
Книги по МРТ КТ на английском языке / MR Imaging in White Matter Diseases of the Brain and Spinal Cord - K Sartor Massimo Filippi Nicola De Stefano Vincent Dou
.pdf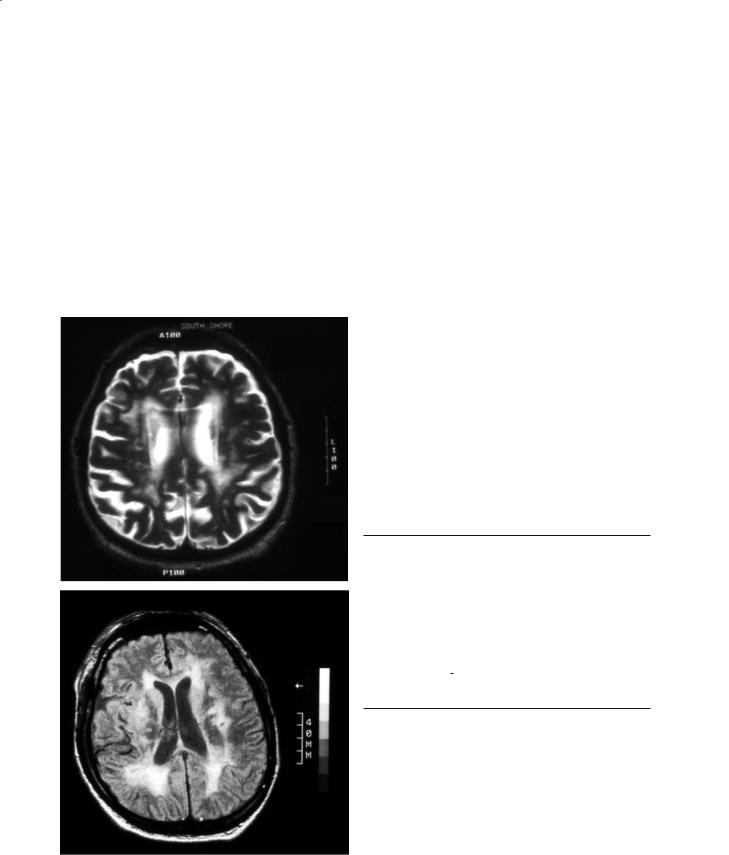
White Matter Abnormalities in Patients with Cerebrovascular Disease |
369 |
Many normal patients have periventricular smooth rims located around the frontal or occipital horns.
Most often, spin-echo (SE) sequences with a long TR and long TE (T2-weighted SE) are used to show white matter abnormalities. If intermediately long TEs (i.e. 40–60 ms) are employed, the lesions have a higher signal than CSF and the surrounding white matter, which improves their identification (protondensity images). Another MR sequence that is particularly suited for the identification of LA is FLAIR (Fig. 25.2). It uses a very long inversion time to suppress CSF, providing an even better contrast between white matter hyperintensities and CSF spaces. In
the elderly, the homogenous low background signal allows for a dramatic demonstration of white matter abnormalities. As previously mentioned, it is the best sequence for separating lacunes,Virchow-Robin spaces and LA (Barkhof and Scheltens 2002).
A variety of scales have been proposed to rate the impact of white matter abnormalities. They have the advantage of providing a semiquantitative and standardized method for reporting LA, but are usually plagued with subjective interpretations and modifications which can lead to a high interand even intraobserver variability (Fazekas et al. 2002). Although no scale has been universally accepted,under the auspices of the European task force on age-related white matter changes a new rating scale was developed and validated for both CT and MRI. Five brain regions are rated following a four-point scale (frontal, pari- eto-occipital, infratentorial, cerebellar and temporal areas). Overall, it showed a good inter-rater reliability for MRI and moderate when CT was used (Wahlund et al. 2001) (Table 25.2, and Fig. 25.3).
The white matter abnormalities commonly found on MRI in the elderly are non-specific and tell the clinician little about their pathogenesis, other than to possibly suggest ischemia. Table 25.3 presents a clinicopathological classification of vascular dementia.
Table 25.2. The age-related white matter changes (ARWMC) scale for CT and MRI.
White matter lesions
0 No lesions
1 Focal lesions
2 Beginning confluence of lesions
3Diffuse involvement of the entire region with or without U fiber involvement
Basal ganglia lesions
0 No lesions
1 1 focal lesion (≥ 5 mm)
2 > 1 focal lesion
3 Confluent lesions
Taken from Wahlund et al. (2001). The following areas are used for rating: frontal, parieto-occipital, temporal, infratentorial/cerebellum, and basal ganglia. Each hemisphere is rated separately.
Fig. 25.2. T2-weighted (top) and FLAIR (bottom) images. The very long inversion time used in FLAIR images results in improved contrast between CSF spaces and white matter hyperintensities
25.5.1 Di usion-Weighted and Di usion Tensor Imaging
Diffusion-weighted imaging (DWI) has the ability to detect (even small) acute and subacute infarcts with great accuracy. It also permits the identification of ischemic lesions in otherwise asymptomatic

370 |
J. A. Gomes and L. R. Caplan |
Table 25.3. Vascular dementia. [Modified with permission from Roman (2002)]
Large-vessel
•Multi-infarct dementia
•Strategic infarct dementia
Small vessel
Subcortical ischemic vascular dementia
•Binswanger’s
•Lacunar dementia (état lacunaire)
•CADASIL
Cortical-subcortical
•Hypertensive and arteriolosclerotic angiopathy
•Cerebral amyloid angiopathies
•Venous occlusions
•Collagen-vascular diseases with dementia
•Other hereditary forms
Ischemic-hypoperfusive
•Diffuse anoxic-ischemic encephalopathy
•Selective vulnerability with restricted injury
•Border-zone infarction
•Incomplete white matter infarction
Hemorrhagic vascular dementia
•Traumatic subdural hematoma
•Subarachnoid hemorrhage
•Cerebral hemorrhage
individuals. Because of these, it may be useful in the longitudinal assessment of patients with LA to monitor the development of new ischemic lesions in the white matter.
