

21. Strained olefins |
1273 |
R
H
(20a) R = H
(20b) R = Me
In spite of the deformations, the strain energy in these compounds is not excessive. EFF calculations by Inoue and coworkers99 predict a difference in strain energy of 9.7 kcal mol 1 between E- and Z-cyclooctene (11.3 kcal mol 1 according to Allinger and Sprague95) and 11.7 kcal mol 1 for the 1-methyl analogues. The strain energy for
20a is calculated to 6.3 kcal mol 195 .
E-Cyclooctene is chiral, and it was resolved into enantiomers by Cope and coworkers100 by separation of diasteromeric platinum complexes containing 20 and (C)-phenyl-2- aminopropane as ligands. Thermal racemization occurred around 150 °C with a rate
corresponding to a barrier of ca 35 kcal mol 1101 .
The original synthesis of E-cyclooctene was performed by a Hofman degradation of trimetylcyclooctylammonium hydroxide102. However, photoisomerization of the Z isomer has emerged as an attractive alternative. Direct isomerization by irradiation with UV light of 185 nm in pentane gives a photostationary state with an E/Z ratio of 0.98103,104, and similar results are obtained by irradiation of E-1-methylcyclooctene at 214 nm99. A good preparative method is singlet-sensitized isomerization using (poly)alkyl benzene(poly)carboxylates as sensitizers99. The mechanism involves exciplex formation with twisted singlet cyclooctene, and by using a chiral singlet sensitizer, e.g. ( )hexa- 1-methylheptyl benzenehexacarboxylate at 87 °C in pentane, an enantiodifferentiating transformation of Z- to E-cyclooctene with an enantiomeric excess (ee) of 52.7% of (C)- E-cyclooctene was achieved105,106. The ee is strongly sensitive to the nature of the chiral sensitizer and generally increases with decreasing temperature. In fact, reversal of the absolute configuration of the major enantiomer at higher temperatures was observed in some cases, which could be explained as an effect of the entropy of activation. In a later work107, even better results were obtained with sensitizers containing a phenyl or cyclohexyl group attached to the chiral component of the sensitizer. The highest ee, 63.5%, was reached with tetrakis-8-cyclohexylmenthyl benzene-1,2,4,5-tetracarboxylate as sensitizer at 89 °C. A similar study of enantiodifferentiating Z- to E- photoisomerization of 20b has recently been reported108.
1,3- and 1,5-Cyclooctadienes are also known as E,Z isomers. E,Z-1,5-cyclooctadiene (21) has been resolved and is stable at not too high temperatures109. However, at ca 150 °C it undergoes a sequence of Cope rearrangements, ending up in Z,Z-1,5-cyclooctadiene110. The 1,3-analogue (22) has been prepared both by stereoselective elimination111 and by photoisomerization112. It has been resolved into enantiomers by chromatography with swollen, microcrystalline triacetylcellulose as stationary phase113, and optically active 22 has been obtained in 17.6% ee in a stereoselective singlet-sensitized photoisomerization with hexamenthyl benzenehexacarboxylate as sensitizer114. Thermal racemization of 22 is not feasible, since already at C90 °C an electrocyclic reaction takes place with appreciable rate, leading to cis-bicyclo[4.2.0]oct-7-ene (23)115,116.
Bouman and Hansen have calculated the CD spectra of (M)-20a and (M)-22 by ab initio methods117,118. Positive rotation was predicted for both compounds. The main contribution to the rotational strength in both compounds comes from the twisted E double
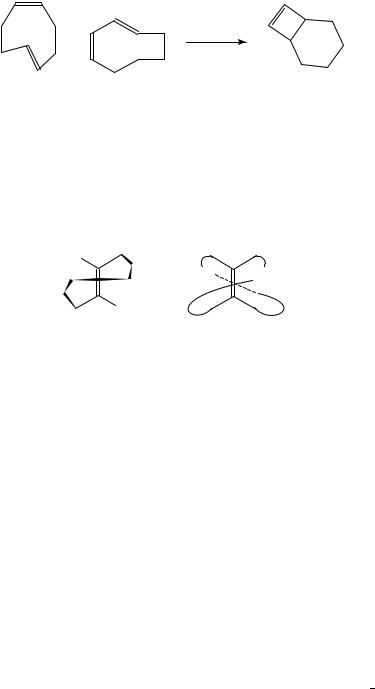
1274 |
Jan Sandstrom¨ |
|
|
|
• |
|
90 °C |
• |
|
t1/2 34 min |
|
(21) |
(22) |
(23) |
bond. More recently, Hansen and coworkers performed similar calculations for 20a with a more elaborate basis set and with a similar result119. The so-called diene helicity rule120, which predicts positive rotational strength for dienes with a positive CDC CDC dihedral angle (P helicity for the E double bond), is not valid for 22.
