
860
.pdfЗа прошедший период (2009-2018 г.г.) накоплен oбширный материал по пoчвам заповедников [9, 13-34, 37-46]. В резyльтате полевых исcледований опиcаны морфологичеcкие признаки по клаcсификации почв Росcии [12] 130 почвенных разрезов, заложенных на поcтоянных фенологичеcких площадках (№ 7, 4, 3, 8, 1, 10, 11) хребта Басеги, а также вне cтационарных площадок в различных раcтительных асcоциациях. На Северном Урале проведена диагноcтика почв на горе Хомги-Нѐл, Чувальский Камень, Ветлан (20 почвенных разрезов) по морфологичеcким признакам, гранулометричеcкому, валовoму соcтавам, физикохимичеcким, киcлотно-оcновным cвойствам почв, грyпповому соcтаву гумуcа, оптичеcким свойcтвам гyминовых киcлот. Соcтавленные картоcхемы по гранулометрическому, микроэлементному соcтавам почв для г. Северный Басег указывают на неоднородноcть и мозаичную пестроту пoчвенного покрoва в пространcтве.
С 2015 года проводитcя сиcтематизация данных по соcтаву и свойcтвам почв для заповедников «Басеги», «Вишерский». Соcтавлены базы данных по yсловиям формирования почв в разных высотно-растительных поясах. Иcпользована математичеcкая обработка резyльтатов различными методами: статиcтический, корреляциoнный, информационно-логичеcкий анализ. На оснoвании полyченных данных методом обобщенного проcтранственного анализа проведено геомoделирование почвенного покрoва заповедника «Басеги» и соcтавлена авторcкая пoчвенная карта [16, 19, 21, 23-25, 32, 45]. Также составлена почвенная карта для ключевого участка на горе Хомги-Нѐл в бассейне реки Большая Молебная на территории заповедника «Вишерский» [20, 43].
Определена cтруктура почвенного покрова выcотных ландшафтов, которая являетcя cложной и многокомпонентной [21, 32, 33]. В горных условиях Северного и Среднего Урала отмечаетcя пeстрота и большое разнoобразие почв в пространcтве. Соcтавлен сиcтематический спиcок почв для хрeбта Басеги, в котором предcтавлены почвы 4 cтволов, 8 отделов, внутри которых определены 15 типов, 17 подтипов почв [25]. Изучение почвеннoго покрова запoведников позволило впервые для горной чаcти Пермского края выделить типы почв, о которых ранее не было извеcтно: петроземы, литоземы, подбуры, ржавозѐмы, элювоземы (возможно, подзолы), глееземы.
Таким образом, в связи с труднодоcтупностью территории, иcследования горных почв Среднего и Северного Урала в первой половине 20 века провoдили для отдельных пунктов Пермского края в основном для поиска территорий для расширения площадей сенокоcов и паcтбищ. Несмотря на отсyтствие сиcтематических исcледований почвенного покрова в горной части Урала, историю изyчения горных почв сотрудниками кафедры почвоведения условно можно разделить на несколько пeриодов:
1 – первые опиcания горных почв и факторов почвообразования (20-30-е г.г.);
2 – первая научная экcпедиция Академии наук СССР на Урал (30-40-е г.г.) по изyчению генезиса почв cредних и низких гoр (без участия сотрудников кафедры почвоведения);
151
3– изучение генезиса почв тyндрового и cубальпийского пояcов (50-е г.г.);
4– обобщение имеющихся данных в виде районирования горной части Урала с выделением почвенного окрyга и почвенных районов (60-е г.г.);
5– 70-е годы-2008 г. – сотрудники кафедры не занимались изучением горных почв;
6– комплекcное изучение почвенного пoкрова (с 2008 г.) и факторов почвообразования, генезиcа почв в разных выcотных ландшафтах.
Иcследования почвенного покрова сотрyдниками кафедры почвоведения показали, что почвы cредне- и низкогорных ландшафтов Урала являются yникальными, так как формируются в оcобых экологичеcких условиях и предcтавляют наyчный интереc вследствие малой изyченности, и для выявления оcобенностей горного почвообразовательного процеcса на Урале.
Литература
1.Иванова Е.Н. Горно-лесные почвы Среднего Урала // Труды Почвенного ин-та АН СССР, 1949. Т. 30. С. 168-193.
2.Иванова Е.Н. Почвы Урала // Почвоведение. 1947. № 4. С. 213-227.
3.Канисев Г.Н. О горно-лесных примитивно-аккумулятивных почвах западного склона Среднего Урала // Вопросы почвоведения и агрохимии. Тр. Пермского СХИ. Пермь. 1964. Т. 22. С. 175-188.