In one study, more than one third of patients with vascular dementia associated with small vessel disease were found to have high-signal abnormalities on DWI suggestive of recent ischemia (Choi et al. 2000). In this study the mean apparent diffusion coefficient (ADC) was 0.71±0.15×10–3 mm2/S, the lesions were small (ranging from 0.07 to 2.40 ml), and
Fig. 25.3. ARWMC rating scale. Left, T2-weighted image showing a grade 2 rating score. Right, example of a grade 3 score. [Taken with permission from Wahlund et al. (2001)]
located most commonly in the deep white matter. Remarkably, 20% of asymptomatic individuals had evidence of (silent) ischemia in the cerebral white matter, while some others had multiple small lesions suggesting either a proximal source of embolism or global hypoperfusion (Choi et al. 2000). The severity of LA also seems to correlate with a higher ADC value, and it has been suggested that DWI can be used to differentiate acute and chronic stroke lesions from LA (Helenius et al. 2002). Another potential advantage of this technique is the use of whole brain ADC histograms as a more reliable, quantitative tool to monitor disease progression at various time points (Mascalchi et al. 2002). Although promising, this needs to be further validated.
High b value diffusion MRI (high-b DWI), using b values >3000 s/mm2, may be a more sensitive technique to identify disorders of myelin. In a small, preliminary study of two patients, high-b DWI was analyzed using the q-space approach (Fig. 25.4); a significant reduction in restricted diffusion (attributed to axonal loss and demyelination) much larger than the corresponding hyperintense lesions on T2weighted or FLAIR images was observed. If corroborated, this finding would suggest that high-b DWI is a more sensitive technique for identifying early white matter changes, and that the extent of white matter abnormalities in these patients may be greater than currently assumed (Assaf et al. 2002).
The rate of diffusion of water molecules in normal white matter is not the same in all directions. In white matter tracts, the diffusion is less restricted along the long axis of the axons than across them. Diffusion tensor imaging (DT-MRI) measures the extent to which diffusion is directional (anisotropic), and the fractional anisotropy (FA) is an index of directionality of diffusion. Using this technique,
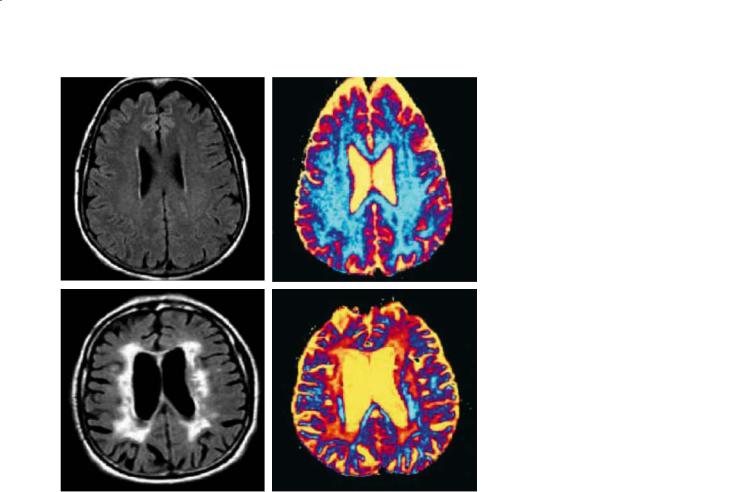
White Matter Abnormalities in Patients with Cerebrovascular Disease |
371 |
Fig. 25.4. FLAIR (left), and q-space probability (right) images of agematched cognitively intact (top) and vascular dementia (bottom) subjects. Arbitrary units are used for the q-space probability image. In the subject with vascular dementia, this imaging modality reveals more extensive white matter abnormalities than those apparent in FLAIR MRI. [Taken from Assaf et al. (2002) with permission]
Jones et al. (1999) found a characteristic pattern of moderate elevation in diffusion trace with a marked loss of FA in patients with LA. The authors postulated that these findings are consistent with the pathological correlate of axonal loss and gliosis commonly observed in LA, both of which would tend to result in decreased anisotropy. DT-MRI was also used to study the normal appearing white matter of patients with LA. Similar findings (increased mean diffusivity and decreased FA) were also found in white matter that appeared normal as assessed by T2-weighted images. Moreover, these subtle changes in DT-MRI correlated with cognitive dysfunction. The pathological substrate of this abnormality is unknown, but these findings also suggest that DTMRI may be a more sensitive technique for the identification of early white matter changes in LA (O’Sullivan et al. 2001).