Even in E-cyclononene (24) the ‘jump-rope’ movement of the saturated chain is sufficiently hindered to permit the isolation of enantiomers, but the free-energy barrier to
racemization was found to be as low as 19.1 š 0.2 kcal mol 1121 . A complete EFF search of the conformational space of 24 has recently been published, leading to a barrier of
21.6 kcal mol 1122 . Earlier EFF calculations predicted the strain energy of 24 to be zero95.
H
(CH2 )m (CH2 )n
H
(24) |
(25) |
Resolution of E-cyclodecene has not succeeded, but its 1,2-dimethyl derivative as well as the corresponding cycloundecene derivative have been obtained in optically active form123.
An interesting group of bicyclic cycloalkenes, the so-called [m,n]betweenanes, contain a double bond, which has E configuration in both rings (25). Most representatives have m and n > 6 and have rather low strain or are strain-free123, but a successful synthesis of a [6.10]betweenane has been reported124.
VI. BIAND POLYCYCLIC BRIDGEHEAD OLEFINS
As mentioned in Section I, Bredt’s rule prohibits double bonds at bridgeheads. For a time, the rule was assumed to be generally valid, but as exceptions began to emerge around 1950, they were, and are often still referred to as Bredt’s rule violators. An early case of a stable though reactive bridgehead olefin is 26125,126. The double bond is part of one Z- and one E-cycloalkene, and a stable product is expected when m C n 5 in 27, i.e. the E-cycloalkene is eight-membered or larger127. However, a number of more strained bridgehead olefins have been studied. A remarkably stable E-cycloheptenoid bridgehead olefin is adamantyl-homoadamantene (28), which can be heated to 185 °C without decomposition128. The likewise cycloheptenoid 29 is much less stable, but Michl and coworkers have isolated it in matrix and in solution and studied its spectroscopic properties129.
Using intramolecular Diels-Alder reactions, Lease and Shea130 have prepared a series of bridgehead olefins, which at the same time are bridgehead lactams with the nitrogen atom at the other bridgehead (30). In these, the CDC double bond and the amide bond are subject to the same kind of distortion. The distortions decrease in the series 30a 30c.
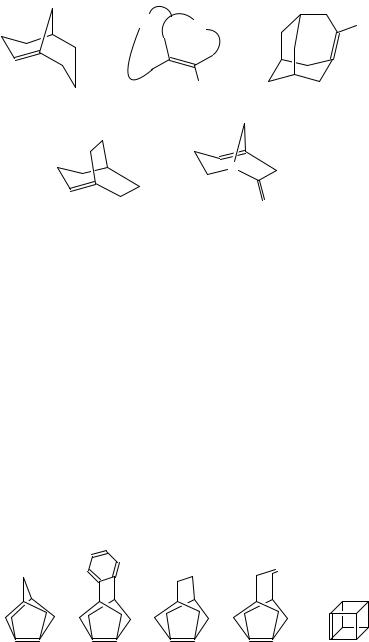
21. Strained olefins |
1275 |
|
(CH2 )m |
(CH2 )n |
Ad |
|
H |
|
(26) |
(27) |
(28) |
|
N |
(CH2 )n |
|
|
O |
(29) |
(30a) |
n = 2 |
|
(30b) |
n = 3 |
|
(30c) |
n = 4 |
In 30a, both the amide nitrogen and the double-bond bridgehead carbon are strongly pyramidalized with somewhat twisted bonds but the deformation at the nitrogen is much stronger than that the carbon.
