
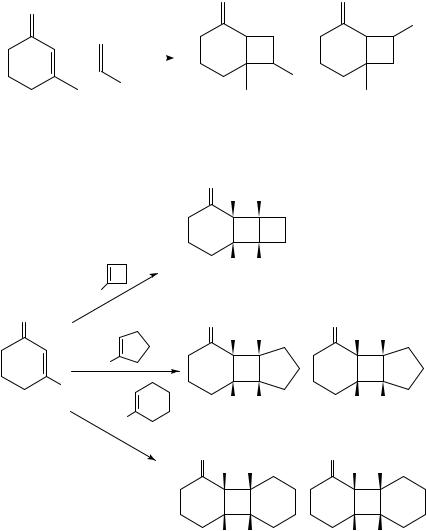
|
13. Photochemistry of compounds containing CDC double bonds |
661 |
|||||
|
|
|
|
O |
|
O |
|
O |
|
|
|
|
|
|
|
|
|
|
|
|
|
|
R |
|
+ |
|
hν |
|
|
+ |
|
|
|
|
|
R |
|
||
|
R |
|
|
|
|
||
|
|
|
|
|
|
||
|
|
|
|
HT |
|
HH |
|
(78) |
(79) |
|
(80) |
|
(81) |
|
|
|
(a) R= OEt |
100 |
: |
0 |
|
||
|
(b) R= CN |
16 |
: |
84 |
|
||
|
|
|
|
SCHEME 18 |
|
|
|
|
|
|
|
O |
|
|
|
|
|
|
|
H |
CO2 Me |
|
|
|
|
R |
H |
|
|
|
|
|
R= Me, CO2 Me >95 (55−60% yield) |
|
|||
|
MeO2 C |
|
|
|
|
|
O |
hν |
|
|
|
|
|
|
O |
|
|
O |
|
|
|
|
|
|
|
||
|
|
H |
CO2 Me |
|
H |
H |
|
MeO2 C |
|
|
+ |
|
|
|
hν |
|
|
|
|
|
|
|
|
|
|
|
|
|
R |
|
|
|
|
|
|
|
R |
H |
|
R |
CO2 Me |
(78) R= Me |
MeO2 C |
R= Me |
50 |
: |
50 (35% total yield) |
|
(82) R= CO2 Me |
R= CO2 Me |
60 |
: |
40 (22% total yield) |
||
|
hν |
|
|
|
|
|
|
|
O |
|
|
O |
|
|
|
H |
CO2 Me |
|
H |
H |
|
|
|
|
+ |
|
|
|
|
R |
H |
|
R |
CO2 Me |
|
|
R= Me |
11 |
: |
89 (49% total yield) |
|
|
|
R= CO2 Me |
<5 |
: |
>95% (87% total yield) |
SCHEME 19
However, the groups of Tada64 and of Lange65 have obtained photoadditions with opposite regioselectivity rationalized by the oriented -complex. Lange and coworkers65 have systematically examined the effect of the ring size in the alkene on the regioselectivity of its photoaddition to cyclohexenones 78 and 82 (Scheme 19). The reversal of regioselectivity with increasing ring size of the alkene is not consistent with the oriented-complex model.
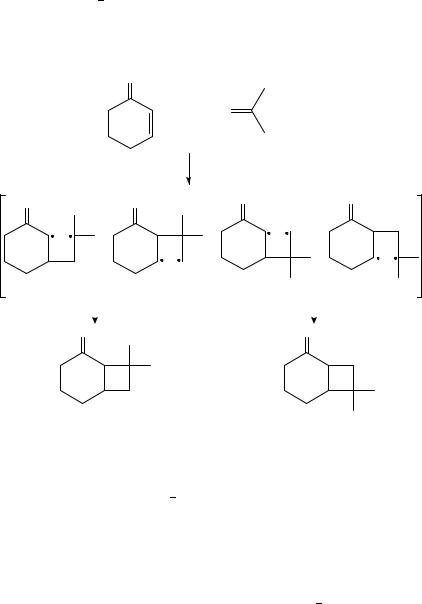
662 |
Nizar Haddad |
Alternatively, Bauslaugh66 proposed that the regiochemistry in [2 C 2] photocycloadditions of enones could be explained without invoking exciplexes, by considering the partitioning of 1,4-diradicals between cyclization and fragmentation pathways. For example, in the photoaddition of cyclohexenone to 2-methylpropene, four possible diradical intermediates (83 86) could be considered. Based on the relative diradical stabilization, he proposed that 84 was unlikely to be formed and 83 was formed by the fastest rate; however, it most likely undergoes efficient fragmentation to the starting materials due to its relative stability. Thus cyclization occurred preferentially from diradicals 85 and 86 affording the corresponding H,T (88) photoproduct (Scheme 20).
