
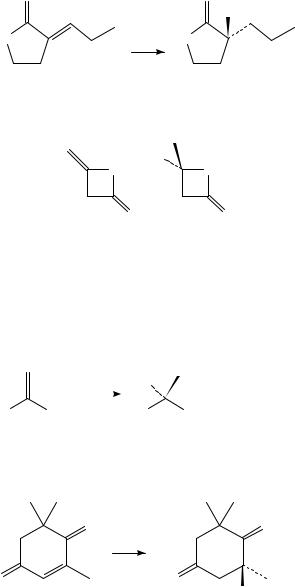
15. Hydrogenation of compounds containing CDC, CDO and CDN bonds 801
O |
O |
|
H |
O |
O |
i |
|
|
95% e.e. |
Reagents:(i)[Ru(BINAP)(OAc)2 ], H2 |
|
SCHEME 22 |
|
H |
|
O |
O |
O |
O |
(20)(21)
unsaturated lactones containing endo double bonds are reduced in much lower e.e.138b. An unusual yet highly selective reduction is also observed in the conversion of butyrolactone 20 to 21 in 70% e.e. using Ru/BINAP139. The DuPHOS ligand P13 and the related P14 have proven to be ideal ligands for the asymmetric reduction of enol acetates when used in conjunction with rhodium(I) (Scheme 23)91a. Asymmetric hydrogenation of ˛,ˇ-
unsaturated aldehydes and ketones remains difficult140, although some very interesting results have been reported, e.g. Scheme 24140a.
|
|
i |
H |
R = CO2 Et, 99% e.e. |
|
|
|
||
R |
|
|
R |
R = CF3 , 95% e.e. |
OAc |
OAc |
Reagents:(i) Rh(I).PH, H2
SCHEME 23
O O
i
50% e.e.
O O
H
Reagents:(i) [Ru(BINAP)Cl.benzene].Cl, H2 , MeOH
SCHEME 24
Alcohols may be employed to aid the direction of asymmetric reductions of proximal double bonds. Even polyenes are very selectively reduced at only the double bond closest
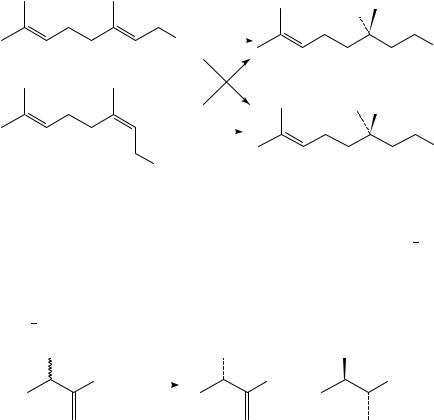
802 |
Martin Wills |
to the hydroxyl group. Many excellent result have been obtained, most notably in the reduction of allylic alcohols using Ru/BINAP combinations (Scheme 25) and in particular [Ru(BINAP)(OAc)2]141. The direction of the reduction is usually predictable and the selectivity high. A high pressure of hydrogen is not required however, and very low levels of catalyst (substrate/catalysts ratios as high as 50,000 are viable at 30 atm H2) may be successfully employed. Detailed full papers on this work have been published141b and the use of closely related ligands has been reported142.
|
|
|
|
|
H |
|
OH |
Ru/S-BINA P |
|
||||
|
|
|
OH |
|||
H2 |
||||||
|
|
|||||
|
|
− 99% e.e. |
||||
96 |
||||||
|
|
Ru/S-BINA P |
|
|||
|
|
|
|
H2 |
|
|
|
|
|
|
|
H |
|
|
|
|
|
|||
|
Ru/S-BINA P |
OH |
||||
|
|
H2 |
||||
|
|
|
||||
OH |
98% e.e. |
SCHEME 25
Kinetic resolutions may be achieved in asymmetric hydrogenations of racemic cyclic allylic alcohols, and in some cases impressive levels of discrimination have been achieved143. In the example given in Scheme 26, using a substrate/cat ratio of 200 1800, the starting material was recovered with an e.e. >99% at a conversion of 76%. Reduction of this recovered material with a non-chiral catalyst was then employed to give an enantiomeric product containing two chiral centres. In a variation on the ‘chiral poisoning’ approach, the combination of racemic BINAP/Rh complex with a ( )-1R,2S-ephedrine resulted in a good level of enantiodifferentiation in the reaction shown in Scheme 27144. At 77 79% conversion the unreacted starting material had an e.e. of >95%.