VII. MISCELLANEOUS COMPOUNDS
In suitable tricyclic alkenes, the steric constraints force the double bond to assume a syn-folded structure. Borden and coworkers have described some highly strained representatives of the series of bridged bicyclo[3.3.0]oct-1(5)-enes. While the passing existence of 31 could only be inferred from its addition product131, 32132 and 33133 could be isolated and studied by a matrix technique, and 34a was stable at ambient temperature134. Its methiodide 34b was subjected to a study by X-ray crystallography and showed pyramidalization angles ( , see Scheme 1) of 20.3 and 12.3°, respectively, at the two double-bond carbons. Hrovat and Borden135 report ab initio, MNDO and EFF calculations of the OS values of 31, 33 and 34c. The results (Table 5) depend to a great extent on the method, but they fall in the expected order. The OS of cubene (35) is calculated to be somewhat larger than that of 31, but 35 was still predicted to be capable of existence, and as for 31 its formation could be inferred from its reaction products136.
X
(31) |
(32) |
(33) |
(34a) |
X = Se |
(35) |
|
|
|
(34b) |
X = SeMe+ |
|
|
|
|
(34c) |
X = CH2 |
|
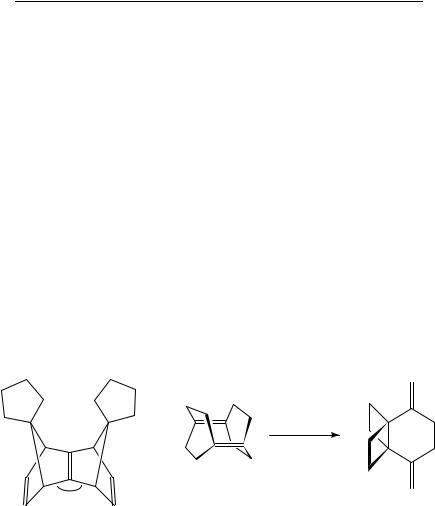
1276 |
|
|
|
Jan Sandstrom¨ |
|
|
|
|
|||
|
TABLE 5. |
Olefinic strain energy (OS, kcal mol 1) calculated for compounds 31 and 33 |
|
35135 |
|||||||
|
|||||||||||
|
Compound |
|
|
3-21G |
|
|
6-31GŁ |
|
|
|
|
|
|
|
SCF |
TCSCFa |
|
SCF |
TCSCFa |
MM2 |
MNDO |
||
31 |
59.5 |
49.5 |
62.1 |
52.3 |
24.5 |
66.6 |
|||||
33 |
38.8 |
33.3 |
41.7 |
37.4 |
18.2 |
46.9 |
|||||
|
34c |
16.0 |
13.9 |
19.2 |
17.1 |
9.8 |
30.8 |
||||
35 |
75.1 |
63.1 |
68.2 |
58.9 |
|
|
|
|
a Two-configuration SCF. |
|
|
|
|
|
TABLE 6. |
Pyramidalization angles ( and |
) for com- |
|
|
pounds containing strongly pyramidalized double-bond car- |
|||
|
bon atoms |
|
|
|
|
|
|
|
|
|
Compound |
(deg) |
(deg) |
Reference |
|
|
|
|
|
36 |
32.4 |
22.7 |
140 |
|
18 |
19.7 |
44.9 |
90 |
|
|
34b |
20.3 |
46.2 |
134 |
37 |
12.3 |
42.1 |
|
|
27.3 |
35.6 |
142 |
||
38 |
7.0 |
7.8 |
143 |
|
|
|
|
|
|
Weakly pyramidalized double-bond carbon atoms are found in norbornenes137, an observation that has aroused the interest of theoreticians138. The deviation from planarity has been found to increase in syn-sesquinorbornenes and even more in analogues containing peripheral double bonds139. The largest deviation from planarity has been observed for the syn-sesquinorbornatriene 36140. The pyramidalization is measured by two angles, (see Scheme 1) and , the latter being the angle between the planes meeting at the double bond (the flap angle). The values for these angles in 36 together with those for some other compounds containing pyramidalized double-bond carbons are found in Table 6. The value found for 36 is the highest so far reported for a compound isolable at ambient temperature. Analogues of 36 without cyclopentane rings have been prepared, but their study has been hampered by their extreme sensitivity to atmospheric oxygen141.