O
+
hν
O O O O
(83) |
(84) |
(85) |
(86) |
||
|
|
|
|
|
|
|
O |
|
O |
HH |
HT |
(87) |
(88) |
SCHEME 20
Recent studies by Schuster and collaborators67,54, based on nanosecond laser flash techniques, revealed important conclusions, including: (a). The enone excited state responsible for the photocycloaddition is the Ł which possesses different polarization than the n- Ł state, considered in rationalizing the effect of the oriented -complex. (b) Direct measurement of the reactivity scale of alkenes measured by nanosecond flash photolysis provided different results from those obtained with no consideration of the diradical fragmentation to starting materials.
Further experimental support for the effect of the fragmentation of the 1,4-diradical intermediates on the regioselectivity of the photocycloaddition was recently reported by Weedon and coworkers68,69, who obtained different H,H/H,T ratios between the photocycloproducts [H,H (93) and H,T (94)] and the trapped products 95 98, upon irradiation in the absence of H2Se in the former case and complete trapping of the diradical intermediates in the presence of H2Se in the latter case (Scheme 21).

13. Photochemistry of compounds containing CDC double bonds |
663 |
SCHEME 21
Schuster’s and Weedon’s results support Bauslaugh’s proposed mechanism that emphasizes the effect of the ratio between cyclization and the alternative fragmentation pathway of the diradical intermediate, on the regioselectivity in the intermolecular photocycloadditions, and propose not to consider the oriented -complex (exciplex) as an intermediate in the mechanistic pathway of the [2 C 2] photocycloaddition of enones to alkenes.
Recent theoretical studies on the regioselectivity of photocycloadditions of triplet cyclohexenones to alkenes have been carried out by Houk and coworkers70, using ab initio theory implemented in the GAUSSIAN programs to locate the transition states for the formation of regioisomeric biradicals and to investigate the influence of substituents on the rates. UMP4(SDTQ)/6-31GŁ single-point calculations on the planar and twisted conformers of the n Ł and Ł triplet states of acroleine, as a model for the enone

664 |
Nizar Haddad |
TABLE 3. |
PMP3/6-31GŁ //UHF/3-21G relative energies (kcal mol 1) for addition of Ł |
triplet acrolein to substituted alkenes. Reprinted with permission from Ref. 70. Copyright (1995) American Chemical Society
Alkene |
Calcd (kcal mol 1) |
Expt (kcal mol 1) |
Expt |
||||
|
EŁ D E ˛ E ˇ |
GŁ |
HH/HT ratio |
||||
Acrylonitrile |
0.9 |
> 1.1 |
>5.7:1 |
||||
Allene |
0.6 |
> 2.0 |
ca 100:0 |
||||
Ethylene |
0.3 |
|
|
|
|
|
|
0.5 |
|
|
|
||||
Isobutene |
0.5 |
1:3.3 |
|||||
Methyl vinyl ether |
1.7 |
< 2.0 |
ca 0:100 |
chromophore, show that the twisted Ł triplet is the global minimum, the planar n Ł triplet is higher by 9.9 kcal mol 1, the planar Ł triplet by 13.2 kcal mol 1 and the twisted n Ł triplet by 23.4 kcal mol 1. The calculated charges of the planar n Ł triplet show that the ˇ-carbon, as proposed by Corey, bears more negative charge than the ˛- carbon. However, in accord with experimental data, the lowest energy is the Ł triplet and possesses opposite polarity to that found in the planar n Ł triplet.
