
- •Introduction
- •Overview of the integration between bone tissue and implants
- •Immune cells and osteoclastogenesis
- •Immune cells and osteogenesis
- •Multiple roles of macrophages in the bone healing process
- •Bone biomaterials modulating the immune response
- •Surface properties of bone biomaterials
- •Biomaterials particle size
- •Porosity and pore size of biomaterials
- •Released ions from bone biomaterials
- •Indirect co-culture using conditioned medium
- •Indirect co-culture using Boyden chambers
- •Direct co-culture
- •Possible evaluation process of osteoimmunomodulation
- •Possible strategies to endow advanced bone biomaterials with favorable osteoimmunomodulation
- •Conclusions
- •Acknowledgements
- •References
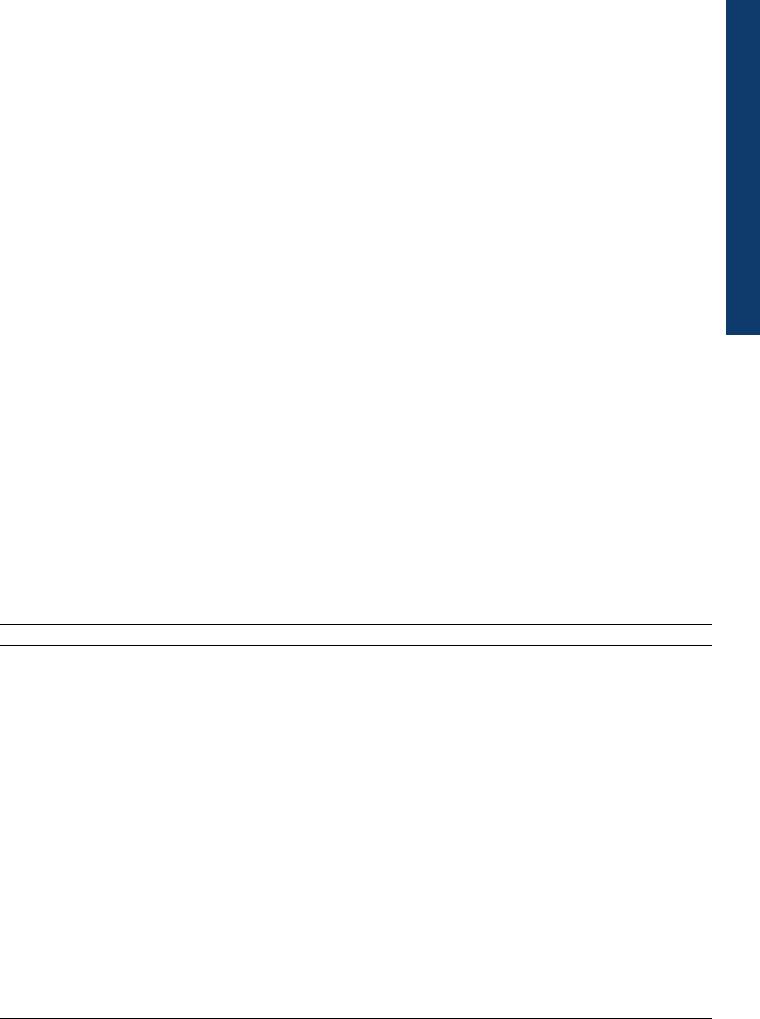
Materials Today Volume 19, Number 6 July/August 2016 |
RESEARCH |
Direct co-culture
Direct co-cultures enable both immune and bone cells to be in direct contact with the materials at the same time, which would be the case in an in vivo environment. It can be performed by layering one cell type on top of another, although this method is fraught with technical difficulties of which reproducibility of results is the most prevalent [194]. It requires the involvement of cells sourced from one individual patient, especially when immune cells are involved. Primary culture of whole bone marrow tissue derived from surgery or biopsy may meet the requirement of providing various types of cells from different tissues, including skeleton and immune systems. Inter-patient variability and limited source of suitable donor tissues makes it difficult to establish this technique as the standard of in vitro evaluation. Another limitation is separating the effects of the different cell types. The cells could be sorted by fluorescence-activated cell sorting (FACS) or magnetic-activated cell sorting (MACS) and then evaluated by gene expression, but this is fraught with difficulties. Also, the proteins in the media cannot be ascribed to one particular cell type. Indirect co-culture using Boyden chambers may, therefore, be the most suitable and reproducible evaluation system for the assessment of OIM.
Possible evaluation process of osteoimmunomodulation
As has been pointed out throughout this review, immune cells play a number of roles during the various phases of bone wound healing. This implies that the evaluation of OIM must be phaseoriented. During the early phase, acute inflammation reaction is predominant and the induced immune environment will decide the recruitment of MSCs and initiation of osteogenesis. There are four major events that takes place during the early phase: (1) the activation of putative inflammation related signaling pathways in
response to the implants; (2) release of inflammatory cytokines responsible for the acute inflammation; (3) release of chemokines and their effect on the recruitments of MSCs; and (4) release of factors that regulate osteogenesis and fibrosis, which ultimately will determine the outcome of new bone regeneration.
The mid phase of the repair response is characterized by three major events: (1) activation of osteogenic signaling pathways in response to the factors released during the early stage; (2) osteogenic differentiation of MSCs that determines the osteogenesis; and (3) release of osteoclastogenic factors from both immune and osteoblast cells that accelerates bone remodeling and degradation of the remaining biomaterials in the late phase.
