Both Schemes 10 and 11 involve a quickly established equilibrium of formation of a CT complex between olefin and bromine.
C |
|
C |
|
C |
+ |
Br− |
|
|
|
|
C |
|
Br |
||
|
|
|
|
|
|
|
|||||||||
+ Br2 |
|
Br2 |
|
|
Br |
|
|
|
|
|
|
|
|
|
|
|
|
|
|
|
|
|
|
|
|||||||
|
|
C |
|
Br |
|
C |
|
|
|
||||||
C |
|
C |
|
|
|
|
|
|
|
|
|||||
|
|
|
|
|
|
|
|
|
|
|
|
|
|||
|
|
|
|
|
|
|
|
|
|
|
|
|
|
||
|
|
|
|
|
|
|
|
|
|
(29) |
|
|
SCHEME 11
The formation of the complex (26) is followed by the formation of a complex (28, the ‘bromonium ion’), in a rate-limiting step. From 28 the products are obtained in a fast step (which is similar to a neutralization) with nucleophiles. The presence of the CT complex is indicated by unambiguous spectroscopic evidence68,77,78 and also by fast monitoring of reaction mixtures immediately after mixing79,80.
Basically, the formation of the bromonium ion may be considered either a reversible or irreversible process. In spite of some evidence of reversibility of the formation of bromonium ion, in 1990, Ruasse affirmed that, in protic solvents, ‘the ionic intermediate is formed irreversibly’81. Now, there is a body of evidence supporting the reversibility of the bromonium ion formation in bromination of olefins.
In a recent article, Bellucci and coworkers extensively discuss this problem82 and conclude that the kinetic results and the distribution of the obtained products provide strong evidence of a return to a CT complex from ion 28. See Scheme 10.
The reversibility of the ionization of the CT complex occurs not only with very congested alkenes, but may also be considered a general feature of bromine addition to CDC double bonds in both protic and aprotic solvents82.
Bromonium ion formation (reversible or irreversible) may be assisted by the presence of a nucleophilic solvent, as shown in 30 of reaction 4 involving alcohols as solvent.
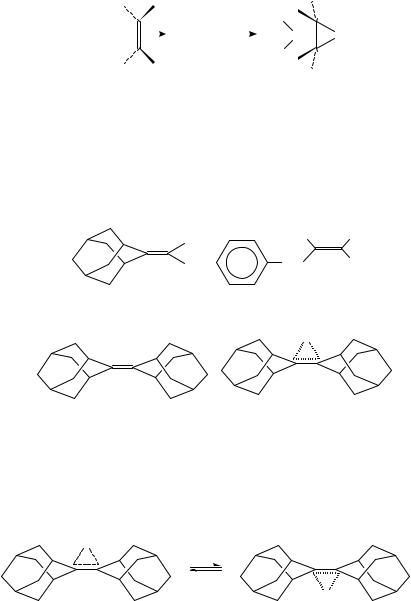
8. Complex formation involving double-bonded functional groups |
379 |
||||||
|
|
|
|
|
|
R |
|
ROH + |
|
Br |
|
Br |
|
O . + Br.Br− |
(4) |
|
|
|
H
(30)
Kinetic investigations83 into the bromination of methylidene adamantane (31) and allylbenzene (32) reveals the importance of the assistance of the solvent to the rate-limiting ionization to form bromonium ion from the CT complex.
The rate of the return from bromonium ion to CT complex depends on the nature of the solvent and of the substituents bonded to olefin81,84. By using adamantylideneadamantane 33, halonium ions 34 are formed as stable (and isolatable) salts85, because of steric hindrance of the nucleophilic counter ion. Consequently, the products of addition, such as the dibromides 29, cannot be obtained.
H H
CH2 H
(31) |
(32) |
X
+
(33) |
(34) X = Cl, Br, I |
In the same way, the thiiranium ion (34, X D S R) has been isolated from a mixture of adamantylideneadamantane and methane sulphenyl chloride86. The stability of 34, X D Br, which is the only known stable bromonium ion, and the fact that 34 cannot form the saturated products of the bromination reactions of olefins enable the investigation87 (by a dynamic 1H-NMR technique) of equilibrium 5, which proceeds via the dissociation of the 1:1 CT complex between 33 and bromine.
X
+
(5)
+
X
The stability constants of some CT complexes between olefins and bromine (equation 6) in 1,2-dichloroethane at 25 °C clearly indicates the influence of the alkyl substituents bonded to the double bonds on the K values: K (mol 1 dm3) D 0.47, 9.7, 290 and 700 for cyclohexene78, tetraisobutylethylene72, adamantylideneadamantane79 33 and dl-D3- trishomocubylidene-D3-trishomocubane88 35.