4.Канисев Г.Н. О дерновых горно-лесных почвах западного склона Среднего Урала. // Рефераты и тезисы докладов научной конференции. Пермь, 1966.
5.Канисев Г.Н. О почвах подзолистого типа на западном склоне Среднего Урала. // Рефераты и тезисы докладов научной конференции/ Пермь, 1966.
6.Коротаев Н.Я. Почвенные районы Молотовской области. 1945. 67 с.
7.Коротаев Н.Я. Почвы Молотовской области и их свойства. Пермь, 1948. 88 с.
8.Коротаев Н.Я. Почвы Пермской области. Пермь, 1962. С. 247-268.
9.Лузянина О.А., Самофалова И.А. Мониторинг гумусного состояния почв (на примере заповедника «Басеги») // Известия Самарского научного центра Российской академии наук. 2013г. Т. 15. № 3(4). С. 1349-1353.
10.Маландин Г.А. Почвы Урала. Свердловск, 1936. 328 с.
11.Никитин В.В., Маландин Г.А. К вопросу о постановке исследований в северных районах Уралобласти (Верховья р. Камы) // Известия ЕНИ. т.6. вып.3. Пермь, 1928. С. 113-149.
12.Полевой определитель почв. М.: Почвенный институт им. В. В. Докучаева. 2008. 182 с.
13.Сайранова П.Ш., Самофалова И.А. Кислотно-основные свойства горных почв на Северном и Среднем Урале // Научный журнал «Антропогенная трансформация природной среды». Пермь: ПГНИУ, 2018. С. 94-98.
14.Сайранова П.Ш., Самофалова И.А. Формы кислотности горных почв на Северном Урале (заповедник «Вишерский») // Научный журнал «Антропогенная трансформация природной среды». Пермь: ПГНИУ, 2017. С. 193-195.
15.Самофалова И.А. Высотная организация почвенно-топографических рядов на горе Хомги-Нѐл (Северный Урал, заповедник «Вишерский») // Эволюция и деградация почвенного покрова: сборник научных статей по материалам V Межд. научной конференции. Ставрополь: СЕКВОЙЯ, 2017. С. 53-55.
16.Самофалова И.А. Информационно-логический анализ дифференциации почвенного покрова высотных геосистем на Среднем Урале // Вестник Алтайского ГАУ. 2017. № 11 (157). С. 105-114.
17.Самофалова И.А. Морфологические особенности и классификация почв на горе Хомги-Нѐл (хребет Молебный Камень, Северный Урал) // Фундаментальные и прикладные вопросы лесного почвоведения: Материалы докл. VI Всерос. науч. конф. по лесному почвоведению. Сыктывкар, 2015. C. 64-66.
18.Самофалова И.А. Морфолого-генетические особенности и субстантивно-профильная классификация почв высотных поясов хребета Молебный Камень (Северный Урал) // Почвы холодных областей: генезис, география, экология: Всерос. науч. конф. с межд. уч. (к 100-летию со дня рождения профессора О.В. Макеева), 31 августа-9 сентября 2015 г., г. Улан-Удэ, (Бурятия), 2015. С. 100-101.
19.Самофалова И.А. Почвенное разнообразие тундровых и гольцовых ландшафтов в заповеднике
152
"Басеги" // Географический вестник. 2018. № 1. C. 16-28.
20.Самофалова И.А. Почвенный покров бассейна р. Большая Молебная (Северный Урал, заповедник «Вишерский») // Экология речных бассейнов: Труды 9-й Междунар. науч.-практ. конф. / Под общ. ред. проф. Т.А. Трифоновой; Владим. гос. ун-т. им. А.Г. и Н.Г. Столетовых, Владимир, 2018. – С. 90-97.
21.Самофалова И.А. Разнообразие почв низкогорных ландшафтов и особенности их формирования на западном макросклоне Среднего Урала (заповедник «Басеги») // Научно-практический журнал Пермский аграрный вестник. 2017. № 3 (19). С. 10-17.
22.Самофалова И.А. Эволюционный ряд поч на г. Хомги-Нѐл (Северый Урал) // Эволюция и деградация почвенного покрова: сборник науч. статей по матер. IV межд. научной конференции (13-15 октября 2015 года). Ставрополь: Ставропольское издательство «Параграф», 2015. С 45-47.
23.Самофалова И.А., Кучева А.А. Особенности генезиса почв в горной тундре по распределению щебня в профиле (Средний Урал, хребет Басеги). // Научный журнал «Материалы по изучению русских почв». Вып. 11 (38) / Под ред. Б.Ф. Апарина. СПб, 2018. С. 151-155.