Houck and coworkers postulate that the origin of the regioselectivity is at the biradicalforming step and directly affected by the polarity of the alkene. The ˇ-carbon, considered as nucleophilic, adds rapidly to the less substituted side of the electron-deficient alkene, whereas a position considered as an ˛-acyl radical (more electrophilic than an alkyl radical) adds rapidly to the less substituted side of electron-rich alkenes. The calculated relative energies for the addition of Ł triplet acrolein to different substituted alkenes at the first bond-forming step (Table 3) are found to be in good agreement with experimental values determined in the photoaddition of cyclohexenone to the related alkene.
Prediction of the regioselectivity in the intermolecular photocycloaddition of enones to alkenes following this method provides similar results to those rationalized by the oriented-complex. However, it is in contrast with Weedon’s previously discussed trapping results which indicate no selectivity in the first bond formation at the ˛- or ˇ-carbon positions in cyclic enones.
2. Intramolecular photocycloadditions
The regioselectivity in the intramolecular photocycloadditions can be controlled to some extent due to geometrical constraints. The effect of substituents and chain length between the reacting alkenes has been extensively investigated in the photoaddition of alkenes to enones and well documented in several excellent reviews54 58. In some cases, the regioselectivity can be predicted based on the empirical ‘rule of five’71, which postulates that the first bond formation will favor closure to a five-membered ring, unless structural or conformational factors interfere. If a five-membered ring cannot be formed (short or long tethered), a six-membered homolog is favored.
Wolff and Agosta72 have investigated the regioselectivity in a large number of acyclic and cyclic enones to tethered alkenes of types 99 102. These compounds generally form cross 1,5-photoproducts. Two factors were found to play an important role in the regioselectivity toward 1,6-parallel addition: (a) placement of a substituent on the C-5 position of the alkenyl chain and (b) incorporation of the conjugated double bond in a fiveor six-membered ring. Selected examples are presented in Scheme 22.
Homologation of the alkenyl side chain was extensively examined in compounds of type 103 and 105. In all cases, only straight and all cis-fused photoproducts were obtained (Scheme 23).

|
13. Photochemistry of compounds containing CDC double bonds |
665 |
|||||||||||||||
|
|
hν |
|
|
|
|
|
|
|
|
|
|
|
|
hν |
|
O |
|
O |
|
|
|
O |
|
|
|
|
|
|
O |
|
|
|
||
|
|
|
|
|
|
|
|
|
|
|
|
|
|
||||
|
|
|
|
|
|
|
|
|
|
|
|
|
|
|
|||
|
(99) |
|
|
|
|
|
88% |
|
|
|
(100) |
|
12% |
|
|||
|
|
|
|
|
|
|
(crossed) |
|
|
|
(crossed) |
|
|||||
|
O |
|
|
|
|
|
|
|
( )n |
|
R |
|
O |
|
|||
|
|
|
|
|
|
|
|
|
|
|
|
||||||
|
R |
|
|
hν |
|
R |
O + |
|
+ |
|
|
||||||
|
|
|
|
|
|
|
|
|
|
|
|
|
|
|
O |
|
|
|
|
|
|
|
|
|
|
|
|
|
|
|
|
|
|
|
|
( |
) |
|
|
|
|
|
|
|
|
|
|
|
|
|
( |
)n |
|
|
n |
|
|
|
|
|
|
|
|
|
|
( |
)n |
|
|
|
|
|
(101) |