t1/2 = 1.1 min
100 °C
180°− ψ |
(37) |
(39) |
(36) |
|
|
An interesting series of polycyclic olefins with notable pyramidalization in the lower members is represented by the ‘superphanes’ 37142 and 38143. Their pyramidalization angles are shown in Table 6. While 37 could be obtained as a solid at ambient temperature
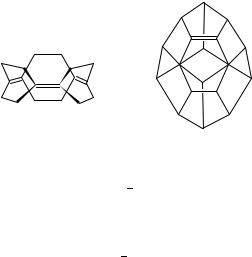
21. Strained olefins |
1277 |
(38) |
(40) |
but underwent a Cope rearrangement to 39 at slightly elevated temperature, 38 could be purified by sublimation and showed mp 259 259.5 °C without decomposition. The He I photoelectron spectrum of 38 shows a small splitting, indicating homoaromatic interaction between the double bonds.
Dodecahedrene (40) is a highly strained hydrocarbon, for which calculations predict42.5° (MM2) and 41.7° (MNDO)17. It has not yet been isolated, but there is strong evidence for its formation in a gas-phase ion molecule reaction in a FT ion cyclotron mass spectrometer144. A tetrasubstituted derivative has been studied in condensed phase145.
VIII. CONCLUSIONS
In the last decades, introduction of new synthetic methods66,146,147 has increased the availability of crowded organic molecules containing CDC bonds, and methods for preparing and studying labile molecules have been developed and refined. The deformations resulting from the strain in CDC bonds in different environments and the limits for existence of strained olefins have been explored in depth, and the theoretical treatment of strained systems by EFF and quantum chemical methods has developed greatly.
IX. REFERENCES
1.K. Wiberg, Angew. Chem., Int. Ed. Engl., 25, 312 (1986).
2.O. Ermer, Aspekte von Kraftfeldrechnungen, Wolfgang Baur Verlag, Munchen,¨ 1981.
3.U. Burkert and N. L. Allinger, Molecular Mechanics, ACS Monograph 177, American Chemical Society, Washington, D.C., 1982.
4.E. Osawa and H. Musso, Top. Stereochem., 13, 117 (1982).
5.E. Huckel,¨ Grundzuge¨ der Theorie ungesattigter¨ und aromatischer Verbindungen, Verlag Chemie, Berlin, 1938.
6.J. E. Douglas, B. S. Rabinovitch and F. S. Looney, J. Chem. Phys., 23, 315 (1955).
7.R. C. Haddon, J. Am. Chem. Soc., 108, 2835 (1986).
8.R. C. Haddon, Acc. Chem. Res., 21, 243 (1988).
9.R. C. Haddon, J. Am. Chem. Soc., 112, 3385 (1990).
10.W. F. Maier and P. von R. Schleyer, J. Am. Chem. Soc., 103, 1891 (1981).
11.A. B. McEwen and P. von R. Schleyer, J. Am. Chem. Soc., 108, 3951 (1986).
12.J. Bredt, H. Thouet and J. Schnitz, Justus Liebigs Ann. Chem., 437, 1 (1924).
13.J. Bredt, Ann. Acad. Sci. Fenn., 29A(2), 1 (1927).
14.G. Sziemies, Chimia, 35, 243 (1981).
15.J. Sandstrom,¨ Top. Stereochem., 14, 83 (1983).
16.P. M. Warner, Chem. Rev., 89, 1067 (1989).
17.W. T. Borden, Chem. Rev., 89, 1095 (1989).
18.W. Luef and R. Keese, Top. Stereochem., 20, 231 (1991).
19.A. Krebs, B. Kaletta, W.-U. Nickel, W. Ruger¨ and L. Tikwe, Tetrahedron, 42, 1693 (1986).
1278 |
Jan Sandstrom¨ |
20.P. R. Brooks, R. Bishop, D. C. Craig, M. L. Scudder and J. A. Counter, J. Org. Chem., 58, 5900 (1993).
21.U. Burkert, Tetrahedron, 37, 333 (1981).
22.G. Favini, M. Simonetta and R. Todeschini, J. Comput. Chem., 2, 149 (1981).
23.T. Pilati and M. Simonetta, J. Chem. Soc., Perkin Trans. 2, 1425 (1977).
24.A. Mugnoli and M. Simonetta, J. Chem. Soc., Perkin Trans. 2, 1831 (1976).
25.D. Lenoir, J. E. Gano and J. A. McTague, Tetrahedron Lett., 27, 5339 (1986).