24.Самофалова И.А., Лузянина О.А. Горные почвы Среднего Урала (на примере ГПЗ «Басеги»). Пермь. ИПЦ «Прокростъ», 2014. 154 с.
25.Самофалова И.А., Лузянина О.А. Почвы заповедника «Басеги» и их классификация // Пермский аграрный вестник. 2014. № 1 (5). С. 50-60.
26.Самофалова И.А., Лузянина О.А. Эколого-генетическая характеристика почв горно-лесного пояса на Среднем Урале // Известия Самарского научного центра Российской академии наук. 2013. Т. 15. № 3(4). С. 1426-1431.
27.Самофалова И.А., Лузянина О.А., Кондратьева М.А., Мамонтова Н.В. Элементный состав почв в ненарушенных экосистемах на Среднем Урале // Вестник Алтайского ГАУ. 2014. № 5 (115). С. 67-74.
28.Самофалова И.А., Рогова О.Б., Лузянина О.А. Диагностика почв различных высотнорастительных поясов Среднего Урала по групповому составу соединений железа // География и природные ресурсы. 2016. № 1. С. 141-148.
29.Самофалова И.А., Рогова О.Б., Лузянина О.А. Использование группового состава соединений железа для диагностики горных почв Среднего Урала // Бюллетень Почвенного института им. В.В. Докучаева. 2015. № 79. С. 111-136.
30.Самофалова И.А., Рогова О.Б., Лузянина О.А., Савичев А.Т. Геохимические особенности распределения макроэлементов в почвах ненарушенных ландшафтов Среднего Урала (на примере заповедника «Басеги») // Бюллетень Почвенного института им. В.В. Докучаева. 2016. № 85. С. 56-76.
31.Самофалова И.А., Черепанова С.А. Эволюционный ряд почв на г. Хомги-Нѐл (хребет Молебный камень, заповедник «Вишерский») //Мат-ы межрегион. науч.-практ. конф. посвящ. 25летию заповедника «Вишерский» и 100-летнему юбилею заповедной системы России. Пермь:ПГНИУ. 2016. С. 47-52.
32.Самофалова И.А., Шутов П.С. Геосистемно-бассейновый подход как основа изучения структуры почвенного покрова // Вестник Алтайского ГАУ. 2017. №1 (147). С. 49-57.
33.Сарманова З.Р., Самофалова И.А. Почвенный покров болотного массива на западном склоне горы Северный Басег // Научный журнал «Антропогенная трансформация природной среды». Пермь: ПГНИУ, 2017. С. 196-198.
34.Соколова Н.В. Свойства почв субальпийских лугов хребта Басеги // Материалы межд. науч. конф. XVII Докучаевские молодежные чтения / под ред. Б.Ф. Апарина. СПб.: Изд. дом СПГУ, 2014. С. 147-148.
35.Тифлов М.А. К познанию горных лугов Урала // Тр. Пермского СХИ. 1951. т. 13. С. 23-40.
36.Тифлов М.А. Почвы горных лугов Западного Урала: Автореф. Л., 1952. 18 с.
37.Хмелева В.В., Самофалова И.А. Влияние изменения высотно-растительных условий на гранулометрию буроземов (Средний Урал) // Научный журнал «Антропогенная трансформация природной среды». Пермь: ПГНИУ, 2017. С. 198-200.
38.Чебина А.В., Соколова Н.В., Мамонтова Н.В. Эколого-генетическая оценка валового состава горных почв хребта Басеги (Средний Урал) // Материалы межд. научной конф. XVII Докучаевские молодежные чтения / под ред. Б.Ф. Апарина. СПб.: Изд. дом СПБУ, 2014. С. 150-152.
39.Черепанова С.А. Распределение элементарных почвенных частиц в профиле горных почв на Среднем Урале // ЛОМОНОСОВ-2014: XXI Межд. науч. конф. студ., аспир. и молодых уч.: Секция «Почвоведение»; Москва, МГУ: Тезисы докладов. М.: МАКС Пресс, 2014. С. 182-183.
40.Cherepanova S., Samofalova I. Humus Soil Profiles in the Northern Urals («Vishercky» Natural Re-
153
serve) // The Proceedings of the International Congress on ―Soil Science in International Years of Soils‖. 19-23 October, 2015. Sochi, Russia. Article book / Editor: Dr. Ev. Shein. Lomonosov Moscow State University. Moscow, Russia. 2015. P. 72-75.