|
|
|
|
|
|
|
|
|
|
|
|
|
|
||
|
|
|
|
|
|
|
|
(straight) |
|
(crossed) |
|
|
|
||||
|
n =1, 2 |
|
|
|
|
56% |
|
|
|
|
7% |
|
8% |
|
|||
|
(a) n = 1, R= H |
|
|
|
: |
: |
|
||||||||||
|
(b) n = 1, R= Me |
84% |
|
|
|
: |
0% |
: |
4% |
|
|||||||
|
(c) n = 2, R= H |
20% |
|
|
|
: |
49% |
: |
2% |
|
|||||||
|
(d) n = 2, R= Me |
76% |
|
|
|
: |
11% |
: |
2% |
|
|||||||
|
O |
|
|
|
|
|
|
|
|
|
|
|
|
|
|
|
|
|
|
|
|
|
|
hν |
|
|
|
|
|
|
Other isomers obtained |
|
|
||
( |
)n |
|
|
|
|
|
|
|
|
|
|
in less than 6% yield. |
|
|
|||
|
|
|
|
( |
)n |
|
|
|
|
||||||||
|
|
|
|
|
|
|
|
|
|
|
|||||||
|
|
|
|
|
|
|
|
O |
|
|
|
|
|
||||
|
(102) |
|
|
|
|
|
|
|
ma jor (50-70%) |
|
|
|
|
||||
|
n = 1, 2 |
|
|
|
|
|
|
|
|
|
|
|
|
|
|
|
|
|
|
|
|
|
|
|
|
|
|
|
SCHEME 22 |
|
|
|
|
||
|
O |
|
|
|
|
|
|
|
|
|
|
O |
|
|
|
|
|
|
|
|
|
|
|
|
|
|
|
|
|
|
|
|
|
||
|
|
|
|
|
|
|
|
|
|
hν |
|
|
|
|
|
|
|
|
( )n |
|
|
|
|
|
R |
( )n |
|
|
|
|
|||||
|
|
|
|
|
|
|
|
|
|
|
|
|
R |
|
|
||
|
|
|
n = 1, 2 |
|
|
|
|
|
|
straight |
|
|
|
|
|||
|
(103) |
|
|
|
|
|
|
(104) |
|
|
|
|
|||||
|
R = H, Me, i-Pr, t-Bu |
|
|
|
|
||||||||||||
|
O |
|
|
|
|
|
|
|
|
|
|
|
|
|
|
|
|
|
|
|
|
|
|
|
|
|
|
|
O |
|
|
O |
|
|
|
|
|
|
|
|
|
|
|
|
hν |
|
|
|
|
|
|||
|
( )n |
|
|
|
|
|
|
|
|
|
|
|
|
|
R |
|
|
|
|
|
|
|
R |
|
R |
|
|
|
|||||||
|
|
|
|
|
|
|
|
|
|
|
|||||||
|
|
|
|
|
|
|
( |
)n |
|
( )n |
|
|
|||||
|
|
|
|
|
|
|
|
|
|
|
|
|
straight |
|
crossed |
|
|
|
(105) |
n = 1, 2 |
(106) |
|
(107) |
|
|||||||||||
|
|
|
|
|
(Sole or major) |
(minor obtained with |
|
||||||||||
|
|
|
|
|
R = H, Me, t-Bu |
|
|
n = 1, R = Me, t-Bu) |
|
SCHEME 23

R |
R |
O |
O |
O |
||||
O |
|
|
R |
R |
R |
|||
|
|
|
R |
|
||||
|
|
hν |
|
+ |
|
+ |
|
|
|
|
|
|
|
|
|||
|
|
|
|
|
|
R |
||
|
|
|
|
|||||
|
|
|
|
|
|
|
|
|
|
( )n |
|
|
|
|
|
R ( )n |
|
|
|
|
|
|
|
|
||
|
|
|
( )n |
( |
) |
|
|
|
|
|
|
|
|
|
n |
||
|
|
|
|
|
straight |
crossed |
||
|
|
|
straight |
(twisted approach) |
||||
(a) n = 1, R= H or CH2 (allene) |
100 |
|
0 |
0 (>95%, Reference 73) |
||||
(b) n = 2, R= H |
|
|
66 |
|
? 17 |
? 17 (>90%, Reference 74) |
||
(c) n = 2, R= CH2 (allene) |
85 |
|
0 |
15 (>95%, Reference 73) |
SCHEME 24
O |
|
|
|
O |
|
O |
|
|
O |
|
|
|
|
|
|
|
|
|
|
|
|
X1 |
X4 |
X1 |
|
X1 |
|
1 |
|
|
||
|
|
hν |
|
|
|
|
|
X |
|
X4 |
|
( )n |
|
+ |
|
|
+ |
|
R |
||
|
|
|
|
|
|
|
||||
X2 |
X2 |
X4 |
X2 |
X4 |
|
|
R ( )n |
|||
X3 |
|
2 |
||||||||
|
|
|
|
|
X3 |
X3 |
|
|
X |
X3 |
|
|
|
|
|
( )n |
|
|
|||
|
|
|
|
|
( )n |
|
|
|
|
|
|
|
|
|
|
straight |
straight |
|
|
|
crossed |
|
|
|
|
|
|
(twisted approach) |
|
|
|
|
(a) n = 1or 2, X1 = X2 = 0, X3 = X4 = H |
|
100 |
0 |
|
2 |
(>73%, Reference 75) |
||||
(b) n = 2, X1 = X2 = X4 = H, X3 = O |
|
100 (straight photo products) |
|
0 |
(60%, Reference 76) |
|||||
(c) n = 2, X1 = X2 = X3 = H, X4 = O |
|
|
|
|
|
(>95%, Reference 77) |
||||
(d) n = 2, X1 = X3 = X4 = H, X2 = O |
|
80 |
20 |
|
0 |
(80%, Reference 78) |
SCHEME 25
666

13. Photochemistry of compounds containing CDC double bonds |
667 |
Further homologation of the alkenyl side chain affects the regioselectivity, as could be seen in the examples in Scheme 24.