During the late phase of the repair response the induced immune environment enhances osteoclastogenesis. There are two major events taking place during the late phase: (1) activation of osteoclastogenic signaling pathways in response to factors released during the mid-phase; (2) differentiation of osteoclasts and enhanced activity shift the overall balance from osteogenesis toward osteoclastogenesis. Should there be a failure to activate osteoclastogenesis during this phase this may prevent the timely degradation of biomaterials and impede a proper integration between bone and materials.
Possible strategies to endow advanced bone biomaterials with favorable osteoimmunomodulation
It has emerged from both our own and other studies that bone biomaterials elicit significant effects on the immune system. The immune response determines subsequent osteogenesis and osseointegration and is a reflection of the importance of the immune system to both normal and pathological bone physiology. Immune cells, such as macrophages, have a high degree of plasticity, which makes it possible to modulate the immune
TABLE 2
Possible strategies to endow bone biomaterials with favorable osteoimmunomodulation.
Strategies |
Methods |
Examples |
|
|
|
Modify the composition |
Incorporate nutrient elements |
Different combinations of nutrient elements (e.g. Mg, Sr, |
|
|
Si) have been applied to manipulate OIM [148,202]. |
|
Incorporate bioactive molecules, such as |
OSM has received great attention in osteoimmunology |
|
macrophage inducer (e.g. IL-4, LPS) or inflammatory |
area, due to its dual effects on regulating both |
|
cytokines (e.g. IL-10, TNFa, IFNg, OSM) |
osteogenesis and osteoclastogenesis [28]. Incorporating |
|
|
proper dose of OSM can be a valuable strategy to direct |
|
|
bone regeneration. |
|
|
|
Optimize the fabrication form |
Immune reaction can be manipulated by changing |
Inert materials such as gold can become immunogenic |
|
the particle size: from bulk, particle, powder, |
when it is fabricated into nano particles [108]. |
|
microscale, to nanoscale. |
|
|
For those fabricated into 3D structured scaffolds, |
A uniform 30–40 mm pore size appears to promote the |
|
pore size and porosity should be optimized. |
polarization toward the M2 phenotype [118], whereas |
|
|
non-porous or random-porous-sized materials appear to |
|
|
favor the M1 phenotype [196]. |
|
|
|
Change the surface properties |
Modify the surface topology of materials (size and |
Material surfaces that provide biomimetic cues, such as |
|
roughness) |
nanoscale structures, have been found to regulate cell/ |
|
|
biomaterial interactions [80,195]. |
|
Modify the surface chemistry of materials |
Macrophages cultured on surfaces with different |
|
(hydrophility and electric potential) |
hydrophilicity results in different protein expression |
|
|
profiles and cytokine responses [66]. |
|
|
|
Others |
Couple with immunomodulatory drugs |
The local administration of anti-inflammatory drug |
|
|
(aspirin) enhances the bone tissue regeneration outcome |
|
|
via regulating the immune response toward implanted |
|
|
stem cells and bone substitute materials [53]. |
RESEARCH: Review
317
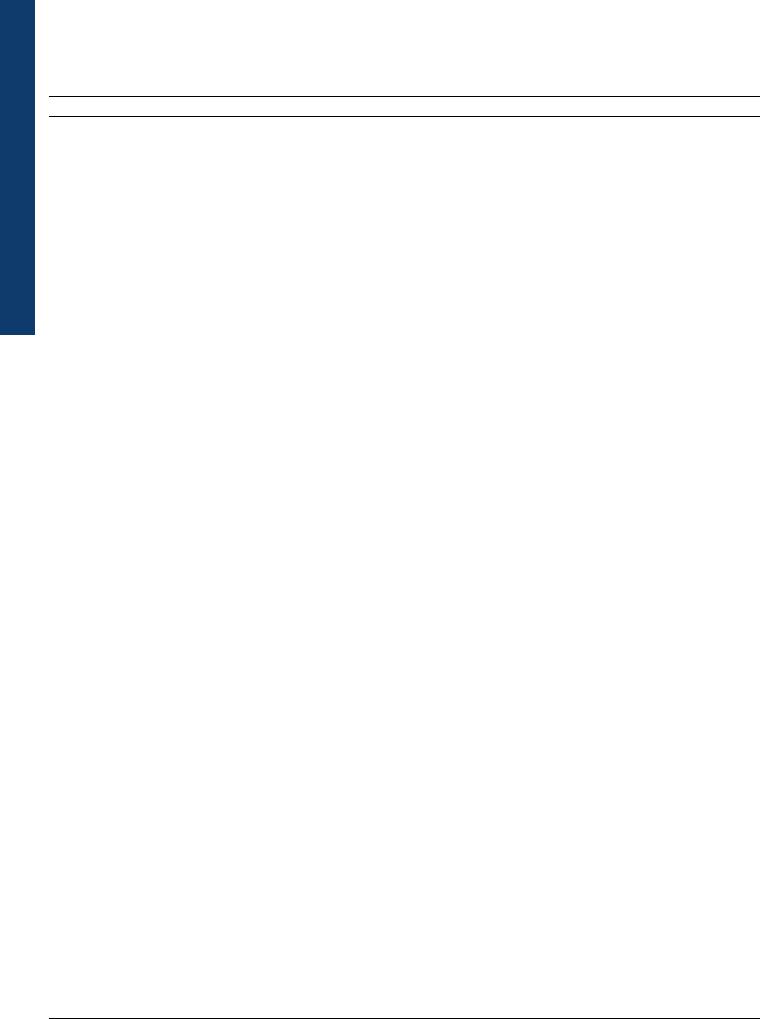
Review RESEARCH:
RESEARCH |
Materials Today Volume 19, Number 6 July/August 2016 |
TABLE 3
Glossary of proteins or cytokines appeared in this review.