26.J. E. Gano, B.-S. Park, G. Subramaniam, D. Lenoir and R. Gleiter, J. Org. Chem., 56, 4806 (1991).
27.H. Sakurai, Y. Nakadaira, H. Tobita, T. Ito, K. Toriumi and H. Ito, J. Am. Chem. Soc., 104, 300 (1982).
28.H. Sakurai, H. Tobita, Y. Nakadaira and C. Kabuto, J. Am. Chem. Soc., 104, 4288 (1982).
29.H. Sakurai, K. Ebata, C. Kabuto and Y. Nakadaira, Chem. Lett., 301 (1987).
30.H. Sakurai, K. Ebata, K. Sakamoto, Y. Nakadaira and C. Kabuto, Chem. Lett., 965 (1987).
31.I. Wennerbeck and J. Sandstrom,¨ Org. Magn. Reson., 4, 783 (1972).
32.J. Sandstrom¨ and U. Sjostrand,¨ Tetrahedron, 34, 371 (1978).
33.J. Sandstrom,¨ in The Chemistry of Enamines (Ed. Z. Rappoport), Wiley, Chichester, 1994, p. 405.
34.J. Sandstrom,¨ Dynamic NMR Spectroscopy, Academic Press, London and New York, 1982.
35.K. Mislow and M. Raban, Top. Stereochem., 1, 1 (1967).
36.J. Sandstrom,¨ U. Sjostrand¨ and I. Wennerbeck, J. Am. Chem. Soc., 99, 4526 (1977).
37.D. Adhikesvalu, N. U. Kamath and K. Venkatesan, Proc. Indian Acad. Sci. (Chem. Sci.), 92, 449 (1983).
38.J. Sandstrom,¨ unpublished.
39.S. Abrahamsson, G. Rehnberg, T. Liljefors and J. Sandstrom,¨ Acta Chem. Scand., B28, 1109 (1974).
40.U. Berg, R. Isaksson, J. Sandstrom,¨ A. Eiglsperger and A. Mannschreck, Tetrahedron Lett., 23, 4237 (1982).
41.Agha Z.-Q. Khan, R. Isaksson and J. Sandstrom,¨ J. Chem. Soc., Perkin Trans. 2, 491 (1987).
42.M. J. Janssen and J. Sandstrom,¨ Tetrahedron, 20, 2339 (1964).
43.J. Sandstrom,¨ K. Stenvall, N. Sen and K. Venkatesan, J. Chem. Soc., Perkin Trans. 2, 1939 (1985).
44.Agha Z.-Q. Khan and J. Sandstrom,¨ J. Chem. Soc., Perkin Trans. 2, 1575 (1994).
45.Agha Z.-Q. Khan, F.-L. Liao, J. Sandstrom¨ and S.-L. Wang, J. Chem. Soc., Perkin Trans. 2, 1569 (1994).
46.C. Dreier, L. Henriksen, S. Karlsson and J. Sandstrom,¨ Acta Chem. Scand., B32, 281 (1978).
47.K. Baum, S. S. Bigelow, N. V. Nguyen, T. G. Archibald, R. Gilardi, J. L. Flippen-Anderson and C. George, J. Org. Chem., 57, 235 (1992).
48.U. Berg and U. Sjostrand,¨ Org. Magn. Reson., 11, 555 (1978) and cited references therein.
49.T. Olsson and J. Sandstrom,¨ Acta Chem. Scand., B36, 23 (1982).
50.U. Sjostrand¨ and J. Sandstrom,¨ Tetrahedron, 34, 3305 (1978).
51.S. Karlsson and J. Sandstrom,¨ Acta Chem. Scand., B32, 141 (1978).
52.Agha Z.-Q. Khan, K.-E. Bergquist and J. Sandstrom,¨ Acta Chem. Scand., 44, 833 (1990).
53.Agha Z.-Q. Khan and J. Sandstrom,¨ J. Org. Chem., 56, 1902 (1991).
54.Agha Z.-Q. Khan, J. Sandstrom,¨ K.-E. Bergquist, C. Y. Cheng and S.-L. Wang, J. Org. Chem., 56, 4919 (1991).
55.Agha Z.-Q. Khan and J. Sandstrom,¨ Acta Chem. Scand., 44, 968 (1990).