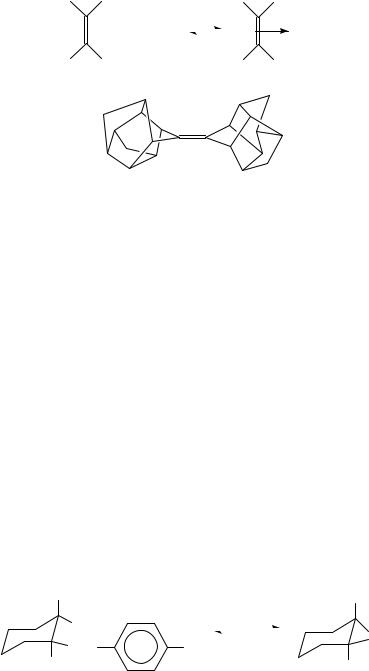
380 |
Luciano Forlani |
|
|
||
+ |
Br2 |
K |
Br2 |
|
|
|
|
(6) |
|||
|
|
||||
|
|
|
|
|
CT complex
(35)
X-ray diffraction of bromonium (X D Br)89 and iodonium (X D I)75 salts confirms the 34 structure.
The bromonium ion tribromide salt of 3390,91 (as well as the triflate salt92) transfers Br2 to other olefins by irreversible reactions forming dibromo derivatives.
Using the UV/VIS spectrophotometric method, the equilibria of Scheme 12 have been investigated93 as a model of the bromonium triand pentabromides, the intermediates of the reactions of olefin bromination.
CBr2 |
|
CBr2 |
|
Bu4NC Br Bu4NC Br3 |
Bu4NC Br5 |
||
|
|
|
|
|
|
|
|
SCHEME 12
In 1,2-dichloroethane, UV/VIS spectroscopic data indicate that in the mixtures of 33 and bromine there are 2:1, 1:1, 1:2 and 1:3 (33:Br2) complexes. Conductivity measurements of the same mixtures indicate that the complex 1:1 is a CT complex whilst the other complexes are ionic in character. The complexes with stoichiometry 1:2 and 1:3 (33:Br2) are bromonium tribromide and pentabromide, respectively, as indicated in Scheme 13. CT complexes with moderate stability were also reported90 between 33 and different charged electrophiles (nitrosonium and nitronium salts)
The bromonium ion may be generated by other methods than direct bromination: solvolytic reaction of trans-2-bromo-[(4-bromophenyl)sulphonyl]cyclohexane (36) (and cyclopentane) forms (reaction 7) the bromonium ion94 (37). If Br is present in the reaction mixture, the generation of Br2 (and olefin) is observed (Scheme 14). This confirms the reversibility of the bromonium ion formation in the usual bromination pathway. When other olefin scavengers are present, a formal BrC transfer is observed94. This may occur without the formation of Br2.
H |
|
|
H |
Br |
|
CH3 COOH |
|
|
|
+ Br (7) |
|
OSO2 |
Br |
CH3 COO− |
|
|
|
||
H |
|
|
H |
(36) |
|
|
(37) |
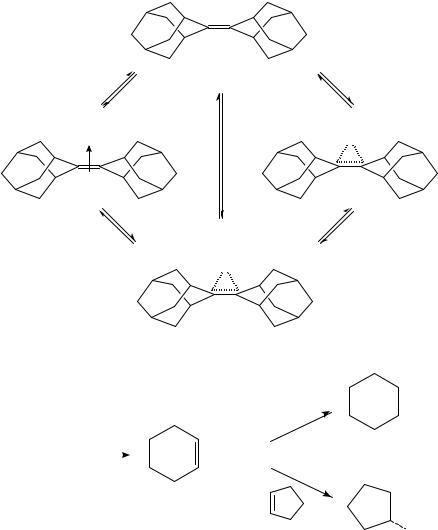
8. Complex formation involving double-bonded functional groups |
381 |
+ Br2
Br2 |
Br5− |
Br |
|
|
+ |
+Br2 |
+Br2 |
Br3 −
Br
+
SCHEME 13
Br
+Br− Br
36 |
+ Br− |
|
+ Br2 |
|
+Br−
Br
+
Br
SCHEME 14
Bromonium ion is generated by reactions of both erythro (38) and threo 2-bromo- 1,2-diphenylethane with hydrogen bromide95 (in 1,2-dichloroethane and in chloroform) as illustrated by Scheme 15. Stilbene (39) and the 1,2-dibromo derivative are the main reaction products. Product distribution and kinetic investigations on the bromination of stilbenes clearly confirm the reversibility of the bromonium/bromide formation in bromination of olefins, through an olefin/bromine CT complex.