Abbreviation |
Name |
Explanation |
|
|
|
TNFa |
Tumor necrosis factor |
Pro-inflammatory cytokines. |
IFNg |
Interferon g |
|
IL-1b, 6 |
Interleukin 1b, 6 |
|
IL-1ra, 10 |
Interleukin-1 receptor antagonist, 10 |
Anti-inflammatory cytokines. |
ROS |
Reactive oxygen species |
Natural byproducts from normal metabolism of oxygen |
NF-kB |
Nuclear factor kappa-light-chain-enhancer of activated B |
NF-kB is a major transcription factor that regulates genes |
|
cells |
responsible for the enhancement of inflammation. |
IkB-a |
Nuclear factor of kappa light polypeptide gene enhancer in |
The major function is to inhibit the NF-kB transcription factor. |
|
B-cells inhibitor, alpha |
|
RANTES |
Regulated on activation, normal T cell expressed and |
Chemokines, participate in cell migrations. |
|
secreted; also known as CCL5 |
|
ENA-78 |
Epithelial-derived neutrophil-activating peptide 78; also |
|
|
known as CXCL5 |
|
MCP-1 |
Monocyte chemotactic protein 1; also known as CCL2) |
|
Wnt5A |
Wingless-related MMTV integration site 5A |
Wnt5A/Ca2+ signaling pathway components. |
Fz5 |
Frizzled-5 |
|
CaMKII |
Calmodulin (CaM)-dependent protein kinase II |
|
CaSR |
Calcium sensing receptor |
CaSR signaling cascade leading to reduce inflammation. |
RANKL |
Receptor activator of nuclear factor kappa-B ligand |
RANKL, an osteoclastogenic factor, binding to RANK, signaling |
AP-1 |
Activator protein 1 |
through AP-1 and NFAT2 to enhance osteoclastogenesis. |
NFAT2 |
Nuclear factor of activated T cells 2 |
|
MyD88 |
Myeloid differentiation primary response gene 88 |
Toll-like receptor (TLR) pathway components. |
Ticam |
Toll-like receptor adaptor molecule |
|
HIFs |
Hypoxia-inducible factors |
Transcription factors that respond to hypoxia microenvironment. |
MCSF |
Macrophage colony-stimulating factor |
MCSF, an osteoclastogenic factor. MCST binds to c-FMS, signaling |
c-FMS |
Colony stimulating factor 1 receptor |
through AKT and MAPK to enhance osteoclastogenesis. |
AKT |
Protein Kinase B |
|
MAPK |
Mitogen-activated protein kinases |
|
OPG |
Osteoprotegerin |
A decoy receptor for RANKL, inhibiting osteoclastogenesis. |
TRAP |
Tartrate-resistant acid phosphatase |
TRAP is largely expressed in osteoclasts and activated |
|
|
macrophages. |
CTSK |
Cathepsin K |
It is a lysosomal cysteine protease involved in bone remodeling and |
|
|
resorption, which is expressed mainly in osteoclasts. |
CA 2 |
Carbonic Anhydrase II |
CA 2 plays an important role in osteoclast differentiation and bone |
|
|
resorption by regulating the homeostasis of intracellular pH and |
|
|
Ca2+. |
CT |
Calcitonin receptor |
CT binds the peptide hormone calcitonin, involving in bone |
|
|
formation and metabolism. |
MMP9 |
Matrix metalloproteinase-9 |
MMP9 is a protease involving in breaking down bone matrix. |
TGF b 1/3 |
Transforming growth factor |
Enhance the formation of fibrocapsule. |
VEGF |
Vascular endothelial growth factor |
An angiogenic factor. |
WNT10b |
Wingless-related MMTV integration site 10b |
A member of WNT family. Can activate canonical WNT pathway and |
|
|
enhance osteogenesis. |
BMP2/6 |
Bone morphogenetic protein 2/6 |
An osteogenic factor. |
BMPR2 |
Bone morphogenetic protein receptor type II |
BMP2 signaling pathway components |
BMPR1A |
Bone morphogenetic protein receptor, type IA |
|
SMAD 1/4/5 |
Mothers against decapentaplegic homolog 1/4/5 |
|
OSM |
Oncostatin M |
An inflammatory cytokine; also a regulator of osteoclastogenesis |
|
|
and osteogenesis. |
OSMR |
Oncostatin M receptor |
OSMR, A receptor of OSM. Signaling through gp130 and STAT3 to |
gp130 |
Glycoprotein 130 |
enhance osteogenesis. |
STAT3 |
Signal transducer and activator of transcription |
|
ALP |
Alkaline phosphatase |
ALP is a byproduct of osteoblast activity. ALP increases when active |
|
|
bone formation is occurring. |
OPN |
Osteopontin |