OH |
|
OH |
OH |
CO2 Me |
i |
CO2 Me + |
CO2 Me |
|
|||
|
|
> 99% e.e. at 76% conversion |
47:1 threo : erythro |
|
|
threo:37% e.e. |
Reagents:(i) [Ru(S-BINAP)(OAc)2 ], s/c 200-1800, 4 atm H2 , 25 ˚C, 11 h
SCHEME 26
Water solubility may be imparted upon chiral diphosphine ligands by the incorporation of polar groups. Whilst sulphonic acids have most commonly been employed90a,145, amines have also been successfully used in this respect90b,c. Another approach is the use of surfactants or micelle-forming amphiphiles to permit neutral ligands to be used in an aqueous environment85c,88b. In all these cases the asymmetric inductions are similar to the level achieved by the original ligands and conditions.
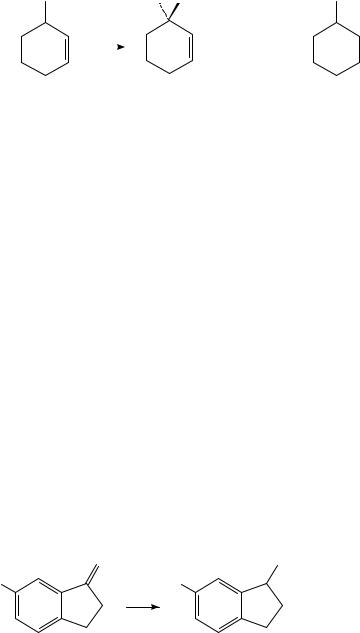
15. Hydrogenation of compounds containing CDC, CDO and CDN bonds 803
OH |
|
H OH |
OH |
|
|
> 93% e.e. at |
|
|
i |
77 |
− 79% conversion + |
|
|
|
Reagents:(i) Ru(±)BINAP cpx, (−)-(1R,S)-ephedrine
SCHEME 27
Immobilization of homogeneous catalysts has the advantage of practical simplicity in terms of use and environmental compatibility, since removal of the metal complex is facile146. Heterogeneous modification of a homogeneous system has been achieved by an unusual strategy in which a Ru(II)/sulphonated-BINAP catalyst is contained within a layer of ethylene glycol on the surface of small particles of glass suspended in an organic solvent. Using this system Naproxen can be prepared by the asymmetric hydrogenation of the precursor acrylic acid (Scheme 20) in up to 95.7% e.e.127b,c. Leakage of catalyst from the ethylene glycol is claimed to be minimal in this reagent, which can be recycled and reused without loss of activity.
Z-Acylaminocinnamic acids have been successfully hydrogenated in high enantiomeric excess using DuPHOS.Rh complexes in supercritical carbon dioxide91g. In some cases the e.e.s of the products exceed those achieved using methanol or hexane solvents.
Modified ligands for asymmetric hydrogenation have been prepared by the incorporation of other function groups such as alcohols147, or acceptors such as borane148, in order to modify their properties and utility in unusual environments. Reports have appeared on the incorporation of chiral diphosphines within dendrimers149 and appended to a peptide backbone150. The latter objective is the development of an optimized ligand by combinatorial modification of the peptide.