Kinetic studies92 of the bromination of cyclohexene, alken-1-ols 41 and 4-pentenoic acid, by the bromonium ion of adamantylideneadamantane bear out the findings shown in Scheme 16.
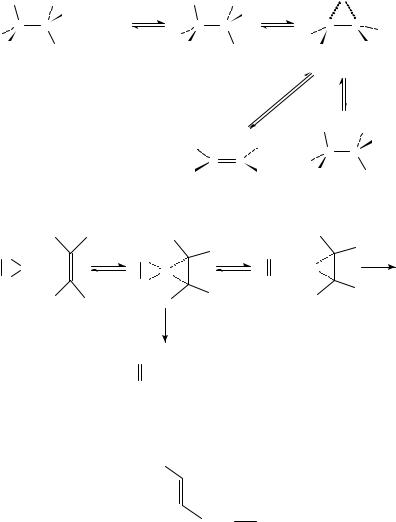
382 |
|
|
Luciano Forlani |
|
|
|
|
|
||
Br |
|
Ph |
Br |
|
Ph |
|
Br |
|
|
|
|
|
|
+ |
|
|
|
||||
|
|
H |
|
|
H |
|
|
|
+ H2 O |
|
C |
C |
+ HBr |
C |
|
C |
|
C |
C |
Ph |
|
H |
|
|
H |
|
|
|
H |
|
|
|
|
|
|
+ OH2 |
|
|
H |
|
|||
Ph |
|
OH |
Ph |
− |
|
Ph |
− |
|
||
(38) |
|
|
Br |
|
|
Br |
|
|
||
|
|
|
|
|
|
|
|
|
|
|
|
|
|
|
|
|
−Br2 |
|
|
|
|
|
|
|
|
|
|
|
Br |
|
Ph |
|
|
|
|
H |
|
|
Ph |
|
|
H |
|
|
|
|
|
|
C |
C |
|
|
||
|
|
|
C |
C |
|
|
|
|||
|
|
|
|
H |
|
|
|
|||
|
|
|
Ph |
|
|
H |
Ph |
|
Br |
|
|
|
|
|
|
(39) |
|
(40) |
|
|
|
|
|
|
SCHEME 15 |
|
|
|
|
|
||
Ad |
|
Ad |
+ |
|
|
Ad |
|
|
|
|
+ Br |
+ |
|
|
+ |
Br + |
|
|
products |
||
|
Br |
|
|
|
|
|||||
Ad |
|
Ad |
|
|
|
Ad |
|
|
|
|
|
|
|
|
|
|
|
|
|
|
|
(34) |
|
|
|
|
|
|
|
|
|
|
Ad
+ products
Ad
Ad Ad = adamantylideneadamantane
SCHEME 16
R
(CH2 )n OH
R = H, |
n = 3 |
R = H, |
n = 4 |
R = CH3 , n = 3
(41)
The addition of an initial amount of adamantylideneadamantane 33 to the reaction mixtures depresses the rate of the BrC transfer from 34 to the olefins, because of the competition of 33 with the other olefins (there is an equilibrium 33 34). In the case of the complex 34, 13C-NMR spectral data provide indications of the presence of a rapid transfer of BrC to olefins, as shown in Scheme 17, probably via a spiro transition state
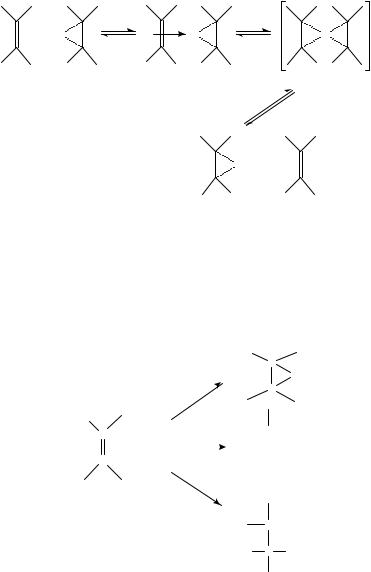
8. Complex formation involving double-bonded functional groups |
383 |
(43)75,91. The bromonium ion may exchange with free olefins through a complex73 (42 of Scheme 17).