An early indicator of osteogenic differentiation. |
OCN |
Osteocalcin |
OCN is supposed to be expressed by more mature osteoblastic |
|
|
phenotypes. Therefore, it is a late indicator of osteogenic |
|
|
differentiation. |
COL1 |
Collagen type 1 |
COL1 is another marker of osteogenic differentiation, which will be |
|
|
increased when the osteogenesis is enhanced. |
IBSP |
Bone sialoprotein 2 |
IBSP is a significant component of the bone extracellular matrix. |
318
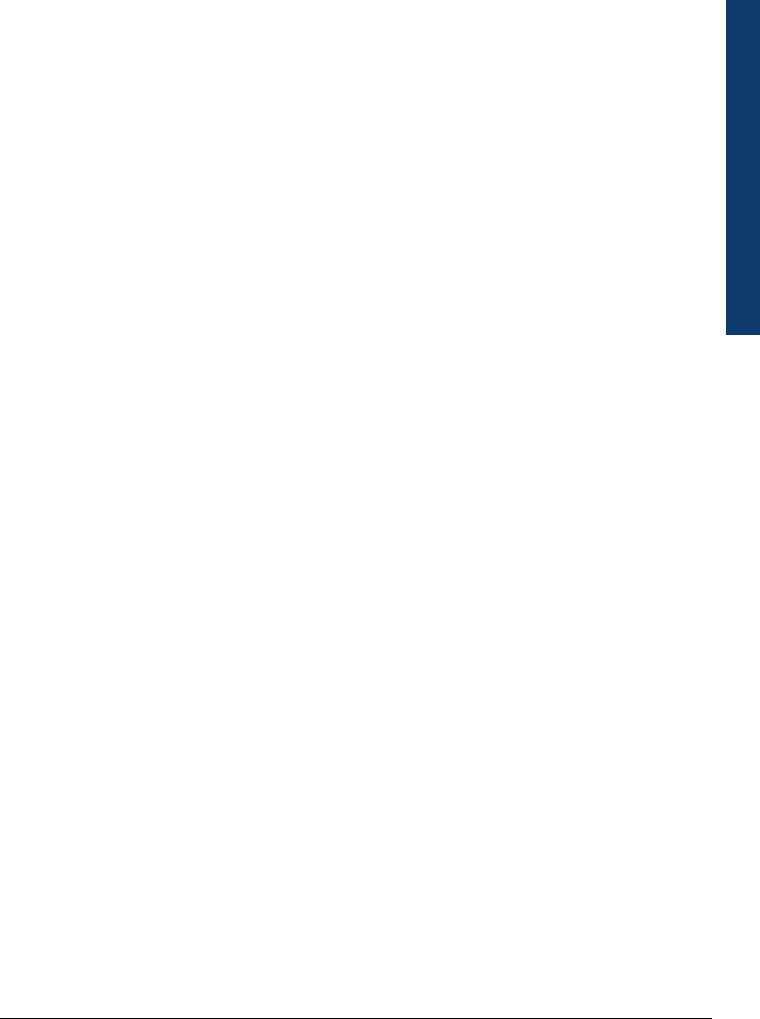
Materials Today Volume 19, Number 6 July/August 2016 |
RESEARCH |
response by manipulating the materials’ composition or structure. Potential strategies for such manipulations are (Table 2): (1) modifying the surface topology of materials (size and roughness) – material surfaces that provide biomimetic cues, such as nanoscale structures, have been found to regulate cell/biomaterial interactions [80,195]; (2) modifying the surface chemistry of materials (hydrophility and electric potential) – macrophages cultured on surfaces with different hydrophilicity results in different protein expression profiles and cytokine responses [66]; (3) changing the particles size – the immune response varies with the change of material sizes, even though the composition remains unchanged; (4) optimizing pore size and porosity – a uniform 30–40 mm pore size appears to promote the polarization toward the M2 phenotype [118], whereas non-porous or random-porous-sized materials appear to favor the M1 phenotype [196]; (5) incorporating nutrient elements
[148,197] – the immune response can be manipulated by a combination of bioactive elements (e.g. Ca, Mg, Sr, Co, Zn) in a controlled-release manner; (6) incorporating bioactive molecules
[198] such as macrophage inducers (e.g. IL-4, LPS) or inflammatory cytokines (e.g. IL-10, TNFa, IFNg); and (7) coupling with immunomodulatory drugs [199,200], for example inflammatory modulation drug (steroid and non-steroidal anti-inflammatory drugs). These strategies should all be considered when designing biomaterials, since their combination can have synergistic effects. Needless to say, a better understanding of the mechanisms underlying the immune response and their effects on osteoclastogenesis and osteogenesis is essential for developing advanced bone biomaterials with favorable OIM properties (Table 3).
Of these strategies, modifying the biomaterials with nutrient elements may have the greatest potential, not least because nutrient elements are fundamental for human physiology. The integration of nutrient elements into biomaterials has already been widely applied to modify materials in an effort to improve their bioactivity. For example, incorporating strontium into bioactive glasses inhibits osteoclast activity and enhances the osteogenesis and has, therefore, expanded the application of hydroxyapatite to patients with osteoporosis [177,201]. The scientific literature clearly reveals that nutrient elements can elicit significant effects on immune response regulation depending on the concentration and combination of the various elements. The implication of this is the possibility of finding an optimal combination of nutrient elements such that one can obtain an advanced bone biomaterial with multiple functions, one of which is OIM. Based on this strategy, we recently combined the elements Sr, Mg, and Si, to fabricate two novel bioceramic coating materials (Sr2MgSi2O7, MgSiO3). Both materials were found to endow the inert titanium substrate (Ti– 6Al–4V) with favorable OIM and reduce inflammation and osteoclastogenesis, while maintaining or enhancing osteogenic capacity compared with hydroxyapatite coated materials [148,202]. These are promising results that highlight the feasibility of this strategy and also that the design and preparation of bone biomaterials must be done with OIM properties in mind.