41.Samofalova I. A., Rogova O. B., Luzyanina O. A. Diagnostics of soils of different altitudinal vegetation belts in the Middle Urals according to group composition of iron compounds // Geography and Natural Resources. 2016. Vol. 1. P. 71–78.
42.Samofalova I. Genetic Charakteristics of Braun Forest Soils on the Middle Urals // American Journal of Environmental Protection. 2015. 4 (3-1). P. 148-156. (http:www.sciencepublishinggroup.com/j/ajep).
43.Samofalova I. Soil Cover in the North Urals // Agricultural Studies. April, 2018. Vol. 2. Issue 2. P. 1- 10. DOI:10.31058/j.as. 2018. 22006. (www.itspoa.com/journal/as).
44.Samofalova I.A. Characteristics of soils in the Northern Urals // The Proceedings of the International Congress on ―Soil Science in International Years of Soils‖. 19-23 October, 2015. Sochi, Russia. Article book / Editor: Dr. Ev. Shein. Lomonosov Moscow State University. Moscow, Russia. 2015. P. 368-371.
45.Samofalova I.A. Geo-modeling of soil cover in inaccessible areas (Perm Region, the Middle Urals) // International Conference ―Global Soil Map 2017‖. Proceedings. Moscow, Russia, July 4-6, 2017. M.: V.V. Dokuchaev Soil Science Institute & Agrarian-Technological Institute, RUDN University, 2017. P. 77.
46.Sayranova P., Samofalova I. Acid track in different types of soils in the Middle Urals // GREEN ROOM SESSIONS 2018. International GEA (Geo Eco-Eco Agro) Conference 1-3 November 2018, Podgorica, Montenegro, 2018. P. 177.
UDC 633.11:631.81
M.K. Khan, A. Pandey, E.E. Hakki
Department of Soil Science and Plant Nutrition, Selcuk University, Konya, Turkey M.S. Akkaya
Department of Chemistry, Middle East Technical University, Ankara, Turkey
WHEAT BIOFORTIFICATION – A POTENTIAL KEY
TO HUMAN MALNUTRITION
Abstract. Wheat production is required to be doubled by 2050 to facilitate the global food assurance. Along with increment in wheat production, improving the nutrient value of wheat varieties is a crucial challenge for wheat breeding society. It is well established that more than 40 % people in the world are dealing with malnutrition caused by the deficiency of Fe, Zn and protein in their food. Numerous strategies are adopted by scientists, breeders and food industries to combat with the situation. In this direction, Biofortificaton has become a successful method to increase the micronutrients content in crop plants either genetically or agronomically. Recently substantial progress has been made in the utilization of molecular marker systems and quantitative trait loci (QTL) to augment the wheat iron, zinc and protein content. Determining the role of GPC-B1 gene in controlling iron, zinc and protein content in wheat genotypes is one of the beneficial steps. Although the gene is found to be associated with elevated micronutrient content, it is responsible for decrease in yield. In order to achieve both, high nutrient content and elevated yield simultaneously, major efforts are required to reveal the genetic control of these features. Also, identifying the wheat genomic resources with elevated nutrient content can play a crucial role. Employment of next generation se-
154
quencing methods and usage of molecular markers in marker assisted selection can be promising to attain the objectives of nutrient rich varieties production. Combining advanced molecular biology and plant breeding techniques for wheat development is a potential strategy in achieving a healthy ―hidden hunger‖ free world.
Keywords: biofortification, grain protein content, malnutrition, wheat
The saying ―Health can be improved by food not medicine‖ is the foundation of wheat breeding and biofortification programs (Powell 2007). More than three billion people, basically from developing countries are victims of micronutrient deficiencies also known as hidden hunger (WHO and FAO 2006). Mainly spread of hidden hunger is attributed to scarcity of necessary micronutrients like iron, zinc and vitamin-A in the food. Although, approximately forty nine nutrients are considered crucial for human metabolic activities (Welch and Graham. 2004), deficiency of any one of them can affect metabolic process leading to poor health and sickness (Branca and Ferrari, 2002; Ramakrishnan et al., 1999). Hidden hunger diminishes physical and mental progress along with the work efficiency of people. As per the estimation of World Health Organization (2009), one fourth world population is suffering from anemia that has maintained its stable position among most disastrous 25 diseases in the world in more than two decades (Murray and Lopez, 2013). Major part of the population in developing countries cannot afford micronutrient loaded diet like fruits and animal based products and depends on staple crops like wheat, rice and maize. Hence, a number of steps are taken forward to enhance the micronutrient supply in human food including mineral supplement, dietary changes and fortification of wheat flour with required vitamins and minerals. In such scenario, consumption of biofortified crops, bred for elevated micronutrient content may serve as a sustainable solution for the problem. Development of micronutrient affluent crops through conventional breeding process or by molecular practices is an effectual tool helpful to remove micronutrient deficient related diseases. Biofortified crops will nourish poor population of the world and considerable improvement of these nutrients can be seen in the aimed people (Welch and Graham, 2004).