25.5.2
Magnetization Transfer MRI
Magnetization transfer (MT) is a technique used for tissue characterization. It measures the interaction
between water protons bound to proteins and other macromolecules (such as those in myelin) and unbound tissue water protons. It has been extensively used in the characterization of multiple sclerosis lesions, and is believed to be more specific for white matter damage. This imaging modality has been applied to subjects with LA, and the MT ratios have been reported to be lower than that of normal white matter (38.6±4.5 and 47.3±2.1, respectively), but not nearly as low as in patients with frank demyelination (26.4±5.0). This finding has been attributed to a lesser extent to demyelination or ischemic damage, and more to increased water content (Reidel et al. 2003).
In patients with dementia and LA, the mean MT ratio is also significantly lower than in non-demented patients with similar degrees of LA (37.4+1.5 versus 41.3±1.4), suggesting differences in the pathological substrate (Fig. 25.5). Moreover, the MT ratio of areas of periventricular hyperintensities correlates well with the mini-mental state examination score (Hanyu et al. 1999). Taken together, these findings suggest that in patients with dementia and LA the white matter changes likely are more severe than in non-demented patients.

372 |
J. A. Gomes and L. R. Caplan |
Fig.25.5.T2-weighted MRI (left) and magnetization transfer ratio images (right) from agematched normal (top) and demented (bottom) subjects. Even though the extent of white matter hyperintensities on T2-weighted images is similar in both subjects, the magnetization transfer images show more decreased intensity in the corresponding white matter of the demented patient. [Taken with permission from Hanyu et al. (1999)]
25.5.3
Magnetic Resonance Spectroscopy
Proton magnetic resonance spectroscopic imaging (1H-MRSI) reveals the distribution of the amino acid N-acetylaspartate (NAA) in the brain. NAA is exclusively found in neurons and is considered to be an indicator of neuronal density and metabolism. In patients with LA several interesting findings have been reported. First, decreased levels of NAA were found in the cortex independent of the amount of atrophy, and the frontal cortex NAA seemed to correlate inversely with the volume of white matter hyperintensities. These findings were quite unexpected and suggest that a subcortical injury could induce secondary changes in the cortex (Capizzano et al. 2000).
Secondly and not surprisingly, decreased levels of NAA were found in these same patients, which is consistent with a greater susceptibility of the white matter to chronic ischemic changes. Thirdly, when patients with Alzheimer disease (AD) were compared with patients diagnosed with vascular dementia, they had a significantly lower NAA/creatine ratio in the hippocampus, suggesting that low hippocampal NAA may be relatively specific for AD and that it may help differentiate it from vascular dementia (Capizzano et al. 2000; Schuff et al. 2003).
When otherwise healthy individuals with white matter lesions, controls, and patients with LA and dementia are compared, the NAA/creatine and NAA/ choline ratios of the hyperintense white matter in asymptomatic individuals are virtually the same as those found in healthy controls. In demented patients

White Matter Abnormalities in Patients with Cerebrovascular Disease |
373 |
with LA however, these ratios are reduced, suggesting chronic demyelination or ischemia. This technique may help identify clinically relevant white matter lesions and may also have a role in the diagnosis of vascular dementia.
25.5.4 Perfusion MRI
With recent advances in technique, it is now possible to calculate absolute regional cerebral blood flow (CBF) from MRI using an exogenous paramagnetic contrast agent with an accuracy and spatial resolution that is equal to or better than that of PET studies. Perfusion MRI has been applied to patients with LA in two settings. One has been to measure CBF and cerebral blood volume (CBV) in the actual area of white matter abnormalities. In one such study, the mean white matter CBF of regions of LA was reduced by almost 40% when compared to healthy controls, and this reduction was apparent in all regions of white matter (i.e. anterior, posterior, and superior). Interestingly, grey matter CBV in this same study was significantly increased in the LA group (Markus et al. 2000). The relevance of this finding is unknown at present, but further supports the notion of cortical dysfunction or injury triggered by subcortical insults.
The other setting in which perfusion MRI has been applied is in quantifying CBF in normal appearing white matter in patients with known LA. It has been found that CBF is significantly reduced in the periventricular white matter of these patients (17.9 vs 21.6 ml/100 g/min in controls). Such a reduction, however, was not found in the centrum semiovale (O’Sullivan et al. 2002). These findings support chronic hypoperfusion and ischemic damage with “incomplete infarction” as the underlying pathophysiologic mechanism in this condition. Perfusion MRI could also be used to identify areas of normal appearing white matter that are at risk by virtue of their decreased CBF value and potentially allow interventions that could prevent further injury.