Interestingly, incorporation of an oxygen heteroatom at any allylic position in these systems strongly affects the regioselectivity of the photocycloaddition and in all cases, summarized in Scheme 25, straight products were obtained as the sole products.
The regioselectivity of the photocycloaddition is strongly affected by the relative stability of the possible diradical intermediates and the relative rate of cyclization vs cleavage of the corresponding diradical intermediate to the starting material. The order of the first bond formation of the alkene to the ˛-carbon or the ˇ-carbon of the enone chromophore plays a major role in the regioselectivity, in cases where the fragmentation of the diradical intermediate to the starting compounds is a neglectable process.
Becker and coworkers79 have systematically investigated the photoaddition of substituted enones 108. Irradiations of Z- or E-108 afforded a similar ratio of endo (110) to exo (111) isomers with minor geometrical isomerization of the alkene in the starting material 108 during the irradiation (less than 10%). Based on these results, they reached the conclusion that first bond formation in these compounds follows the ‘rule of five’ and takes place exclusively via diradical 109. Different ratios were obtained in the photoaddition of the corresponding cyclopentenone systems80. However, the similar endo/exo ratio obtained in the photocycloaddition of Z and E alkenes is in full agreement with the diradical intermediate 109 in which the obtained ratio is affected by steric effects in the cyclization step of the diradical to the corresponding [2 C 2] photoproducts (Scheme 26).
R1 |
|
|
|
|
|
|
|
|
|
|
|
O |
|
|
|
O |
R1 |
|
|
|
|
|
|
|
|
|
|
|
|
|
|
||||
|
|
|
|
|
|
|
|
|
|
|
|
2 |
|
hν |
|
|
• |
• |
|
|
|
|
|
R |
|
|
|
R2 |
|
|
|
|
|
||
( )n |
|
|
|
( )n |
|
|
|
|
|
|
|
(108) |
|
|
|
|
|
|
|
|
|
|
|
|
|
|
(109) |
|
|
|
|
|
|
||
|
|
|
|
|
|
|
|
|
|
||
|
|
|
|
|
O |
H |
|
R1 |
|
O |
R1 |
|
|
|
|
|
|
|
|
|
|
H |
|
|
|
|
|
|
|
|
|
R2 |
+ |
|
R2 |
|
|
|
|
|
|
|
|
|
|
|
|
|
|
|
|
|
( )n |
|
|
|
|
( )n |
|
|
|
|