+
+ X + |
X + |
X |
|
(42) |
(43) |
+ X +
X = Cl, Br
SCHEME 17
Rotational spectroscopy and ab initio calculations give an evaluation of the distance between donor and acceptor for ethene/halogen or interhalogen (BrCl) complexes73,96. Ab initio calculations indicate that a ‘spiro’ transition state73 (43) is involved in the transfer of positive halogens to olefins; an asymmetrical 1:1 halogen-ion/olefin CT complex (42) precedes the TS 43.
In the bromination mechanism different reaction pathways may compete68, as shown in Scheme 18.
R
C
+ Br
C
R
C |
+ Br2 |
|
R |
|
C + |
||||
|
|
||||||||
|
|
|
|
|
|
|
|
|
|
|
|
||||||||
C |
|
|
|
|
C |
|
Br |
||
|
|
|
|
|
|
||||
|
|
|
|
|
|
||||
|
|
|
|
|
|
|
|
|
|
C +
R C Br
SCHEME 18
The ‘multistep pathway’81 explains some observed features, including the evaluation of the electronic effect of substituents81,97 using the Hammett (/) equation which is a non-linear correlation, but it may also be explained by a single reaction with two or
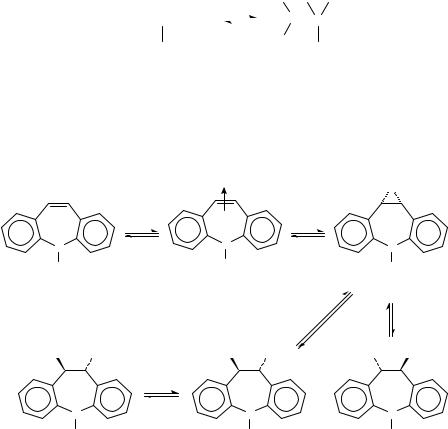
384 |
Luciano Forlani |
more steps involving different electronic requirements98. A halogen/olefin CT complex precedes the reactions of Scheme 18. The different pathways depend on the nucleophilic power of the solvent and on the nature of the olefin81,83. Steric hindrance is relevant to state the nature of the bromonium ion and the importance of the solvent for stabilization.
The chemoselectivity of olefin bromination is reported84 to occur after the attack of the bromine on the double bond, but the formation of the bromonium ion is the slow step of the reaction. As a consequence, the distribution of products and the selectivity of addition of nucleophiles can hardly be explained by substituent effects (both steric and electronic) bonded to the CDC double bond in a fast step of the reaction.
The reversibility of the formation of bromonium ion is a process comparable to the formation of ˇ-bromo carbocations. In fact, the carbocation formation may be solventassisted81 as reported in reaction 8, which parallels reaction 4.
|
|
|
|
|
|
|
R |
|
|
ROH + |
|
|
|
|
|
|
O . C+ |
Halog− |
(8) |
|
C |
|
Halog |
||||||
|
|
|
|||||||
|
|
||||||||
|
|
|
H
Furthermore, in the addition of bromine to 44 (in 1,2-dichloroethane, chloroform or carbon tetrachloride, see Scheme 19) to obtain the trans dibromo derivative99 (47), which is found to be in two main conformers100, the formation of the bromonium ion (46) from 5H-dibenz[b,f]azepine-5-carbonyl chloride (44) is a reversible step. The formation of the bromonium ion (46) follows the association of reagents in the CT complex (45). 49 reacts with hydrogen bromide through the protonated 48, forming99 both the dibromo derivative 47 and the olefin 44. The bromonium ion (46) is the probable intermediate
|
|
Br2 |
|
|
Br |
|
|
|
|
|
+ |
|
|
+Br2 |
|
|
|
N |
|
N |
|
|
N |
|
|
|
|
||
X |
|
X |
|
|
X |
|
|
|
|
||
(44) |
|
(45) |
|
|
(46) |
|
|
|
|
−H2 O |
|
HO |
Br |
H2 O+ |
Br |
Br |
Br |
|
N |
|
N |
|
N |
|
X |
|
X |
|
X |
|
(49) |
|
(48) |
|
(47) |
X = COCl
SCHEME 19
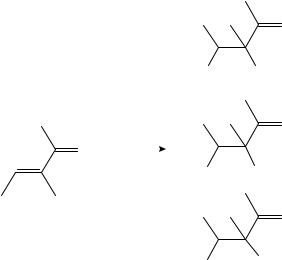
8. Complex formation involving double-bonded functional groups |
385 |
of these reactions. Clearly, 45 is formed in a reversible pathway from olefin (44) and bromine.