References
Alzheimer A (1894) Die arteriosklerotische atropkie des gehirns. Neurol Zentralbl 13:765-768
Assaf Y, Mayzel-Oreg O, Gigi A et al (2002) High b value q-
space-analyzed diffusion MRI in vascular dementia: a preliminary study. J Neurol Sci 203-204:235-239
Awad I, Spetzler R, Hodak J et al (1986) Incidental subcortical lesions identified on magnetic resonance imaging in the elderly. I. Correlation with age and cerebrovascular risk factors. Stroke 17:1084-1089
Babikian V, Ropper AH (1987) Binswanger disease: a review. Stroke 18:1-12
Bacskai BJ, Hickey GA, Skoch J et al (2003) Four-dimensional multiphoton imaging of brain entry, amyloid binding, and clearance of an amyloidligand in transgenic mice. PNAS 100:12462-12467
Ball MJ (1988) Ischemic axonopathy: further evidence that neocortical pathology accompanying cerebral hypoperfusion during systemic hypotension causes white matter rarefaction in the elderly and some people with Alzheimer dementia. J Neuropathol Exp Neurol 47:388
Ball MJ (2003) White matter lesions, dementia, and ischemic axonopathy. Alz Dis Assoc Dis 17:55
Barkhof F, Scheltens P (2002) Imaging of white matter lesions. Cerebrovasc Dis 13 [Suppl 2]:21-30
Binswanger O (1894) Die abgrenzung der allgemeinen progressiven paralyse. Berl Klin Wochenschr 31:1103-1105, 1137-1139, 1180-1186
Blass JP, Hoyer S, Nitsch R (1991) A translation of Otto Binswanger’s article: the delineation of generalized progressive paralysis. Arch Neurol 48:961-972
Bogousslavsky J, Regli F, Uske A (1987) Leukoencephalopathy with ischemic stroke. Stroke 18:896-899
Boon A, Lodder J, Heuts-van Raak L et al (1994) Silent brain infarcts in 755 consecutive patients with a first-ever supratentorial ischemic stroke: relationship with indexstroke subtype, vascular risk factors, and mortality. Stroke 25:2384-2390
Bornebroek M, Hann J, van Buchem et al (1996) White matter lesions and cognitive deterioration in presymptomatic carriers of the amyloid precursor protein gene codon 693 mutation. Arch Neurol 53:43-48
Breteler MMB, Van Swieten JC, Bots MI et al (1994) Cerebral white matter lesions, vascular risk factors, and cognitive function in a population-based study: the Rotterdam study. Neurology 44:1246-1252
Brooks WM, Wesley MH, Kodituwakku PW et al (1997) 1H MRSI differentiates white matter hyperintensities in subcortical arteriosclerotic encephalopathy from those in normal elderly. Stroke 28:1940-1943
Brown WR, Moody DM, Thore CR, Challa VR (2000) Apoptosis in leukoaraiosis. Am J Neuroradiol 21:79-82
Capizzano AA, Schuff N, Amend DL et al (2000) Subcortical ischemic vascular dementia: assessment with quantitative MR imaging and 1H MR spectroscopy. Am J Neuroradiol 21:621-630
Caplan LR (1995) Binswanger’s disease revisited. Neurology 45:626-633
Caplan LR (2000) Caplan’s stroke, 3rd edn. ButterworthHeinemann, Boston
Caplan LR, Schoene W (1978) Subcortical arteriosclerotic encephalopathy (Binswanger disease): clinical features. Neurology 28:1206-1217
Chan S, Kratha K,Yoon SS et al (1996) Hilal, multifocal hypointense cerebral lesions on gradient-echo MR are associated with chronic hypertension. Am J Neuroradiol 17:18211827

374
Choi SH, Na DL, Chung CS et al (2000) Diffusion-weighted MRI in vascular dementia. Neurology 54:83-89
Davous P (1998) CADASIL: a review with proposed diagnostic criteria. Eur J Neurol 5:219-233
Dechambre A (1838) Mémoire sur la curabilité du ramollissement cerebral. Gaz Méd Paris 6:305-314
Domínguez RO, Marschoff ER, Serra JA et al (2002) Stroke vs. chronic progressive cerebrovascular disease: a magnetic resonance imaging study of symptomatic outpatients. J Neurol Sci 203/204:67-71
Dubas F, Gray F, Roullet E, Escourolle R (1985) Leukoencephalopathies arteriopathiques. Rev Neurol 141:93-108
Durand-Fardel M (1843) Traité du ramollissement du cerveau. Baillière, Paris
Fazekas F, Barkhof F, Wahlund LO et al (2002) CT and MRI rating of white matter lesions. Cerebrovasc Dis 13 [Suppl 2]:31-36
Fisher CM (1965) Lacunes, small deep cerebral infarcts. Neurology 15:774-784
Fisher CM (1969) The arterial lesions underlying lacunes.Acta Neuropathol 12:1-15
Fisher CM (1989) Binswanger’s encephalopathy: a review. J Neurol 236:65-79
Furuta A, Ishii N, Nishihara Y et al (1991) Medullary arteries in aging and dementia. Stroke 22:442-446
Ghika J, Bogousslavsky J (1996) White matter disease and vascular dementia. In: Prohovnik I, Wade J, Knezevic S, Tatemichi T, Erkinjuntti T (eds) Vascular dementia: current concepts. Wiley, New York, pp 113-141
Gorter JW for the stroke prevention in reversible ischemia trial (SPIRIT), and European atrial fibrillation trial (EAFT) study groups (1999) Major bleeding during anticoagulation after cerebral ischemia. Neurology 53:1319
Grabowski TJ, Cho HS, Vonsattel JPG et al (2001) Novel amyloid precursor protein mutation in an Iowa family with dementia and severe cerebral amyloid angiopathy. Ann Neurol 49:697-705
Gray F, Dubas F, Roullet E et al (1985) Leukoencephalopathy in diffuse hemorrhagic cerebral amyloid angiopathy. Ann Neurol 18:54-59
Greenberg SM (2002) Cerebral amyloid angiopathy and vessel dysfunction. Cerebrovasc Dis 13 [Suppl 2]:42-47