|
|
|
(110) |
|
|
|
|
(111) |
(a) R1 = Z-Me, E-Me, E-i-Pr, R2 = H, n = 2; |
|
|
endo |
1 |
: |
1 |
exo |
||||
(b) R1 = R2 = D, n = 2; |
|
|
|
|
endo |
1 |
: |
1 |
exo |
||
(c) R1 = Z-Me, R2 = H, n = 1; |
|
|
|
|
endo |
1 |
: |
2 |
exo |
||
(d) R1 = E-Me, R2 = H , n = 1; |
|
|
|
|
endo |
1 |
: |
2 |
exo |
||
(e) R1 = Z-t-Bu, R2 = H, n = 1; |
|
|
|
|
endo |
1.1 |
: |
1 |
exo |
||
(f) R1 = E-t-Bu, R2 = H, n = 1: |
|
|
|
|
endo |
1.1 |
: |
1 |
exo |
SCHEME 26
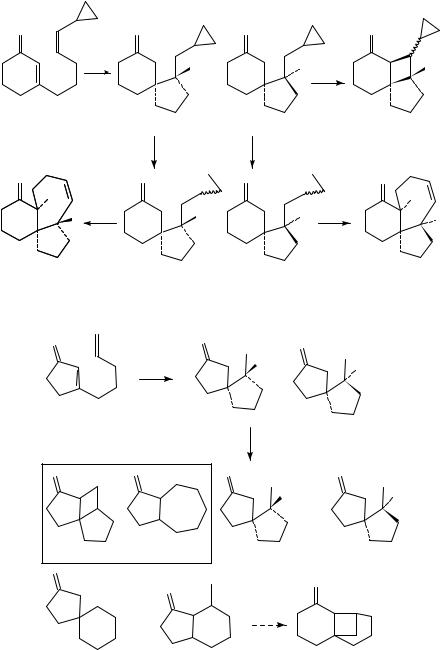
668 |
|
|
Nizar Haddad |
|
|
|
|
|
O |
O |
|
|
O |
|
|
|
O |
hν |
• |
• |
H |
|
• |
• |
H |
H |
|
+ |
|
||||||
|
|
|
|
|
|
|
[2+2] |
|
(112) |
(113) |
|
(114) |
|
|
|
(115) |
|
O |
O |
|
• |
O |
|
|
• |
O |
H |
|
|
|
|
|
|
|
H |
H |
|
• |
H |
+ |
• |
|
H |
H |
|
|
|
|
|
|
|||
|
|
|
|
|
|
|
|
|
(118) |
|
(116) |
|
|
(117) |
|
(119) |
|
Major |
|
|
|
|
|
|
|
Minor |
SCHEME 27
O |
|
|
|
|
|
|
O |
|
|
O |
|
|
|
|
|
|
|
|
|
|
|
|
• |
|
|
|
|
|
|
|
|
|
|
|
|
• |
H |
• |
• |
H |
|
|
|
|
|
hν |
|
|
|
+ |
|||||
|
|
|
|
|
|
|
|
|
|
||||
|
|
|
|
|
|
|
|
|
|
|
|||
( |
)n |
|
|
|
|
|
( |
)n |
|
( |
)n |
|
|
(120) |
n =1, 2 |
|
|
|
|
|
(121) |
|
(122) |
|
|
||
|
|
|
|
|
|
|
|
|
|
H2 Se |
|
|
|
O |
|
|
O |
|
|
|
• |
O |
|
|
|
O |
|
|
|
|
|
|
|
|
|
|
H |
|
|
H |
|
|
|
|
|
|
|
|
|
|
|
+ |
|
||
|
|
|
|
|
|
|
|
|
|
|
|
|
|
( |
) |
|
( |
)n |
• |
|
|
( |
) |
|
|
( |
) |
|
n |
|
|
|
|
|
|
|
n |
|
|
|
n |
|
(125) |
|
|
(126) |
|
|
|
(123) |
|
|
(124) |
||
O |
|
|
|
|
|
|
|
|
|
|
|
|
|
|
|
• |
|
|
O |
|
|
• |
|
|
O |
|
|
|
|
|
|
|
|
|
|
|
|
|
|
||
( |
)n |
• |
+ |
|
|
|
|
|
|
|
|
|
|
|
|
|
|
|
|
|
|
|
|
|
|
||
|
|
|
|
|
( |
)n |
• |
|
|
|
|
|
|
|
(127) |
|
|
|
|
(128) |
|
|
|
(129) |
|
||
|
|
|
|
|
|
|
SCHEME 28 |
|
|
|
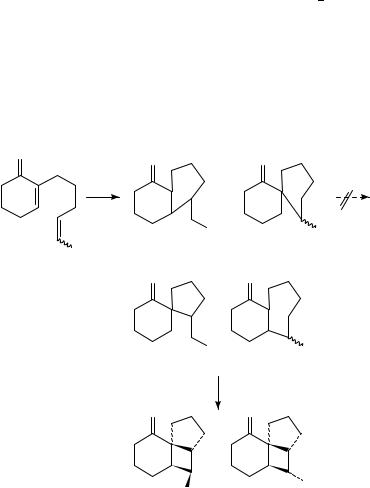
13. Photochemistry of compounds containing CDC double bonds |
669 |
Furthermore, Becker and coworkers81 have succeeded in trapping the 1,4-diradical intermediates 113 and 114 in the irradiation of compound 112 providing direct evidence for these intermediates and support for the regioselectivity in the first bond formation (Scheme 27).