Biofortification and its process. Biofortification is the increment in micronutrient bioavailability of cereal crops utilizing conventional breeding or genetic engineering based techniques (Nestel et al., 2006; Hotz and McClafferty, 2007). It is advantageous over fortification due to its strategy of improving nutrient content of during plant development and thus, becoming more approachable to poor communities. Also, micronutrients accumulated in crop grains facilitate the crop production especially when grown in micronutrient deficient soils (White and Zasoski, 1999). Both, agronomic and genetic biofortification are widely being used for nutritional improvement mainly in hidden hunger suffering regions of the world (Bouis et al. 2011). Agronomic biofortification relies on the addition of fertilizers for micronutrient increment in edible part of the crops. Although it is an effective approach handling the malnutrition issue, genetic biofortification is more sustainable. Genetic biofortification including traditional breeding and transgenic methodology leads to introduction of nutritionally rich crop varieties in wheat improvement
155
programs (Gomez-Galera et al., 2010).
Biofortification is a multidisciplinary process relying on skilled expertise of several breeders, geneticists, nutritionists and economists. It starts from the survey of more diverse and nutritious germplasm that can be crossed with high yield genotypes to produce varieties featured with both high yield and nutrient content. Developed varieties are further tested for bioavailability and maintenance of nutrients in various environmental and growth conditions. Among these varieties, most proficient lines should surpass some efficacy tests determining quantifiable amount of nutrient and finally released as novel variety. Both food scientists and economists carry out research to assess the effect of utilizing biofortified crops on human health. Specialists may consider popularizing the crops whose taste or color changes on increasing nutrient content.
These types of colors can facilitate customers for classifying nutritious products. Harvest Plus is contributing to produce and distribute different forms of micronutrient rich (mainly iron, zinc and vitamin A) food worldwide, mainly in developing countries (Brown 1991).
Role of Wheat as a Biofortified Crop. Wheat has gained a tremendously important position among cereal crops because of its nutritional value. Its flour has become a necessary element of bread and other foodstuffs due to the dough making viscoelastic property being main source of nutrition in developing countries. Researchers confirmed that presence of micronutrient enhancement characters in wheat genome ease the improvement of nutrient content in genotypes with no yield loss. Implementing plant breeding is one of the suitable approaches to eradicate malnutrition; however, micronutrient bioavailability is a major concern (Welch 2005). Although many researchers are working to increase nutrient quality of wheat grains, more efforts are necessary to combat with the challenge of nutritional disorders.
Advances in Wheat Biofortification. Till date, extensive progress has been made in field of wheat biofortification addressing relevant issues like uptake of zinc and iron and their accumulation in grain, genetic causes behind this gathering, micronutrients bioavailability and their genetic variation at different wheat ploidy levels. Several scientists have determined that movement of micronutrients in plants, their translocation and bioavailability is reliant on genetic variation and growth conditions as well as controlled by several genes (Bouis and Welch 2010). Different transporters are involved in signaling Fe and Zn mobilization (Sperotto et al., 2012; Deinlein et al., 2012). Despite of their high total content in grain, bioavailability of Fe and Zn is decreased due to their accumulation in aleurone layer that is lost during milling (Borg et al., 2012) and binding with phytates (Guttieri et al. 2006).
Crop improvement strategies through plant breeding are basically dependent on the wheat genetic variation in micronutrient content. Wheat ploidy and evolution have their own roles in developing Zn and Fe content. Cakmak et al., 1999 emphasized on the contribution of A and D genomes in developing zinc efficiency, hence, determining high Zinc efficiency in hexaploids in comparison to tetraploids. Researchers have shown that wild relatives of commercial wheat varieties possess comparably higher
156
grain iron and zinc content than modern/ cultivated wheat cultivars (Cakmak et al., 2000). Harvest-Plus is a key project of Consultative Group on International Agricultural Research (CGIAR) to assess the natural genetic variation for grain nutrient content in wheat germplasm. They have targeted more than 3000 varieties for iron and zinc screening under different environmental and growth conditions that may be involved in further wheat improvement plans. Potential varieties including wheat progenitors like emmer wheat were engaged in breeding programs to transfer high micronutrient trait to selected genotypes making it more effective for quality consumption (Cakmak et al., 2000; OrtizMonasterio et al., 2007).