Greenberg SM, Vonsattel JPG (1997) Diagnosis of cerebral amyloid angiopathy. Sensitivity and specificity of cortical biopsy. Stroke 28:1418-1422
Gupta SR, Naheedy MH, Young J et al (1988) Periventricular white matter changes and dementia. Clinical, neuropsychological, radiological, and pathological correlate. Arch Neurol 45:637-641
Hachinski V, Potter P, Merskey H (1987) Leuko-araiosis. Arch Neurol 44:21-23
Hanyu H, Asano T, Sakurai H et al (1999) magnetization transfer ratio in cerebral white matter lesions of Binswanger’s disease. J Neurol Sci 166:85-90
Hanyu H, Tanaka Y, Shimizo S et al (2003) Cerebral microbleeds in Binswanger’s disease: a gradient-echo T2*- weighted magnetic resonance imaging study. Neurosci Lett 340:213-216
Helenius J, Soinne L, Salonen O et al (2002) Leukoaraiosis, ischemic stroke, and normal white matter on diffusionweighted MRI. Stroke 33:45-50
Hendricks HT, Franke CL, Theunissen PHMH (1990) Cerebral
J. A. Gomes and L. R. Caplan
amyloid angiopathy: diagnosis by MRI and brain biopsy. Neurology 40:1308-1310
Hénon J, Godefroy O, Lucas Ch et al (1996) Risk factors ofr leuko-araiosis in stroke patients.Acta Neurol Scand 94:137144
Hijdra A, Verbeeten B Jr (1991) Leukoaraiosis and ventricular enlargement in patients with ischemic stroke. Stroke 22:447-450
Hijdra A, Verbeeten B Jr, Verhulst JAPM (1990) Relationship of leuko-araiosis to lesion type in stroke patients. Stroke 21:890-894
Inzitari D, Diaz F, Fox A et al (1987) Vascular risk factors and leuko-araiosis. Arch Neurol 44:42-47
Jones Dk, Lythgoe D, Horsfield MA et al (1999) Characterization of white matter damage in ischemic leukoaraiosis with diffusion tensor MRI. Stroke 30:393-397
Kase CS (1994) Cerebral amyloid angiopathy. In: Kase CS, Caplan LR (eds) Intracerebral hemorrhage. ButterworthHeinemann, Boston, pp 179-200
Kato H, Izumiyama K, Takahashi K et al (2002) Silent cerebral microbleeds on T2*-weighted MRI: correlation with stroke subtype, stroke recurrence, and and leukoaraiosis. Stroke 33:1536-1540
Leys D, Englund E, Del Ser T et al (1999) White matter changes in stroke patients: relationship with stroke subtype and outcome. Eur Neurol 42:67-75
Liao D, Cooper L, Cai J et al (1997) The prevalence and severity of white matter lesions, their relationship with age, ethnicity, gender, and cardiovascular risk factors: the ARIC study. Neuroepidemiology 16:149-162
Lindgren A, Roijer A, Rudling O et al (1994) Cerebral lesions on magnetic resonance imaging, heart disease, and vascular risk factors in subjects without stroke; a populationbased study. Stroke 25:929-934
Loes DJ, Biller J, Yuh WTC et al (1990) Leukoencephalopathy in cerebral amyloid angiopathy: MR imaging in four cases. Am J Neuroradiol 11:485-488
Longstretch WT Jr, Manolio T, Arnold A et al (1996) Clinical correlates of white matter findings on cranial magnetic resonance imaging of 3301 elderly people: the cardiovascular health study. Stroke 27:1274-1282
Mandybur TI (1986) Cerebral amyloid angiopathy: the vascular pathology and complications. J Neuropathol Exp Neurol 45:79-90
Mäntylä R, Aronen HJ, Salonen et al (1999) Magnetic resonance imaging white matter hyperintensities and mechanism of ischemic stroke. Stroke 30:2053-2058
Marie P (1901) Des foyers lacunaires de disintegration et des differents autres états cavitaires du cerveau. Rev Méd 21:281-298
Markus HS, Lythgoe DJ, Ostegaard L et al (2000) Reduced cerebral blood flow in white matter in ischaemic leukoaraiosis demonstrated using quantitative exogenous contrast based perfusion MRI. J Neurol Neurosurg Psychiatry 69:48-53
Martinez-Lage P, Hachinski V (1998) Multi-infarct dementia. In: Barnett HJM, Mohr JP, Stein BM, Yatsu FM (eds) Stroke: pathophysiology, diagnosis and management. Churchill Livingstone, New York, pp 875-894
Mas JL, Dilouya A, de Recondo J (1992) A familial disorder with subcortical ischemic strokes, dementia, and leukoencephalopathy. Neurology 42:1015-1019
Mascalchi M, Moretti M, Della Nave R et al (2002) Longitudinal

White Matter Abnormalities in Patients with Cerebrovascular Disease |
375 |
evaluation of leukoaraiosis with whole brain ADC histograms. Neurology 59:938-940
Merino JG, Hachinski V (2000) Leukoaraiosis. Arch Neurol 57:925-926
Moody DM, Brown WR, Challa VR, Anderson RL (1995) Periventricular venous collagenosis: association with leukoaraiosis. Radiology 194:469-476
Murata T, Handa H, Mori K et al (1981) The significance of periventricular lucency on computed tomography: experimental study with canine hydrocephalus. Neuroradiology 20:221-227
Nag S (1984) Cerebral changes in chronic hypertension: combined permeability and immunohistochemical studies. Acta Neuropathol 62:178-184
O’Sullivan M, Summers PE, Jones DK et al (2001) Normalappearing white matter in ischemic leukoaraiosis: a diffusion tensor MRI study. Neurology 57:2307-2310
O’Sullivan M, Lythgoe DJ, Pereira AC et al (2002) Patterns of cerebral blood flow reduction in patients with ischemic leukoaraiosis. Neurology 59:321-326
Ohtani R,Tomimoto H,Kawasaki T et al (2003) Cerebral vasomotor reactivity to postural change is impaired in patients with cerebrovascular white matter lesions. J Neurol 250:412-417