56.F. P. Colonna, G. Distefano, J. Sandstrom¨ and U. Sjostrand,¨ J. Chem. Soc., Perkin Trans. 2, 279 (1978).
57.P. U. Biedermann, A. Levy, J. J. Stezowski and I. Agranat, Chirality, 7, 199 (1995).
58.N. P. Bailey and S. E. Hull, Acta Crystallogr., B34, 3289 (1978).
59.J. S. Lee and S. C. Nyburg, Acta Crystallogr., C41, 560 (1985).
60.D. Lenoir and P. Lemmen, Chem. Ber., 113, 3112 (1980).
61.M. Ballester, J. Castaner,˜ J. Riera, G. de la Fuente and M. Camps, J. Org. Chem., 50, 2287 (1985).
62.R. Kuhn, H. Zahn and K. L. Scholler, Justus Liebigs Ann. Chem., 582, 196 (1953).
63.I. R. Gault, W. D. Ollis and I. O. Sutherland, J. Chem. Soc., Chem. Commun., 269 (1970).
64.L. L. Yeung, Y. C. Yip and T.-Y. Luh, J. Chem. Soc., Chem. Commun., 981 (1987).
65.D. K. P. Ng and T.-Y. Luh, Tetrahedron Lett., 29, 5131 (1988).
21. Strained olefins |
1279 |
66.X.-j. Wang and T.-Y. Luh, J. Org. Chem., 54, 263 (1989).
67.C. Yip, X.-j. Wang, D. K. P. Ng, T. C. W. Mak, P. Chiang and T.-Y. Luh, J. Org. Chem., 55, 1881 (1990).
68.U. Grieser and K. Hafner, Tetrahedron Lett., 35, 7759 (1994).
69.P. U. Biedermann, A. Levy, M. R. Suissa, J. J. Stezowski and I. Agranat, Enantiomer, 1, 75 (1996).
70.E. Harnik and G. M. J. Schmidt, J. Chem Soc., 3295 (1954).
71. P. A. Apgar and |
E. Wasserman, unpublished; more |
accurate |
results quoted in G. Shoham, |
|
S. Cohen, R. M. Suissa and |
I. Agranat, Molecular |
Structure |
and Biological Activity, IUCr |
|
Crystallographic |
Symposia 2 |
(Eds. J. J. Stezowskil, |
J.-L. Huang and M.-C. Shao), Oxford |
University Press, 1988, p. 290.
72.J. F. D. Mills and S. C. Nyburg, J. Chem. Soc., 308 (1963).
73.I. Agranat and Y. Tapuhi, J. Am. Chem. Soc., 98, 615 (1976).
74.I. Agranat and Y. Tapuhi, Nouveau J. Chim., 1, 361 (1977).
75.I. Agranat and Y. Tapuhi, J. Am. Chem. Soc., 100, 5604 (1978).
76.I. Agranat and Y. Tapuhi, J. Am. Chem. Soc., 101, 665 (1979).
77.I. Agranat, Y. Tapuhi and J. Y. Lallemand, Nouveau J. Chim., 3, 59 (1979).
78.I. Agranat and Y. Tapuhi, J. Org. Chem., 44, 1941 (1979).
79.Y. Tapuhi, O. Kalisky and I. Agranat, J. Org. Chem., 44, 1949 (1979).
80.A. Warshel and M. Karplus, J. Am. Chem. Soc., 96, 5677 (1974).
81.A. Korenstein, K. A. Muszkat and G. Seger, J. Chem. Soc., Perkin Trans. 2, 1536 (1976).
82.A. Korenstein, K. A. Muszkat and E. Fischer, Chem. Phys. Lett., 36, 509 (1975).
83.E. Fischer, Reviews of Chemical Intermediates, 5, 393 (1984).
84.D. L. Fanselow and H. G. Drickamer, J. Chem. Phys., 61, 4567 (1974).
85.B. L. Feringa, W. F. Jager and B. de Lange, Tetrahedron Lett., 33, 2887 (1992).
86.Y. Okamoto, S. Honda, I. Okamoto, H. Yuki, S. Murata, R. Noyori and H. Takaya, J. Am. Chem. Soc., 103, 6971 (1981).
87.B. L. Feringa, W. F. Jager, B. de Lange and E. W. Meyer, J. Am. Chem. Soc., 113, 5468 (1991).