Usually, the attack of the nucleophile on the bromonium ion is a fast process. On the other hand, kinetic investigations100 on the bromination shown in Scheme 19 indicate that bromonium ion formation (i.e. the ionization of the CT complex 45) cannot be the RDS. The apparent activation energy for the overall bromination (and the experimental reaction order in bromine, which changes by changing the temperature) confirms the neutralization of the bromonium ion to form the product (47) in a step limiting the observed rate of the overall process.
The regiochemistry of the bromination (Scheme 20) of ˛,ˇ-unsaturated ketones101 (in methanol) by bromine is affected by the presence of N-bromosuccinimide, which also depresses the overall rate of bromination. A possible explanation of the observed behaviour is the presence of a complex (50) involving the CDC double bond of olefin, N-bromosuccinimide and bromine, as shown in Scheme 21. Probably, NBS acts as a hydrogen bromide scavenger, thereby causing a change in the bromination mechanism from an acid-catalysed pathway to a bromonium ion mechanism.
|
|
|
O |
|
|
CH3 O |
Br |
|
|
|
+ |
|
|
|
O |
O |
Br2 (CH3OH) |
|
|
|
|
|
|
Br2 /NBS |
|
||
|
|
||
|
|
Br |
OCH3 |
|
|
and/or: |
|
NBS = N-bromosuccinimide |
O |
||
|
|||
|
|
Br |
Br |
SCHEME 20
The rate of cyclohexene bromination, too (in carbon tetrachloride), is lowered by the presence of NBS (or of other hydrogen bromide scavengers)74,102; probably, the reaction is complicated by the presence of further complexes, such as the tribromide of the cyclohexene bromonium ion.
A mixture of ethene and molecular chlorine (in the gas phase), and of the isotopomers C2H5 ! 37Cl2, C2H5 ! 35Cl37Cl and C2H5 ! 37Cl35Cl, was investigated by rota-
tional spectra obtained by a pulsed FT microwave spectrometer103 (together with other complexes of molecular chlorine and HCN, PH3)104.
The observations strongly support the hypothesis that the addition of molecular chlorine to the CDC double bond follows the reactions shown in Scheme 10 (X D Cl). The formation of cation 27 (and/or 28) is the RDS and the reaction, in dark and in fairly polar solvents, is of the second-order law, first order in both reagents.
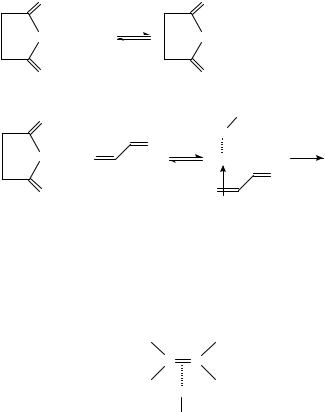
386 |
|
Luciano Forlani |
|
O |
|
O |
|
NBr |
+ Br2 |
NBr3 |
|
O |
|
O |
|
(NBS) |
|
|
|
O |
|
|
NBS |
|
|
|
|
|
|
Br |
|
|
+ |
O |
|
NBr3 |
Br |
products |
|
|
|
|
O |
O |
|
|
|
(50)
SCHEME 21
The formation of 27 is preceded by a rapid equilibrium that forms complex 26 (of Scheme 10, X D Cl): there is evidence of the existence of a molecular complex between the halogen and the CDC double bond (51) by means of a weak interaction. The Cl2 molecule lies along the C2 axis of ethene that is perpendicular to the molecular plane, as shown in 51. The distance between the CDC bond and the inner chlorine atom is 3.128 A˚ .
C C
Cl
Cl
(51)
The geometry of the complex between ethene and molecular chlorine, and the strength of these complexes are subjects of discussion105.
G. Interactions of Fluorine with C=C Double Bond
The number of reactions of addition of fluorine to the CDC double bond is not as large as that of addition of chlorine or bromine, and few examples of practical application of reactions of elemental fluorine are available. However, there is wide scope for development106 of these reactions for preparative purposes.
The behaviour of elemental fluorine differs from that observed for chlorine or bromine, mainly because fluorine addition proceeds in a stereoselective syn manner (Scheme 22). The syn addition is explained by the formation of tight ion pairs forming the addition
products or carbocation rearrangement products107. |
|
|
|
The ion-pair formation is preceded by an initial equilibrium, |
which quickly leads |
||
to |
a perpendicular complex (Scheme 23). A similar reaction |
pathway |
is reported |
for |
the reactions of iodine (and bromine) monofluorides107,108. |
Also, the |
addition of |
fluoroxytrifluoromethane CF3OF to both cis- and trans-stilbenes109 enables a syn addition