Reduction of simple alkenes. Few good methods exist for the asymmetric reduction of CDC bonds which contain no available groups for co-ordination to the chiral metal complex. One recent notable exception is the use of a Ru(II)/BINAP complex which is reported to be capable of the reduction of exo terminal alkenes in e.e.s of up to 78% (Scheme 28)151. Rather more success has been achieved using chiral titanocene catalysts and related organometallics152. Following some very promising early reports153 a series of very valuable and versatile reagents has emerged. The complex 22 for example, catalyses the reduction process summarized in Scheme 29, for which substrates 23 to 26 are reduced with the e.e.s shown154. It should be noted that the actual active catalyst is formed by treatment of 22 with 2 equivalents of n-butyllithium and 2.5 equivalents of diphenylsilane prior to use. The related samarium complex 27 catalyses the reduction of simple alkenes
R |
R |
|
|
||
i |
|
n =5, 6, up to 78% e.e. |
( ) |
( ) |
n |
n |
Reagents:(i) [Ru. (BINAP) (OAc)2 ], H2 |
|
|
SCHEME 28 |
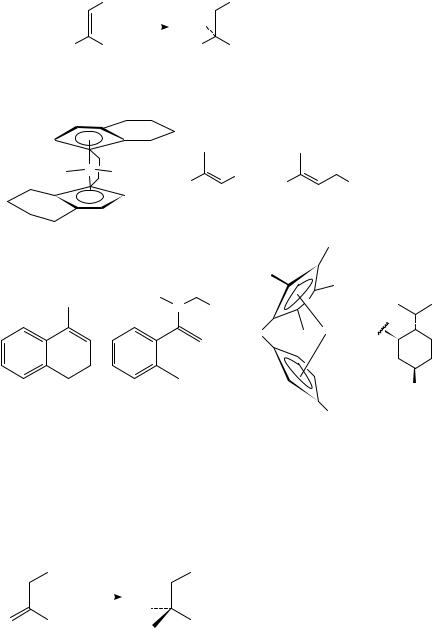
804 |
|
|
Martin Wills |
|
|
R2 |
|
R2 |
|
|
|
i |
H |
70−90% yield |
|
|
|
83−94% e.e. |
|
|
|
|
|
|
R |
R1 |
R |
R1 |
Reagents:(i) Catalyst from 22 + 2 eq. nBuLi+ 2.5 eq. Ph2 SiH2 , 2000 psiH2
SCHEME 29
Cl |
Ti Cl |
Ph |
|
|
Ph |
Ph |
NBn2 |
|
|
>99% e.e. |
95% e.e. |
|
(22) |
(23) |
(24) |
N Ph
Me2 Si SmCl2
R =
93% e.e. 96% e.e.
(25) |
(26) |
(27) |
R |
in up to 96% e.e. after activation in the same way, but at lower temperature and hydrogen pressure155.
An excellent review describing asymmetric transfer hydrogenation has been published156. Many excellent results have been achieved in recent studies of acrylic acid reductions employing the same catalysts of ruthenium or rhodium with a chiral diphosphine as were used in the hydrogen gas process133f,157. In this case, however, the most common hydrogen source is the combination of formic acid with an amine. The choice of amine is often critical; in the reduction shown in Scheme 30, the use
CO2 H |
CO2 H |
Amine |
e.e. |
||
S-α-methylbenzylamine |
>97% |
||||
|
|
|
|||
|
i |
|
R-α-methylbenzylamine |
81− 87% |
|
|
|
H |
Benzylamine |
87− 89% |
|
|
|
|
|
||
CO2 H |
CO2 H |
|
|
Reagents:(i) Rh(I).(S)-BPPM (P7), HCO2H, R or S-α-methylbenzlamine
SCHEME 30
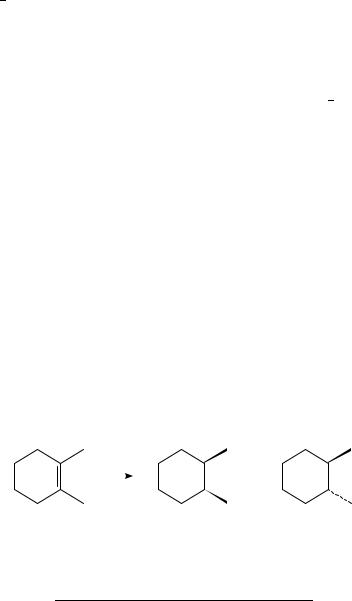
15. Hydrogenation of compounds containing CDC, CDO and CDN bonds 805
of S-˛-methylbenzylamine gives product in >97% e.e. whilst use of the R-amine gives only 81 87% e.e.133f. In some cases an alcohol, often isopropanol, may be employed to
provide the hydrogen in this class of reduction157c.
B. Heterogeneous Methods
1. General comments
Heterogeneous hydrogenation of CDC bonds is a very well established synthetic process which is widely employed and has been extensively reviewed158 160. The most commonly used catalysts are finely dispersed metals such as palladium, rhodium, nickel or platinum supported on a neutral support such as alumina, silica or carbon (graphite). These reagents have the particular advantage associated with ease of use and recoverability and reusability. One disadvantage of the method is that facile double-bond isomerization can take place in certain cases. More dramatic rearrangements are observed in other cases, although homogeneous catalysts also suffer the same drawbacks on occasions161. The aim of this review will not be to extensively survey this area, or to discuss the kinetics and the transport phenomenon of the reactions, but to emphasize some of the more recent highlights of the literature.