88.B. L. Feringa, W. F. Jager and B. de Lange, J. Chem. Soc., Chem. Commun., 288 (1992).
89.K. S. Dichmann, S. C. Nyburg, F. H. Pickard and J. A. Potworowski, Acta Crystallogr., B30, 27 (1974).
90.R. L. Viavattene, F. D. Greene, L. D. Cheung, R. Majeste and L. M. Trefonas, J. Am. Chem. Soc., 96, 4342 (1974).
91.R. N. Butler, A. M. Gillan, P. McArdle and D. Cunningham, J. Chem. Soc., Chem. Commun., 1016 (1987).
92.R. Bonneau, J. Joussot-Dubien, L. Salem and A. J. Yarwood, J. Am. Chem. Soc., 98, 4329 (1976).
93.Y. Inoue, T. Veroka, T. Kuroda and T. Hakushi, J. Chem. Soc., Chem. Commun., 1031 (1981).
94.R. Bonneau, J. Joussot-Dubien and A. J. Yarwood, Tetrahedron Lett., 235 (1977).
95.N. L. Allinger and J. T. Sprague, J. Am. Chem. Soc., 94, 5734 (1972).
96.M. Traetteberg, Acta Chem. Scand., B29, 29 (1975).
97.O. Ermer and S. Lifson, J. Am. Chem. Soc., 95, 4121 (1973).
98.M. Traetteberg, P. Backen and A. J. Almenningen, J. Mol. Struct., 74, 321 (1981).
99.H. Tsuneishi, Y. Inoue, T. Hakushi and A. Tai, J. Chem. Soc., Perkin Trans. 2, 457 (1993).
100.A. C. Cope, C. R. Ganellin and H. W. Johnson, Jr., J. Am. Chem. Soc., 84, 3191 (1962).
101.A. C. Cope and B. A. Pawson, J. Am. Chem. Soc., 87, 3649 (1965).
102.A. C. Cope, R. A. Pike and C. F. Spencer, J. Am. Chem. Soc., 75, 3212 (1953).
103.Y. Inoue, S. Takamuku and H. Sakurai, Synthesis, 111 (1977).
104.Y. Inoue, S. Takamuku and H. Sakurai, J. Phys. Chem., 81, 7 (1977).
105.Y. Inoue, N. Yamasaki, T. Yokoyama and A. Tai, J. Am. Chem. Soc., 111, 6480 (1989).
106.Y. Inoue, N. Yamasaki, T. Yokoyama and A. Tai, J. Org. Chem., 57, 1332 (1992).
107.Y. Inoue, N. Yamasaki, T. Yokoyama and A. Tai, J. Org. Chem., 58, 1011 (1993).
108.H. Tsuneishi, T. Hakushi, A. Tai and Y. Inoue, J. Chem. Soc., Perkin Trans. 2, 2057 (1995).
109.A. C. Cope, J. K. Hecht, H. W. Johnson, Jr., H. Keller and H. J. S. Winkler, J. Am. Chem. Soc., 88, 761 (1966).
110.H.-D. Martin, M. Kunze, H.-D. Beckhaus, R. Walsh and R. Gleiter, Tetrahedron Lett., 3069 (1979).
111.A. C. Cope and C. Bumgardner, J. Am. Chem. Soc., 78, 2812 (1956).
112.R. S. H. Liu, J. Am. Chem. Soc., 89, 112 (1967).
1280 |
Jan Sandstrom¨ |
113.R. Isaksson, J. Roschester, J. Sandstrom¨ and L. Wistrand, J. Am. Chem. Soc., 107, 4074 (1985).
114.Y. Inoue, private communication.
115.K. M. Shumate, P. N. Neuman and G. J. Fonken, J. Am. Chem. Soc., 87, 3996 (1965).
116.J. J. Bromfield and J. S. McConaghy, Jr., Tetrahedron Lett., 3723 (1969).
117.T. H. Bouman and A. E. Hansen, J. Am. Chem. Soc., 107, 4828 (1985).
118.T. H. Bouman and A. E. Hansen, Croat. Chim. Acta, 62, 227 (1989).
119.K. L. Bak, A. E. Hansen, K. Ruud, T. Helgaker, J. Olsen and P. Jørgensen, Theor. Chim. Acta, 90, 441 (1995).