Recently substantial progress has been made in the utilization of molecular marker systems, quantitative trait loci (QTL) and next generation sequencing techniques to augment the wheat iron, zinc and protein content. Initially, Joppa et al., (1997) proposed and further Uauy et al., 2006 validated that GPC-B1 gene located on chromosome 6BS is a major QTL linked with elevated Fe, Zn and protein content in wild emmer wheat (Triticum turgidum ssp. dicoccoides). Xuhw89 is one of the tightly associated SSR markers to GPC-B1 locus (Distelfeld et al., 2006). Although, several molecular and physiological strategies are used to determine significance of this gene and related transcription factors in T. turgidum accessions, their non-functionality in T. durum and T. aestivum posed new challenges for the proper utilization of the trait. Thus, gene introgression from wild emmer wheat leading to chromosomal substitution lines was proved as a sustainable approach. Some other noteworthy mapping studies determined two different QTLs on 2A and 7A chromosomes associated with grain Fe content and one QTL on 7A chromosome linked with grain Zinc content (Tiwari et al., 2009). On one hand, where Ozkan et al., 2006 demonstrated chromosome 5B linked with elevated iron, zinc, manganese and copper content; on other hand, Genc et al., 2009 found one QTL and four QTLs allied with grain Fe and Zn content respectively in double haploid population. Also, some of the researchers concentrated on regulation of wheat grains Fe and Zn content by silencing of homeoand paralogous genes of GPC-B1 in wheat (Avni et al., 2014). With the advancement of molecular marker technologies since last several years, SNP markers are also being used in this direction to perform association mapping of these crucial traits with different wheat genotypes (Akhunov et al., 2009; Chen et al., 2011; Edae et al., 2013; Saintenac et al., 2013). Determined genes and linked molecular markers can widely facilitate marker assisted breeding programs. However, some of the genes like GPC-B1 responsible for high Fe, Zn and protein content are simultaneously associated with decrease in yield. In order to achieve both, high nutrient content and elevated yield, major efforts are required to reveal the genetic control of these features.
Employing molecular biology and wheat breeding methods with agronomic biofortification gained a considerable success in diminishing food malnutrition from the modern world. However, utilization of promising genetic variability of wild germplasm is a sustainable solution for increasing the nutrient content, bioavailability of those nutrients is a crucial factor need to be addressed. Also, climate change and global warming is emerging as a major problem negatively affecting the wheat grains Fe and Zn content.
157
Other than scientific advancement, it is necessary to develop social vigilance, so that farmers can effectively use these improved nutrient rich wheat varieties for production.
MKK availed TUBITAK Post Doctoral Fellowship during the preparation of the manuscript. MKK and AP equally contributed in designing the manuscript and it was checked by both MSA and EEH.
References
1.Akhunov E., Nicolet C., Dvorak J., (2009) Single nucleotide polymorphism genotyping in polyploid wheat with the Illumina GoldenGate assay. Theor Appl Genet 119: 507-517.
2.Avni R., Zhao R., Pearce S., Jun Y., Uauy C., Tabbita F., Fahima T., Slade A, Dubcovsky J, Distelfeld A (2014) Functional characterization of GPC-1 genes in hexaploid wheat. Planta 239: 313-324.
3.Borg S., Brinch-Pedersen H., Tauris B., Madsen L.H., Darbani B., Noeparvar S., Holm P.B. (2012). Wheat ferritins: improving the iron content of the wheat grain. J Cereal Sci 56: 204-213.
4.Bouis H.E., Eozenou P., Rahman A., (2011) Food Prices, Household Income, and Resource Allocation: Socioeconomic Perspectives on Their Effects on Dietary Quality and Nutritional Status. Food Nutr Bull 32: s14–s23.
5.Bouis H.E., Welch R.M. (2010) Biofortification-a Sustainable Agricultural Strategy for Reducing Micronutrient Malnutrition in the Global South. Crop Sci 50: s1-s13.
6.Branca F., Ferrari M. (2002) Impact of micronutrient deficiencies on growth: the stunting syndrome. Ann Nutr Metab 46: 8-17.
7.Brown K.H. (1991) The Importance of Dietary Quality versus Quantity for Weanlings in Less Developed Countries: A Framework of Discussion. Food Nutr Bull 13: 86–92.
8.Cakmak I. (2002) Plant nutrition research: priorities to meet human needs for food in sustainable ways. Plant and Soil 247: 3-24.