Heterogeneous catalytic hydrogenation takes place on the surface of the catalyst, and in general cis-addition of hydrogen to alkenes is observed. A small amount of stereochemical control can be lost due to the problem of isomerization, although this can often be minimized by the correct selection of catalyst (Scheme 31/Table 3)158. The process of hydrogenation is very complex; a recent report described, for the first time, proof that ethylene on the Ni(III) surface was reduced by bulk hydrogen moving out of the metal to the surface, whilst the surface hydrogen had no effect162. Many efforts have been made to improve the definition of the surface of heterogeneous catalysts. Some of these have focussed on the preparation of carbon ‘nanotubes’ of defined shape and size designed to admit only substrate molecules or a specific size range, for example163. The same objective is shared by palladium catalysts suspended in hollow polymer fibres, some of which show improved selectivity for diene over monoene reduction164. Palladium catalysts supported in montmorillonite clays have been found to be capable of selective reductions of methyl acrylates over acrylates containing larger groups at the ester position165.
i |
+ |
|
Reagents:(i) Metalheterogeneous c atalyst (see below), H2
SCHEME 31
TABLE 3. Selectivity of reduction in Scheme 31
Metal |
atm H2 |
cis:trans |
|
Pt |
1 |
82 |
:18 |
Pt |
300 |
96 |
:4 |
Os |
1 |
98.4:1.6 |
|
Ir |
1 |
98.9:1.1 |
|
|
|
|
|
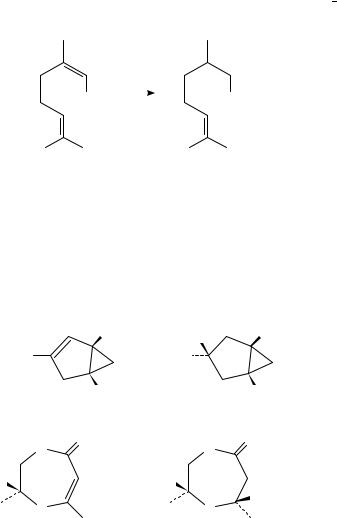
806 |
Martin Wills |
A size-selective synthesis of nanostructured transition metal clusters (Pd, Ni) has been reported166, as has the preparation of colloidal palladium in organic solvents167, the latter of which is an active and stable catalyst for selective hydrogenation. The use of microwaves in the preparation of palladium catalysts on alumina and silica resulted in hydrogenation catalysts with improved crystallite size and activity168.
The selective reduction of the CDC bond in enones is generally favoured over the reduction of isolated double bonds using a variety of catalysts (e.g. Scheme 32)169. The incorporation of a lanthanide(III) additive can increase the selectivity of CDC reduction over CDO in some cases170. New methods have been reported for the full reduction of aromatic rings171, and for methods of reduction to the cyclohexene product172. The heterogeneous hydrogenation of C60 to C60H2 has been reported173, and, conversely, the preparation and use of a hydrogenation catalyst based on C60 [C60Pdn (n D 4.61 5.15 ] for the reduction of alkenes and alkynes has been described174.