Conclusions
Bone biomaterials can determine an immune response. The type of response is related to the properties of the biomaterials, such as surface topography, particle size, porosity and pore size, and ion release. Components from the degraded materials and released
molecular signals from the interaction between immune system and implants, significantly affect the biological behaviors of bone cells, thereby determining the bone regeneration outcome. Accordingly, OIM is proposed to define this process, which involves the interaction between biomaterials, immune cells and bone cells, thus emphasizing the importance of immune cells during the biomaterial-mediated osteogenesis. Biomaterials with the appropriate OIM can create an immune environment that enhances osteogenesis, and regulates proper osteoclastogenesis that can participate in the bone remodeling and cell-mediated materials degradation. Such a property is important for the development of advanced immunomodulatory bone biomaterials. Biomaterials with poor OIM may cause excessive inflammation and lead to an imbalance of osteoclastogenesis over osteogenesis; such materials would, therefore, be excluded from further tests. Future studies should focus on determining the kind of immune environment that is conducive for osteogenesis and osseointegration, thus providing a set of design aims for the modification of bone biomaterials. Understanding how the biomaterials modulate the immune environment can guide the development of modification strategies, and integral to this enterprise is a systematic evaluation of modification strategies to explore an optimized modification strategy to endow advanced bone biomaterials with favorable OIM.
Acknowledgements
Funding for this study was provided by the Q-CAS Biotechnology Fund (GJHZ1505), Recruitment Program of Global Young Talent, China (C.W.), the National High Technology Research and Development Program of China (863 Program, SS2015AA020302), Natural Science Foundation of China (Grants 31370963, 81201202 and 81190132), Program of Shanghai Outstanding Academic Leaders (15XD1503900), Innovative Project of SIC, CAS, NHMRC (APP1032738) and ARC (DP120103697).
References
[1]O.S. Schindler, et al. Knee 14 (6) (2007) 458–464.
[2]E.A. Cook, J.J. Cook, Clin. Podiatr. Med. Surg. 26 (4) (2009) 589–605.
[3]J.S. Carson, M.P.G. Bostrom, Injury 38 (Suppl. 1) (2007) S33–S37.
[4]R. Oliveira, et al. Implant Dent. 21 (5) (2012) 422–426.
[5]J.R. Arron, Y. Choi, Nature 408 (6812) (2000) 535–536.
[6]H. Takayanagi, Nat. Rev. Immunol. 7 (4) (2007) 292–304.
[7]S. Franz, et al. Biomaterials 32 (28) (2011) 6692–6709.
[8]H. Takayanagi, J. Periodontal Res. 40 (4) (2005) 287–293.
[9]B.N. Brown, S.F. Badylak, Acta Biomater. 9 (2) (2013) 4948–4955.
[10]S.D. Kobayashi, et al. Arch. Immunol. Ther. Exp. (Warsz.) 53 (6) (2005) 505–517.
[11]J.M. Anderson, et al. Semin. Immunol. 20 (2) (2008) 86–100.
[12]L. Tang, et al. Proc. Natl. Acad. Sci. U. S. A. 95 (15) (1998) 8841–8846.
[13]Z. Xia, J.T. Triffitt, Biomed. Mater. 1 (1) (2006) R1–R9.
[14]P.H. Hart, et al. J. Leukoc. Biol. 66 (4) (1999) 575–578.
[15]J. Klein-Nulend, et al. Bone (2012).
[16]L.F. Bonewald, Bonekey Osteovision 3 (10) (2006) 7–15.
[17]Z. Chen, et al. J. Biomed. Mater. Res. A 102 (8) (2014) 2813–2823.
[18]M. Ma, et al. Adv. Mater. 23 (24) (2011) H189–H194.
[19]M. Yamashita, et al. Regul. Pept. 162 (1–3) (2010) 99–108.
[20]M. Zaidi, Nat. Med. 13 (7) (2007) 791–801.
[21]L.E. Theill, et al. Annu. Rev. Immunol. 20 (2002) 795–823.
[22]A. Chakravarti, et al. Blood 114 (8) (2009) 1633–1644.
[23]F. Yoshitake, et al. J. Biol. Chem. 283 (17) (2008) 11535–11540.
[24]M.H. Abdel Meguid, et al. Rheumatol. Int. 33 (3) (2013) 697–703.
[25]R.D. Devlin, et al. J. Bone Miner. Res. 13 (3) (1998) 393–399.
[26]P. Palmqvist, et al. J. Immunol. 169 (6) (2002) 3353–3362.
[27]I.G. Richardson, Cem. Concr. Compos. 22 (2) (2000) 97–113.