120.For a review, see E. Charney, The Molecular basis of Optical Activity, Wiley, New York, 1979, p. 217.
121.C. A. Cope, K. Banholzer, H. Keller, B. A. Pawson, J. J: Whang and H. J. S. Winkler, J. Am. Chem. Soc., 87, 3644 (1965).
122.I. Kolossvary´ and W. C. Guida, J. Mol. Struct. (Theochem), 308, 91 (1994).
123.J. A. Marshall, Acc. Chem. Res., 13, 213 (1980).
124.V. Cere,´ C. Paolucci, S. Pollicino, E. Sandri and A. Fava, J. Org. Chem., 46, 486 (1981).
125.J. A. Marshall and H. Faubl, J. Am. Chem. Soc., 89, 5965 (1967).
126.J. R. Wiseman, J. Am. Chem. Soc., 89, 5966 (1967).
127.J. R. Wiseman and W. A. Pletcher, J. Am. Chem. Soc., 92, 956 (1970).
128.S. F. Sellers, T. C. Klebach, F. Hollowood, M. Jones, Jr. and P. v. R. Schleyer, J. Am. Chem. Soc., 104, 5492 (1982).
129.M. S. Gudipati, J. R. Radziszewski, P. Kaszynski and J. Michl, J. Org. Chem., 58, 3668 (1993).
130.T. G. Lease and K. J. Shea, J. Am. Chem. Soc., 115, 2248 (1993).
131.G. E. Renzoni, T.-K. Yin and W. T. Borden, J. Am. Chem. Soc., 108, 7121 (1986).
132.R. Greenhouse, W. T. Borden, K. Hirotsu and J. Clardy, J. Am. Chem. Soc., 99, 1664 (1977).
133.T.-K. Yin, F. Miyake, G. E. Renzoni, W. T. Borden, J. G. Radziszewski and J. Michl, J. Am. Chem. Soc., 108, 3544 (1986).
134.D. A. Hrovat, F. Miyake, G. Trammell, K. E. Gilbert, J. Mitchell, J. Clardy and W. T. Borden,
J. Am. Chem. Soc., 109, 5524 (1987).
135.D. A. Hrovat and W. T. Borden, J. Am. Chem. Soc., 110, 4710 (1988).
136.P. E. Eaton and M. J. Maggini, J. Am. Chem. Soc., 110, 7230 (1988).
137.L. A. Paquette, A. G. Schaefer and J. F. Blount, J. Am. Chem. Soc., 105, 3642 (1983).
138.K. N. Houk, N. G. Rondan, F. K. Brown, W. L. Jorgensen, J. D. Madura and D. C. Spellmeyer, J. Am. Chem. Soc., 105, 5980 (1983) and references cited therein.
139.W. H. Watson, J. Galloy, D. A. Grossie, P. D. Bartlett and G. L. Combs, Acta Crystallogr., C40, 1050 (1984).
140.L. A. Paquette and C.-C. Shen, J. Am. Chem. Soc., 112, 1159 (1990).
141.H. Kunzer,¨ E. Litterst, R. Gleiter and L. A. Paquette, J. Org. Chem., 52, 4740 (1987).
142.K. B. Wiberg, M. G. Matturro, P. J. Okarma and M. E. Jason, J. Am. Chem. Soc., 106, 2194 (1984).
143.J. E. McMurry, G. J. Haley, J. R. Matz, J. C. Clardy, G. Van Duyne, R. Gleiter, W. Schafer¨ and D. H. White, J. Am. Chem. Soc., 106, 5018 (1984).
144.J. P. Kiplinger, F. R. Tollens, A. G. Marshall, T. Kobayashi, D. R. Lagerwall, L. A. Paquette and J. E. Bartmess, J. Am. Chem. Soc., 111, 6914 (1989).
145.J.-P. Melder, R. Pinkos, H. Fritz and H. Prinzbach, Angew. Chem., Int. Ed. Engl., 28, 305 (1989).
146.D. H. R. Barton, M. R. Britten-Kelly and F. S. Guziec, Jr., J. Chem. Soc., Perkin Trans. 1, 2078 (1976) and references cited therein.
147.J. E. McMurry, M. P. Fleming, K. L. Kees and L. R. Krepski, J. Org. Chem., 43, 3255 (1978).