i
CHO |
|
CHO |
Reagents: (i) NH4 HCO3 , Pd/C, 25°C, 4h
SCHEME 32
2. Diastereoselective reductions
As was the case for homogeneous catalysts, certain reactive groups can mediate hydrogenation reactions through either a steric blocking effect or a co-ordinating, directing effect. Steric effects resulting in the blocking of a reaction on one face of an alkene are
generally easy to identify and explain158,175. The selective reductions of 28 to 29176, of 30 to 31177 and of 32 to 33178 serve as typical examples. The latter example served as a key
|
NHBoc |
H |
NHBoc |
|
|
|
|
MeO2 C |
|
MeO2 C |
|
|
H |
|
H |
(28) |
|
(29) |
|
H |
O |
H |
O |
N |
|
N |
|
H |
|
H |
|
O |
|
O |
H |
|
|
||
(30) |
|
(31) |
|
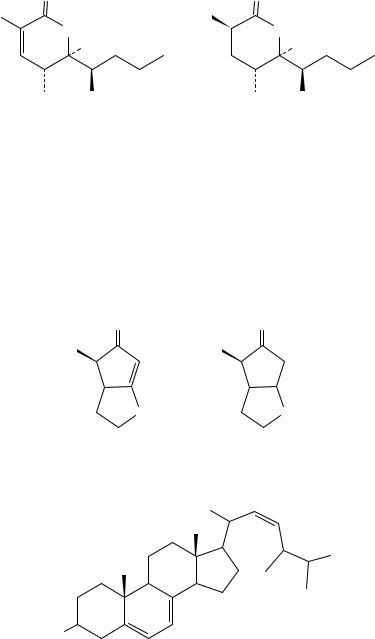
15. Hydrogenation of compounds containing CDC, CDO and CDN bonds 807
O |
O |
O |
O |
H |
H |
(32) |
(33) |
step in the synthesis of invictolide. Diastereoselective reductions of acylaminoacrylates attached to chiral auxiliaries have been employed as effective methods for the asymmetric synthesis of many amino acids179. A similar strategy has been employed for the asymmetric synthesis of 1,4-diesters and their derivatives180. The reduction of the CDC bond in enones is readily achieved using hetereogeneous catalysts (Scheme 33)181, although this can on occasions be accompanied by the formation of acetals, assuming that an alcohol is employed as solvent182. Transfer hydrogenation methods may also be employed in heterogeneous hydrogenations, usually with the use of a formate salt to provide the hydrogen183,184. Dramatically different selectivities can be observed depending on which method is used; reduction of 34 with a copper or alumina catalyst and hydrogen gas in toluene solution gives 35 as the major product, whilst transfer hydrogenation conditions (cyclohexanol, 140 °C) furnish the diasteoisomeric product 36184.
O O
i
H H
H O O
Reagents: (i) 10% Pd/C, 60 psi H2 , T HF, 60 h
SCHEME 33
HO
(34)
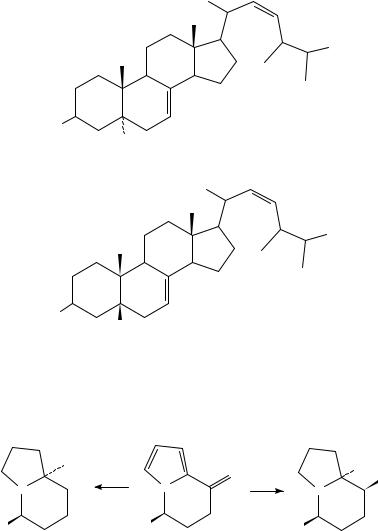
808 |
Martin Wills |
HO
H
(35)
HO
H
(36)
The reduction of heterocyclic compounds is a versatile process of great use in the synthesis of alkaloids185. The choice of catalyst is crucial however, as illustrated by the indolizidine alkaloid synthesis shown in Scheme 34185a. The reduction of 37 to 38 proceeds with a very high degree of stereocontrol which sets up the correct framework for intramolecular cyclization to complete a short synthesis of -lycorane 39186.
|
H |
O |
|
H |
|
i |
ii |
OH |
|
|
|
|||
N |
N |
|
N |
|
|
|
|||
MeO2 C |
MeO2 C |
|
|
MeO2 C |
Regants. (i) H2 , Pd/C, H2 SO4 or AcOH, (ii) Rh/Al2 O3 , H2
SCHEME 34
The stereocontrol of reduction reactions by co-ordinating groups is far less prevalent than in the case of homogeneous catalysts, in which discrete molecular species are involved. There is evidence in some cases that this sort of effect might be operating through interactions of hydroxy and amino groups, although it is by no means a common phenomenon and is restricted only to certain metals. Examples are given in Schemes 35 and 36187. In the case of nickel an anchoring effect appears to be operating, whilst in other cases the functional group exerts only a steric effect. The reduction of citronellol using a
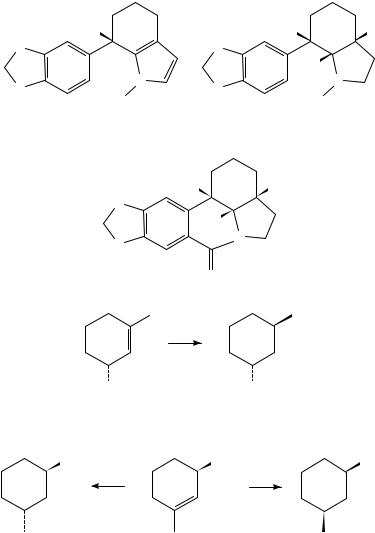
15. Hydrogenation of compounds containing CDC, CDO and CDN bonds 809
H |
H |
H |
O |
O |
|
|
H |
|
N |
O |
N |
O |
|
|
ButO2 C |
ButO2 C |
|
(37) |
(38) |
|
H |
H |
|
O |
|
|
|
H |
|
O |
N |
|
|
|
|
|
O |
|
(39) |
|
|
i |
|
|
OH |
OH |
|
Reagents: (i) Ni catalyst, H2 |
|
|
SCHEME 35 |
|
|
NH2 |
NH2 |
NH2 |
i |
ii |
|
80% |
60% |
|
Reagents:(i) Nior Pd, H2 , (ii) Pd/C, H2
SCHEME 36
platinum/alumina catalyst modified with a carboxylic acid is selective for the double bond proximal to the alcohol, which is highly suggestive of a directing effect (Scheme 37)188.