Olszewski J (1965) Subcortical arteriosclerotic encephalopathy. World Neurol 3:359-374
Pantoni L (2002) Pathophysiology of age-related cerebral white matter changes. Cerebrovasc Dis 13 [Suppl 2]:7-10
Pantoni L, Garcia J (1997) Pathogenesis of leukoaraiosis. Stroke 28:652-659
Raiha I, Tarvonen S, Kurki T et al (1993) Relationship between vascular factors and white matte low attenuation of the brain. Acta Neurol Scand 87:286-289
Ravens JR (1974) Anastomoses in the vascular bed of the human cerebrum. In: Cervós-Navarro J (ed) Pathology of cerebral microcirculation. De Gruyter; Berlin, pp 26-38
Reidel MA, Stippich C, Heiland S et al (2003) Differentiation of multiple sclerosis plaques, subacute ischemic cerebral infarcts, focal vasogenic oedema and lesions of subcortical arteriosclerotic encephalopathy using magnetization transfer measurements. Neuroradiology 45:289-294
Roman GC (1991) White matter lesions and normal-pressure hydrocephalus: Binswanger’s disease or Hakim syndrome. Am J Neuroradiol 12:40-41
Roman GC (2002) On the history of lacunes, état criblé, and the white matter lesions of vascular dementia. Cerebrovasc Dis 13 [Suppl]:1-6
Roman GC, Erkinjuntti T, Wallin A et al (2002) Subcortical ischaemic vascular dementia. Lancet Neurol 1:426-436 Rosenberg GA,Sullivan N,Esiri M (2001) White matter damage
is associated with matrix metalloproteinases in vascular dementia. Stroke 32:1162
Scheltens P, Barkhof F, Leys D et al (1995) Histopathological correlates of white matter changes on MRI in Alzheimer’s disease and normal aging. Neurology 45:883-888
Schmidt R, Schmidt H, Kapeller P et al (2002) Evolution of white matter lesions. Cerebrovasc Dis 13 [Suppl 2]:16-20
Schmidt R, Enzinger C, Ropele S et al (2003) Progression of cerebral white matter lesions: 6-year results of the Austrian stroke prevention study. Lancet 361:2046-2048
Schuff N, Capizzano AA, Du AT et al (2003) Different patterns of N-acetylaspartate loss in subcortical ischemic vascular dementia and AD. Neurology 61:358-364
Smith EE, Rosand J, Knudsen KA et al (2002) Leukoaraiosis is associated with warfarin-related hemorrhage following ischemic stroke. Neurology 59:193-197
Streifler JY, Eliaziw M, Benavente OR et al (1995) Lack of relationship between leukoaraiosis and carotid artery disease. Arch Neurol 52:21-24
Tanaka A, Ueno Y, Nakayama Y et al (1999) Small chronic hemorrhages and ischemic lesions in association with spontaneous, intracerebral, hematomas. Stroke 30:1637-1642
Tomonaga M,Yamanouchi H, Tohgi H et al (1982) Clinicopathologic study of progressive subcortical vascular encephalopathy (Binswanger type) in the elderly. J Am Geriatr Soc 30:524-529
Tournier-Lasserve E, Iba-Zizen I-T, Romero N, Bousser M-G (1991) Autosomal dominant syndrome with stroke-like episodes and leukoencephalopathy. Stroke 22:1297-1302
Van den Bergh R,van der Eecken H (1968) Anatomy and embryology of cerebral circulation. Prog Brain Res 30:1-26
Veldink JH, Scheltens P, Jonker C et al (1998) Progression of cerebral white matter hyperintensities on MRI is related to diastolic blood pressure. Neurology 51:319-320
Vermeer SE, Koudstaal PJ, Oudrek M et al (2002) Prevalence and risk factors of silent brain infarcts in the populationbased Rotterdam scan study. Stroke 33:21-25
Vinters HV, Gilbert JJ (1983) Cerebral amyloid angiopathy: incidence and complications in the aging brain. II. The distribution of amyloid vascular changes. Stroke 14:924928
Vonsattel JP, Myers RH, Hedley-Whyte ET et al (1991) Cerebral amyloid angiopathy without and with cerebral hemorrhages: a comparative histological study. Ann Neurol 30:637-649
Wahlund LO, Barkhof F, Fazekas F et al (2001) A new rating scale for age-related white matter changes applicable to MRI and CT. Stroke 32:1318-1322
Wattendorff AR, Frangione B, Luyendijk W et al (1995) Hereditary cerebral hemorrhage with amyloidosis, Dutch type (HCHWA-D): clinicopathological studies. J Neurol Neurosurg Psychiatry 58:699-705
Whitman GT, Tang T, Lin A et al (2001) A prospective study of cerebral white matter abnormalities in older people with gait dysfunction. Neurology 57:990-994
Ylikoski A, Erkinjuntti T, Raininko et al (1995) White matter hyperintensities on the MRI in the neurologically nondemented elderly: analysis of cohorts of consecutive subjects aged 65 to 85 years living at home. Stroke 26:1178111787
Zimmerman RD, Fleming CA, Lee BCP et al (1986) Periventricular hyperintensities as seen by magnetic resonance: prevalence and significance. AJNR 7:13-20

Neurodegenerative Diseases with Associated White Matter Pathology |
377 |
26Neurodegenerative Diseases
with Associated White Matter Pathology
Mario Mascalchi
CONTENTS
26.1Introduction 377
26.2Degenerative Dementias 378
26.2.1Alzheimer Disease 378
26.2.1.1Conventional MR 378
26.2.1.2Non-conventional MR 379
26.2.2 Fronto-temporal Dementia (FTD) 380
26.2.2.1Conventional MR 380
26.2.2.2Non-conventional MR 380
26.3Degenerative Ataxias 380
26.3.1Conventional MR 381
26.3.2Non-conventional MR 381
26.3.2.11H-MRS 381
26.3.2.2MTI 383