RESEARCH: Review
319
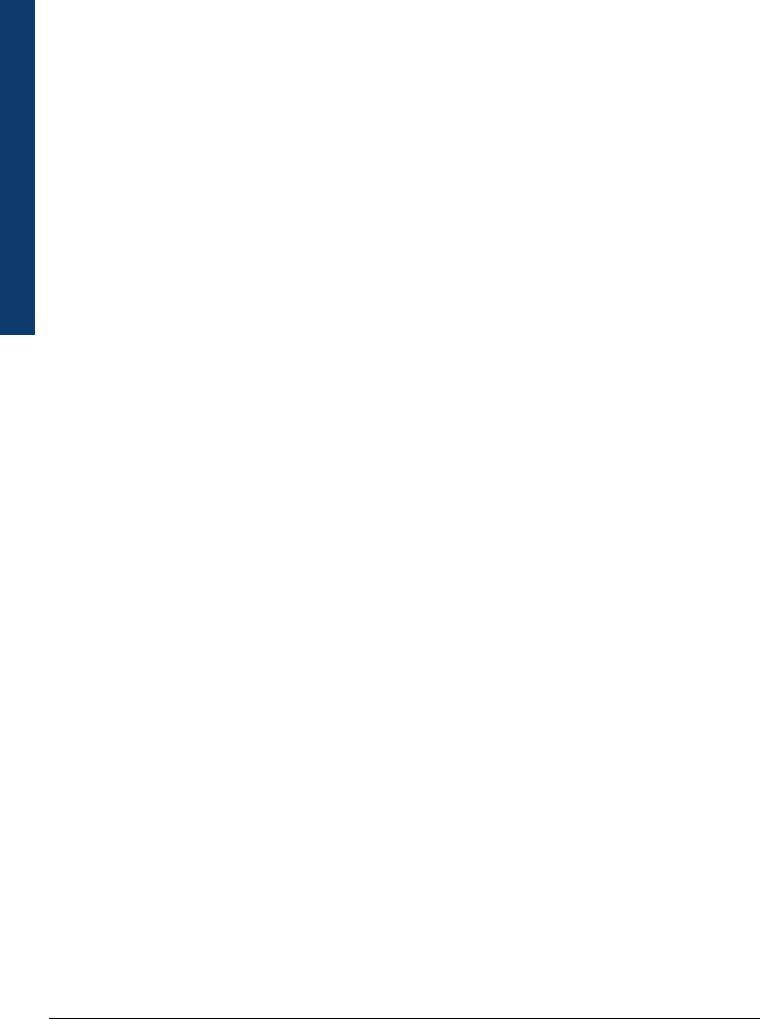
Review RESEARCH:
RESEARCH |
Materials Today Volume 19, Number 6 July/August 2016 |
[28]N.A. Sims, J.M.W. Quinn, BoneKEy Rep. 3 (2014).
[29]H.L. Wright, et al. Curr. Rev. Musculoskelet. Med. 2 (1) (2009) 56–64.
[30]B.F. Boyce, L. Xing, Arthritis Res. Ther. 9 (Suppl 1) (2007) S1.
[31]Y. Li, et al. Blood 109 (9) (2007) 3839–3848.
[32]R. Pacifici, Arch. Biochem. Biophys. 503 (1) (2010) 41–53.
[33]D. Grcevic, et al. J. Immunol. 165 (8) (2000) 4231–4238.
[34]I. Fouilloux, et al. Bone 38 (1) (2006) 59–66.
[35]N. Chiappetta, B. Gruber, Semin. Arthritis Rheum. 36 (1) (2006) 32–36.
[36]R. Silberstein, et al. Bone 12 (4) (1991) 227–236.
[37]L.A. Fitzpatrick, et al. Proc. Natl. Acad. Sci. U. S. A. 100 (10) (2003) 6027–6032.
[38]R. Gruber, Wien. Med. Wochenschr. 160 (17–18) (2010) 438–445.
[39]J. Caetano-Lopes, et al. Autoimmun. Rev. 8 (3) (2009) 250–255.
[40]M.K. Chang, et al. J. Immunol. 181 (2) (2008) 1232–1244.
[41]D. Toben, et al. J. Bone Miner. Res. 26 (1) (2011) 113–124.
[42]S. Reinke, et al. Sci. Transl. Med. 5 (177) (2013), 177ra136.
[43]A.R. Pettit, et al. Bone 43 (6) (2008) 976–982.
[44]Y. Honda, et al. Biochem. Biophys. Res. Commun. 345 (3) (2006) 1155–1160.
[45]S.M. Wahl, et al. Ann. N. Y. Acad. Sci. 593 (1990) 188–196.
[46]L. Rifas, J. Cell. Biochem. 98 (4) (2006) 706–714.
[47]K. Hess, et al. Bone 45 (2) (2009) 367–376.
[48]J. Ding, et al. Life Sci. 84 (15–16) (2009) 499–504.
[49]X. Yang, et al. Bone 41 (6) (2007) 928–936.
[50]P. Guihard, et al. Am. J. Pathol. 185 (3) (2015) 765–775.
[51]L. Gilbert, et al. Endocrinology 141 (11) (2000) 3956–3964.
[52]M. Feldmann, R.N. Maini, J. Immunol. 185 (2) (2010) 791–794.
[53]Y. Liu, et al. Nat. Med. 17 (12) (2011) 1594–1601.
[54]J. Chang, et al. Proc. Natl. Acad. Sci. U. S. A. 110 (23) (2013) 9469–9474.
[55]C.D. Mills, et al. J. Immunol. 164 (12) (2000) 6166–6173.
[56]D.M. Mosser, J.P. Edwards, Nat. Rev. Immunol. 8 (12) (2008) 958–969.
[57]P. Guihard, et al. Stem Cells 30 (4) (2012) 762–772.
[58]D.O. Freytes, et al. J. Cell. Biochem. 114 (1) (2013) 220–229.
[59]C.M. Champagne, et al. Bone 30 (1) (2002) 26–31.
[60]N. Mokarram, R.V. Bellamkonda, Ann. Biomed. Eng. 42 (2) (2014) 338–351.
[61]T. Lucas, et al. J. Immunol. 184 (7) (2010) 3964–3977.
[62]A. Remes, D.F. Williams, Biomaterials 13 (11) (1992) 731–743.