9.Cakmak I., Kalayci M., Ekiz H., Braun H.J., Kilınçe Y., Yilmaz A. (1999) Zinc deficiency as a practical problem in plant and human nutrition in Turkey: a NATO-science for stability project. Field Crops Res 60: 175-188.
10.Chen, Liang; Wang, Shi-Qiang and Hu, Yin-Gang. Detection of Snps in the VRN-A1 Gene of Common Wheat ('Triticum aestivum' L.) by a Modified Ecotilling Method Using Agarose Gel Electrophoresis. Australian Journal of Crop Science, Vol. 5, No. 3, Mar 2011: 321-329.
11.Deinlein U., Weber M., Schmidt H., Rensch S., Trampczynska A., Hansen T.H., Husted S., Schjoerring J.K., Talke I.N., Kramer U., Clemens S. (2012) Elevated nicotianamine levels in Arabidopsis halleri roots play a key role in Zn hyperaccumulation. Plant Cell 24: 708-723.
12.Distelfeld A., Uauy C., Fahima T., Dubcovsky J. (2006) Physical map of the wheat high-grain protein content gene Gpc-B1 and development of a high-throughput molecular marker. New Phytol 169: 753-763.
13.Edae E.A., Byrne P.F., Manmathan H., Haley S.D., Moragues M., Lopes M.S., Reynolds M.P. (2013) Association mapping and nucleotide sequence variation in five drought tolerance candidate genes in spring wheat. Plant Genome 6(2). doi:10.3835/plantgenome2013.04.0010
14.Genc Y., Verbyla A.P., Torun A.A., Cakmak I., Willsmore K., Wallwork H., McDonald G.K. (2009) Quantitative trait loci analysis of zinc efficiency and grain zinc concentration in wheat using whole genome average interval mapping. Plant Soil 314: 49-66.
15.Gomez-Galera S., Rojas E., Sudhakar D., Zhu C., Pelacho A.M., Capell T., Christou P. (2010) Critical evaluation of strategies for mineral fortification of staple crops. Transgenic Res 19: 165-180.
16.Guttieri M.J., Peterson K.M., Souza E.J., (2006) Agronomic performance of low phytic acid wheat. Crop Sci 21: 2623-2629.
17.Hotz C., McClafferty B. (2007) From harvest to health: Challenges for developing biofortified staple foods and determining their impact on micronutrient status. Food Nutr Bull 28: s271-s279.
18.Joppa L.R., Du C., Hart G.E., Hareland G.A. (1997) Mapping gene(s) for grain protein in tetraploid wheat (Triticum turgidum L.) using a population of recombinant inbred chromosome lines. Crop Sci
37:1586-1589.
19.Murray C.J., Lopez A.D. (2013) Measuring the global burden of disease. N Engl J Med 369: 448-457.
20.Nestel P., Bouis H.E., Meenakshi J.V., Pfeiffer W. (2006) Biofortification of staple food crops. J Nutr
136:1064-1067.
21.Organization W.H. (2009) Worldwide prevalence of anaemia 1993-2005: WHO global database on anemia. 2008. Edited by Benoist B., McLea E., Egli I, Cogswell M. Geneva: WHO.
22.Ortiz-Monasterio I.J., Palacios-Rojas N., Meng E., Pixley K., Trethowan R., Pena R.J. (2007) Enhancing the mineral and vitamin content of wheat and maize through plant breeding. J Cereal Sci, 46: 293-307.
158
23.Ozkan H., Brandolini A., Torun A., Altintas S., Eker S., Kilian B. (2006) Natural variation and identification of microelements content in seeds of Einkorn Wheat (Triticum monococcum). In: Proceedings of the 7th International Wheat Conference, 27 Novembere2 December 2005, Mar del Plata, Argentina,
pp.455e462.
24.Powell K. (2007) Functional foods from biotech—an unappetizing prospect?. Nat Biotechnol 25: 525-531.
25.Ramakrishnan U., Manjrekar R., Rivera J., Gonzales-Cossio T., Martorell R. (1999) Micronutrients and pregnancy outcome: a review of the literature. Nutr Res 19: 103-159.
26.Saintenac C., Jiang D., Wang S., Akhunov E. (2013). Sequence-Based Mapping of the Polyploid Wheat Genome. G3: Genes, Genomes, Genetics 3: 1105-1114.
27.Sperotto R.A., Ricachenevsky F.K., Waldow V.A., Fett J.P. (2012) Iron biofortification in rice: it's a long way to the top. Plant Sci 190: 24-39.