26.3.2.3Diffusion MR 383
26.4 |
Motor Neuron Disease 384 |
26.4.1Conventional MR 384
26.4.2Non-conventional MR 384
26.4.2.11H-MRS 384
26.4.2.2MTI 386
26.4.2.3Diffusion MR 386
26.5Conclusions 386 References 386
26.1 Introduction
Degenerative diseases of the central nervous system (CNS) comprise a variety of sporadic or inherited conditions characterized by progressive neuronal dysfunction and loss of unknown or incompletely known etiopathogenesis which typically are selective of some neuronal systems (Adams and Victor 1985). While the neuropathological hallmark of degenerative diseases of the CNS is a generally symmetric gray matter cortical or subcortical damage, white matter
M. Mascalchi, MD
Professor, Sezione di Radiodiagnostica, Dipartimento di Fisiopatologia Clinica, Università di Firenze, Viale Morgagni, 85, 50134 Firenze, Italy
changes in the brain or spinal cord are increasingly recognized as a frequent magnetic resonance (MR) finding in most of them. In some instances they are apparent in the conventional MR examination and are useful diagnostic features, in other cases they are not visible in the conventional MR examination but can be demonstrated using non-conventional MR techniques.
Conventional MR imaging includes T1, proton density and T2 (with and without inversion recovery RF pulse to attenuate the CSF signal) weighted sequences. The non-conventional MR include proton MR spectroscopy (1H-MRS), magnetization transfer imaging (MTI), and diffusion MR imaging.
1H-MRS enables an in vivo biochemical characterization of operator selectable volume of interest through the evaluation of the absolute or relative concentrations of some proton containing metabolites such as N-acetyl-aspartate (NAA), which is a marker of neuronal integrity and function, creatine plus phosphocreatine (Cr), which are markers of cell density and energy state, choline-containing compounds (Cho), which are markers of membrane metabolism, myo-inositol (mI), which is a marker of glial cell density, and glutamine (Gln) and glutamate (Glu). Although in many 1H-MRS studies of degenerative diseases of the CNS it was claimed that the volume of interest was placed in the cortical gray matter, with the current resolution of the single and multi-voxel techniques used for 1H-MRS there is always partial volume with the white matter and the spectral findings variably reflect also white matter changes.
Magnetization transfer imaging (MTI) is based on the interaction between protons in a relatively free state and those in a restricted motion state and indirectly reflects the capacity of the molecules of the tissue matrix to exchange energy with the surrounding water molecules (McGowan et al. 1998). A decrease of the MT ratio usually indicates a damage of the tissue matrix.
Diffusion MR imaging exploits the sensitivity of MR to motion of water molecules and provides a sensitive tool to explore the integrity of tissue and

378
cellular barriers to water motion (Schaefer et al. 2000; Dong et al. 2004). The evaluation of maps of apparent diffusion coefficients (ADC) or mean diffusivity (D) evaluation is emerging as a quantitative, reproducible, and sensitive method to assess white and gray matter brain diseases (Cercignani et al. 2000; Bozzali et al. 2001; Cercignani et al. 2003). In particular, analysis of the brain D can provide regional and global quantification not only of the macroscopic damage shown as areas of prolonged T2 but also of microscopic tissue damage which is not demonstrated by T2 weighted images. To this end, region of interest and histogram approaches are complementary. In fact, histogram analysis is intrinsically more powerful from a statistical point of view than region of interest analysis (Chun et al. 2000) and is more suited to evaluate diffuse pathological processes as those underlying degenerative diseases of the CNS (van Buchem 1999). On the other hand, the loss of spatial information associated to histogram analysis can be compensated using regions of interest analysis based on an a priori knowledge of the distribution of the pathological damage.
In this chapter we shall review the most frequent degenerative diseases of the CNS in which conventional and non-conventional MR studies have documented significant “macroscopic” or “microscopic” white matter changes.
26.2
Degenerative Dementias
26.2.1
Alzheimer Disease
Although the neuropathological hallmarks of Alzheimer disease (AD), namely intracellular neurofibrillary tangles and extracellular amyloid plaques, are observed in the cerebral gray matter, diffuse white matter damage which is related to microvascular pathology is demonstrated in the brains of AD patients (Brun and Englund 1986; Sjobeck et al. 2003). Moreover, Kril et al. (2002) observed that the degree of hippocampal neuronal loss and atrophy was similar in demented patients fulfilling the neuropathological criteria for small vessel disease dementia and AD, respectively. These data indicate that even on a pathological basis, a complete separation of AD from white matter damage due to vascular disease is not always possible.