[63]R.D. Stout, et al. J. Immunol. 175 (1) (2005) 342–349.
[64]G. Raes, et al. J. Leukoc. Biol. 71 (4) (2002) 597–602.
[65]J.P. Edwards, et al. J. Leukoc. Biol. 80 (6) (2006) 1298–1307.
[66]J.A. Jones, et al. J. Biomed. Mater. Res. A 83 (3) (2007) 585–596.
[67]J.K. Yun, et al. J. Biomed. Mater. Res. 29 (2) (1995) 257–268.
[68]R.M. Boehler, et al. Biotechniques 51 (4) (2011) 239–240.
[69]A. Hezi-Yamit, et al. J. Biomed. Mater. Res. A 90 (1) (2009) 133–141.
[70]M.A. Dobrovolskaia, S.E. McNeil, Nat. Nanotechnol. 2 (8) (2007) 469–478.
[71]Y. Tan, et al. Hum. Gene Ther. 10 (13) (1999) 2153–2161.
[72]L. Weiss, Int. Rev. Cytol. 26 (1969) 63–105.
[73]B. Wojciak-Stothard, et al. Cell Biol. Int. 19 (6) (1995) 485–490.
[74]A.K. Refai, et al. J. Biomed. Mater. Res. A 70 (2) (2004) 194–205.
[75]J. Takebe, et al. J. Biomed. Mater. Res. A 64 (2) (2003) 207–216.
[76]J.M. Rice, et al. Biomaterials 24 (26) (2003) 4799–4818.
[77]G. Mendonca, et al. Biomaterials 29 (28) (2008) 3822–3835.
[78]M.J. Dalby, et al. Nat. Mater. 6 (12) (2007) 997–1003.
[79]N.E. Paul, et al. Biomaterials 29 (30) (2008) 4056–4064.
[80]B.S. Smith, et al. Biomater. Sci. 1 (3) (2013) 322–332.
[81]P. Roach, et al. J. Am. Chem. Soc. 127 (22) (2005) 8168–8173.
[82]C.J. Wilson, et al. Tissue Eng. 11 (1–2) (2005) 1–18.
[83]L.C. Xu, C.A. Siedlecki, Biomaterials 28 (22) (2007) 3273–3283.
[84]C.E. Giacomelli, et al. J. Colloid Interface Sci. 220 (1) (1999) 13–23.
[85]W.J. Hu, et al. Blood 98 (4) (2001) 1231–1238.
[86]B. Nilsson, et al. Mol. Immunol. 44 (1–3) (2007) 82–94.
[87]J.V. Sarma, P.A. Ward, Cell Tissue Res. 343 (1) (2011) 227–235.
[88]J. Andersson, et al. J. Immunol. 168 (11) (2002) 5786–5791.
[89]B.G. Keselowsky, et al. Biomaterials 28 (25) (2007) 3626–3631.
[90]C.D. McFarland, et al. J. Biomed. Mater. Res. 49 (2) (2000) 200–210.
[91]A.K. McNally, et al. J. Biomed. Mater. Res. A 86 (2) (2008) 535–543.
[92]A.K. McNally, J.M. Anderson, Adv. Exp. Med. Biol. 713 (2011) 97–111.
[93]B.G. Keselowsky, et al. Proc. Natl. Acad. Sci. U. S. A. 102 (17) (2005) 5953–5957.
[94]L. Tang, J.W. Eaton, J. Exp. Med. 178 (6) (1993) 2147–2156.
[95]A.K. McNally, J.M. Anderson, Proc. Natl. Acad. Sci. U. S. A. 91 (21) (1994) 10119–10123.
[96]G. Berton, C.A. Lowell, Cell. Signal. 11 (9) (1999) 621–635.
[97]A.K. McNally, J.M. Anderson, Am. J. Pathol. 160 (2) (2002) 621–630.
[98]L. Zhang, et al. J. Biol. Chem. 287 (9) (2012) 6177–6186.
[99]A.M.B. Collie, et al. J. Biomed. Mater. Res. A 96A (1) (2011) 162–169.
[100]H.S. Lee, et al. J. Biomed. Mater. Res. A 101 (1) (2013) 203–212.
[101]J.A. Cooper, J. Cell Biol. 105 (4) (1987) 1473–1478.
[102]A.E. Nel, et al. Nat. Mater. 8 (7) (2009) 543–557.
[103]A. Aderem, D.M. Underhill, Annu. Rev. Immunol. 17 (1999) 593–623.
[104]K. Hirota, et al. Colloids Surf. B: Biointerfaces 87 (2) (2011) 293–298.
[105]K. Hirota, H. Terada, Endocytosis of particle formulations by macrophages and its application to clinical treatment, 2012.
[106]W.K. Oh, et al. ACS Nano 4 (9) (2010) 5301–5313.
[107]P. Laquerriere, et al. Biomaterials 24 (16) (2003) 2739–2747.
[108]B.R. Cuenya, Thin Solid Films 518 (12) (2010) 3127–3150.
[109]M.O. Oyewumi, et al. Expert Rev. Vaccines 9 (9) (2010) 1095–1107.
[110]O. Malard, et al. J. Biomed. Mater. Res. 46 (1) (1999) 103–111.
[111]V. Karageorgiou, D. Kaplan, Biomaterials 26 (27) (2005) 5474–5491.
[112]M.W. Laschke, et al. Tissue Eng. 12 (8) (2006) 2093–2104.
[113]U. Klinge, et al. J. Surg. Res. 103 (2) (2002) 208–214.