3. Enantioselective reductions
Unlike the reductions of CDO bonds, asymmetric methods of heterogeneous hydrogenation are relatively rare. In general, this area is difficult to study because of the difficulties
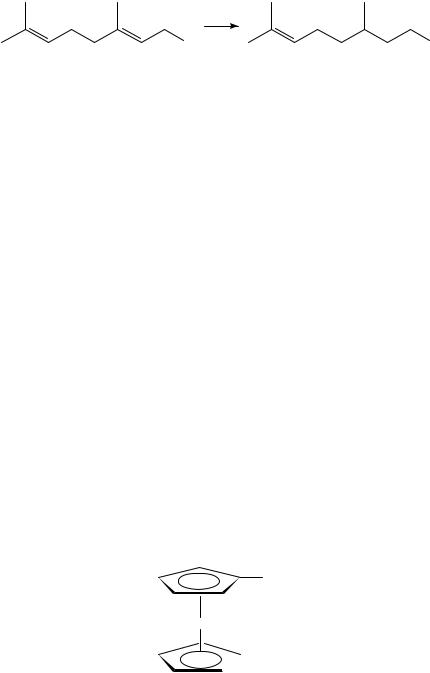
810 |
Martin Wills |
i
OH |
OH |
Reagents: (i) H2 , Pt/Al2 O3 modified by RCO2 H
SCHEME 37
associated with the formation of a catalyst of a predictable and reproducible structure, which is an essential requirement. Enantiomeric excesses of 17% and 38% have been achieved through the use of a tartaric acid modified raney nickel189 and a palladium black modified by ( )-dihydrovincolide alkoloid190, respectively. Rather better results have been achieved in the reduction of Z-˛-(acylamino)acrylates with a rhodium phosphine complex tethered to a zeolite support. In this example, where as little as 0.01 mol% catalyst is required, enantiomeric excesses of up to 97.9% have been achieved191. The large pore size of the zeolites selected for study is believed to be critical to the success of the catalyst.
III.HYDROGENATION OF C=O BONDS
A.Homogeneous Methods
1. General comments
Unlike the heterogeneous variant, homogeneous hydrogenation of CDO bonds has not developed at the rate of the corresponding CDC reduction process. This is due mainly to the lack of early success in the use of the Wilkinson catalyst in this respect. In work that has been described elsewhere192, the selection of the correct phosphine within a cationic complex was critical. Since these breakthroughs, progress in this area has been made at a tremendous pace, particularly in the area of asymmetric reductions, as will be described below.
New catalysts for the hydrogenation of CDO bonds continue to be reported. The ferrocene-based diphosphine 40, in the form of a cationic rhodium complex [(COD)Rh(40)]OTf, is an excellent catalyst, promoting rapid hydrogenation, at the 0.2 mol% level, of ketones and aldehydes at room temperature at 60 psi hydrogen pressure193. New cationic ruthenium194 catalysts containing bipyridyl ligands have also been reported, although even the neutral [RuCl2(PPh3)3] complex is an effective catalyst for CDO bond hydrogenation at the 0.1 mol% level, a result which demonstrates the improved compatibility of this metal compared to rhodium195.
PPh2
Fe
PPh2
(40)