M. Mascalchi
26.2.1.1 Conventional MR
Proton density and T2 weighted images can show focal or diffuse white matter signal hyperintensity in patients with clinically probable AD (Fazekas et al. 1987). The most characteristic hyperintensities are symmetric and located along the cerebral periventricular regions or in the subcortical hippocampal and sylvian regions (Fazekas et al. 1987; Scheltens et al. 1990) (Fig. 26.1). Interestingly, de Leeuw et al. (2004) recently reported that white matter lesions were present on MR imaging in 29% of patients with a clinical diagnosis of probable AD and that there was a correlation between white matter signal changes, especially in the frontal and parieto-occipital regions, and hippocampal atrophy suggesting a relationship between vascular and typical AD pathology.
Although a loss of bulk of the white matter contributes to the pattern and progression of global and regional atrophy demonstrated by 3D T1 weighted images in cross-sectional and longitudinal studies in AD (Chan et al. 2001; Rusinek et al. 2003), most volumetric studies in AD measured the hippocampal formations alone (Jack et al. 2002; Dixon et al. 2002).
Voxel based morphometry (VBM) was introduced in 2000 as a new approach for global and regional estimation of brain volumes which is based on the statistical parametric mapping (SPM) soft-
Fig. 26.1. Alzheimer disease. Axial T2 weighted FLAIR image shows symmetric hyperintensity in the hippocampus and subhippocampal white matter of a patient with probable AD

Neurodegenerative Diseases with Associated White Matter Pathology |
379 |
ware developed for nuclear medicine and functional MR techniques. SPM analysis combines a strong statistical power with an observer unbiased high resolution analysis (Ashburner and Friston 2000). Curiously, many studies employing VBM emphasized regional cortical gray matter loss (Baron et al. 2001; Frisoni et al. 2002; Karas et al. 2003), but none explored possible white matter volume changes in AD.
26.2.1.2 Non-conventional MR
26.2.1.2.1 1H-MRS
In degenerative diseases of the CNS, 1H-MRS shows a decrease of the concentration of NAA or of the NAA/Cr ratio in the areas where there is the maximal neuronal damage and loss on neuropathological examination.
Spectroscopy was employed since the early 1990s in the evaluation of patients with clinical diagnosis of AD. Single voxels and MR spectroscopic imaging acquisitions were performed with volumes of interest placed in a number of sites, which included the occipital region (Shonk et al. 1995), the frontal region (Ernst et al. 1997), the parietal region (Schuff et al. 2002) the hippocampus (Dixon et al. 2002) the posterior cingulate (Kantarci et al. 2003) and the subcortical white matter (Catani et al. 2000).
The two main findings of 1H-MRS in AD, essentially independent of the voxel locations, are a decrease of NAA concentration or NAA/Cr ratio and an increase of mI concentration or of the mI/Cr ratio, which can be expressed as NAA/mI ratio (Shonk et al. 1995). The sensitivity and the specificity of the two 1H-MRS findings considered alone or combined for differentiation of mildly demented cases from age matched controls and for differentiation of AD from others dementias are high but not perfect (Shonk et al. 1995; Rapaport 2002; Catani et al. 2000). The decrease of NAA, NAA/Cr or NAA/mI correlate with the severity of the disease as judged based on the clinical and neuropsychological evaluation (Chantal et al. 2002; Waldman and Rai 2003), but its capacity to mark disease progression or cognitive decline in longitudinal studies is controversial (Jessen et al. 2001; Dixon et al. 2002).
This notwithstanding, 1H-MRS was employed as a surrogate marker to evaluate the efficacy of a drug in a randomized clinical trial in patients with AD (Kishnan et al. 2003).
26.2.1.2.2 MTI
Bozzali et al. (2001) reported that the peak of the MT ratio histogram of the cerebral hemispheres and of the temporal lobes was reduced in patients with AD as compared to age matched healthy controls. Similar results in the whole brain, temporal and frontal lobes of patients with AD and mild cognitive impairment were reported in a study showing that the cognitive decline in these two categories of patients correlated with the relative peak height of the whole brain MT ratio histogram (van der Flier et al. 2002).
26.2.1.2.3 Di usion MR
Two early studies of diffusion MR imaging in patients with clinical suspicion of AD employed the region of interest analysis in order to detect possible D changes in the cortical gray matter and subcortical white matter (Hanyu et al. 1998; Bozzao et al. 2001). Although both studies failed to demonstrate significant D changes in the hippocampi, one study (Hanyu et al. 1998) reported an increase of D and a decrease of anisotropy in the white matter of the temporal lobe which correlated with the clinical severity. Moreover, ADC perpendicular to the commissural fibers of the corpus callosum was increased and anisotropy decreased in patients with AD, the latter correlating with the mini-mental state examination score (Hanyu et al. 1999). Subsequent studies with diffusion tensor imaging confirmed the increase of D and the reduction of the fractional anisotropy in the association white matter tracts but not in the internal capsule of patients with clinical diagnosis of AD (Rose et al. 2000; Bozzali et al. 2002). Moreover, a strong correlation was reported between the score at the mini-mental state examination and an average overall white matter D and fractional anisotropy (Bozzali et al. 2002). Using histogram analysis after segmentation of the gray and white matter based on different FA, Bozzali et al. (2001) confirmed the increase of D in the cerebral white matter and cortical gray matter in patients with AD.
Interestingly,loss of anisotropy of the frontal white matter was found to increase with age and to correlate with neuropsychological evidence of cognitive decline in normal aging (O’Sullivan et al. 2001).