Further support was recently obtained by Maradyn and Weedon82 on complete trapping of diradicals 121 and 122 with hydrogen selenide (H2Se) affording products 123 and 124. The absence of cross-products 129, of [2 C 2] photoproduct 125 and of other trapped products, precludes the formation of diradical intermediates 126 128 and provides further evidence for the selectivity in the formation of the first bond as predicted by the ‘rule of five’ (Scheme 28).
Irradiation of cyclohexenones 130 possessing the alkenyl side chain at the ˛-carbon have been systematically examined by Becker and coworkers79,80 and found to afford different ratios of endo (135) and exo (136) photoproducts. It is generally accepted that in these systems diradicals 133 and 134 could be formed; however, the absence of cross-products clearly rules out diradical intermediates 131 and 132 (Scheme 29).
Trapping experiments on cyclic enones which possess the alkenyl side chain at the ˛-carbon were examined by Becker and coworkers83 via the irradiation of compounds
O |
O |
|
O |
|
|
|
|
||
hν |
• |
+ |
• |
Cross-products |
|
|
|||
|
|
• |
• |
|
|
|
|
|
|
|
|
R |
|
R |
R |
(131) |
|
(132) |
|
(130) |
|
|
||
|
O |
|
O |
|
+ |
• |
+ |
• • |
|
|
• |
|
|
|
|
|
R |
|
R |
(133)(134)
O O
+
R
R
|
(135) |
(136) |
(a) R= Z-Me |
5.8 |
1 |
(b) R= E-Me |
35 |
1 |
(c) R= Z-i-Pr |
1.4 |
1 |
(d) R= E-i-Pr |
3.8 |
1 |
SCHEME 29

670 |
Nizar Haddad |
137. Formation of trapping product 140 in over 54% yield provides strong support for preferential formation of the first bond at the ˛-carbon, as could be predicted by the ‘rule of five’. Higher trapping yield (65%) was obtained in the irradiation of 137 (R D t-Bu) at 50 °C. Formation of the Z-trapped product 141 and the E/Z ratio of the trapped products 140/141 (52:13 respectively) could be attributed to an equilibrium between the cyclopropylcarbinyl radical 138 and its corresponding homoallyl radical. However, further studies are required to verify this assumption (Scheme 30).
O |
O |
O |
hν |
|
+ |
R |
R |
R |
(137) |
(138) |
(139) |
R= H, t-Bu |
|
|
O |
O |
O |
+ |
|
+ |
R |
R |
R |
(140) |
(141) |
(142) |
SCHEME 30
The steric effect of R-substituents at C-4 in the cyclohexenone was found to affect the diastereofacial selectivity of the photocycloaddition84.
Winkler and Shao85 have examined the first bond formation in the photoaddition of labeled dioxinones 143 and 146. Irradiation of 143 afforded an epimeric mixture of the more strained photoproducts 145, while irradiation of 146 gave 148 as a single product. These results are consistent with diradicals 144 and 147 as the intermediates in the photoaddition of 143 and 146, respectively, pointing out that the first bond formation in both cases takes place exclusively at the ˇ-carbon of the dioxinone chromophore (Scheme 31).
The role of the dioxinone chromophore on the selectivity in the first bond formation, was examined by comparison to the enone analogs 149 and 151, lacking the ˇ-oxygen found in the dioxinone chromophore. Irradiation of 149 and 151 afforded only the trans-fused photoproducts 150 and 152 respectively, which indicates that in contrast to dioxinones, the first bond formation in the enone photocycloadditions can take place from either the ˛- or ˇ-carbon of the enone via six-membered ring of the corresponding diradical intermediate (Scheme 32).