28.Tiwari V.K., Rawat N., Chhuneja P., Neelam K., Aggarwal R., Randhawa G.S., Dhaliwal H.S., Keller B., Singh K. (2009) Mapping of quantitative trait loci for grain iron and zinc concentration in diploid
agenome wheat. J Hered 100: 771-776.
29.Uauy C., Brevis J.C., Dubcovsky J. (2006) The high grain protein content gene Gpc-B1 accelerates senescence and has pleiotropic effects on protein content in wheat. J Exp Bot 57: 2785-2794.
30.Welch R.M. (2005) Biotechnology, biofortification, and global health. Food Nutr Bull 26: 419-421.
31.Welch R.M., Graham R.D. (2004) Breeding for micronutrients in staple food crops from a human nutrition perspective. J Exp Bot 55: 353-364.
32.White J.G., Zasoski R.J. (1999) Mapping soil micronutrients. Field Crops Res 60: 11-26.
33.WHO (World Health Organization) and FAO (Food and Agriculture Organization of the United Nations) (2006) Guidelines on Food Fortification with Micronutrients. Geneva.
UDK 631.82
S. Onbası, H. Can, M. Hamurcu, S. Gezgın, E.E. Hakkı,
Department of Soil Science and Plant Nutrition, Faculty of Agriculture, Selcuk University, Konya, Turkey
ADEQUATE SUPPLY OF THE TWO CRITICAL MICROELEMENTS (IRON AND ZINC) TO PLANTS AND TO THE HUMAN
Abstract. Iron (Fe) and zinc (Zn) are micronutrients absolutely necessary for normal development and growth of plants as well as human. When these nutrients are unsufficient in plant tissues, due to the inadequacy in soil, especially at loamy calcareous soil with low organic matter and alkaline reaction, or inefficiency of the crop plants deriving enough of these elements from the soil, many metabolic functions and enzyme activities in plants are inhibited. As a result of this, not only adequate product yield and the desired quality of the produce is prevented but also animals fed on the produce as well as humans consuming these products present deficiency of these elements. Hence, a problem not solved in the soil lead to a chain reaction ended with human health problems.
Keywords: micronutrients, iron, zinc, plant
Micronutrient are absolutely necessary for healthy growth and development of plants and people. Plants, animals as well as human metabolic activities are highly
159
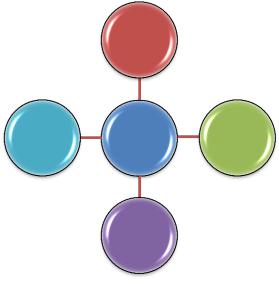
affected by the deficiency of certain micronutrients including iron and zinc. Plants absorb minerals primarily from the soil and accumulate them in different tissues, including grain (Messias et al., 2015). Nearly one half of the world population is effected by malnutrition in terms of micro elements especially iron and zinc as well as elements like selenium and iodine (Zhao and McGrath, 2009). In Turkey and the rest of the developing world, including Africa, Asia and Latin America, children under 5 years of age and pregnant women, are the group most vulnarable to the effects of the deficiency of micronutrients (Bouis and Welch, 2010). When these nutrients are unsufficient in plants, many plant metabolic functions and enzyme activities are inhibitted. As a result of this, adequate product yield and the desired quality is prevented. Thus, in animals or human fed with these products sequential deficiency problems arise. This widespread, but not limited to, developing World problem can possibly be avoided by the agricultural biofortification and/or sophisticated breeding approaches (Garcia-Banuelos et al., 2014; Hefferon, 2015; Velu et al., 2014).
Micronutrients of utmost importance. Considering cereals (especially rice, wheat and maize) are the staple crops of the World, they should be the first crop choices for biological fortification. As of the micronutrients to be considered, iron is one of the most important trace elements for plants and human because its deficiency is the most common and widespread nutritional disorder worldwide (Kacar and Katkat, 2010). In the presence of iron deficiency (Figure 1) important physiological functions and several biochemical reactions catalyzing various enzymes in plant are affected (Nozoye et al., 2014). In human, anemia and susceptibility to many diseases increases and mental development disorders are more frequent (Puig et al., 2007). Micronutrient malnutrition affects 2 billion people in the World, especially in the developing world. Iron (Fe) deficiency alone affects more than 47 % of all preschool aged children.
High
pH
Low |
Iron |
|
Organic |
Deficienc |
Drought |
Matter |
y in Plants |
|
High
Active
CaCO3
Figure 1. The causes of iron deficiency in the plants
Iron is not, however, the only element to consider. Zinc also affects billions of people throught the world (Cakmak et al., 2010). Hence, the second most important trace
160