[114]Y. Kuboki, et al. Connect. Tissue Res. 43 (2–3) (2002) 529–534.
[115]K. Junge, et al. Langenbecks Arch. Surg. 397 (2) (2012) 255–270.
[116]D. Weyhe, et al. World J. Surg. 30 (8) (2006) 1586–1591.
[117]K. Garg, et al. Biomaterials 34 (18) (2013) 4439–4451.
[118]A.J. Marshall, et al. Abstr. Pap. Am. Chem. S. 228 (2004) U386.
[119]L.R. Madden, et al. Proc. Natl. Acad. Sci. U. S. A. 107 (34) (2010) 15211–15216.
[120]M. Bohner, et al. J. Eur. Ceram. Soc. 32 (11) (2012) 2663–2671.
[121]Y.-W. Chen, et al. J. Mater. Chem. B 3 (35) (2015) 7099–7108.
[122]B.C. Wu, et al. J. Endod. 40 (8) (2014) 1105–1111.
[123]C.J. Hung, et al. J. Endod. 39 (12) (2013) 1557–1561.
[124]A. De, Acta Biochim. Biophys. Sin. 43 (10) (2011) 745–756.
[125]R.J. MacLeod, et al. Am. J. Physiol. Gastrointest. Liver Physiol. 293 (1) (2007) G403–G411.
[126]A. De, Acta Biochem. Biophys. Sin. 43 (10) (2011) 745–756.
[127]X. Zhou, et al. Immunology 143 (2) (2014) 287–299.
[128]E.M. Carlisle, Nutr. Rev. 40 (7) (1982) 193–198.
[129]E.M. Carlisle, Ciba Found. Symp. 121 (1986) 123–139.
[130]E.M. Carlisle, Science 167 (916) (1970) 279–280.
[131]E.M. Carlisle, Nutr. Rev. 40 (7) (1982) 210–213.
[132]F.H. Nielsen, R. Poellot, J. Trace Elem. Exp. Med. 17 (3) (2004) 137–149.
[133]D.M. Reffitt, et al. Bone 32 (2) (2003) 127–135.
[134]A.M. Pietak, et al. Biomaterials 28 (28) (2007) 4023–4032.
[135]P.E. Keeting, et al. J. Bone Miner. Res. 7 (11) (1992) 1281–1289.
[136]I.D. Xynos, et al. Biochem. Biophys. Res. Commun. 276 (2) (2000) 461–465.
[137]I.D. Xynos, et al. J. Biomed. Mater. Res. 55 (2) (2001) 151–157.
[138]C.T. Wu, et al. J. Mater. Chem. B 1 (6) (2013) 876–885.
[139]A.K. Gaharwar, et al. Adv. Mater. 25 (24) (2013) 3329–3336.
[140]Y. Chen, et al. Toxicol. Ind. Health 20 (1–5) (2004) 21–27.
[141]S.S. Teuber, et al. Biol. Trace Elem. Res. 48 (2) (1995) 121–130.
[142]K.M. Ainslie, et al. ACS Nano 2 (5) (2008) 1076–1084.
[143]M.P. Staiger, et al. Biomaterials 27 (9) (2006) 1728–1734.
[144]J. Wang, et al. J. Biomed. Mater. Res. B: Appl. Biomater. 100 (6) (2012) 1691–1701.
[145]J. Sugimoto, et al. J. Immunol. 188 (12) (2012) 6338–6346.
[146]J.I. Pearl, et al. Biomaterials 32 (24) (2011) 5535–5542.
[147]T. Kawai, S. Akira, Nat. Immunol. 11 (5) (2010) 373–384.
[148]C. Wu, et al. ACS Appl. Mater. Interfaces 6 (6) (2014) 4264–4276.
[149]H. Zhou, et al. Biomaterials 33 (29) (2012) 6933–6942.
[150]D. Shweiki, et al. Nature 359 (6398) (1992) 843–845.
[151]T.S. Li, et al. Am. J. Physiol. Heart Circ. Physiol. 283 (2) (2002) H468–H473.
[152]C. Wu, et al. Biomaterials 33 (7) (2012) 2076–2085.
[153]M. Zhang, et al. J. Mater. Chem. 22 (40) (2012) 21686–21694.
[154]J.M. Smith, et al. J. Mater. Chem. B 1 (9) (2013) 1296–1303.
[155]M. Azevedo, et al. J. Mater. Chem. 20 (40) (2010) 8854–8864.
[156]T. Cramer, et al. Cell 112 (5) (2003) 645–657.
[157]T. Oda, et al. Am. J. Physiol. Cell Physiol. 291 (1) (2006) C104–C113.
[158]C.C. Blouin, et al. Blood 103 (3) (2004) 1124–1130.
[159]T. Hellwig-Burgel, et al. Blood 94 (5) (1999) 1561–1567.
[160]D.J. Langton, et al. J. Bone Joint Surg. Br. 92 (1) (2010) 38–46.
[161]Z. Chen, et al. J. Arthroplasty 29 (3) (2014) 612–616.
[162]E.M. Evans, et al. J. Bone Joint Surg. Br. 56B (4) (1974) 626–642.
[163]M. Yamaguchi, J. Trace Elem. Exp. Med. 11 (2–3) (1998) 119–135.
[164]J.K. Park, et al. Adv. Mater. 22 (43) (2010) 4857–4861.
[165]H.S. Hsieh, J.M. Navia, J. Nutr. 110 (8) (1980) 1581–1588.
[166]J.T. Kim, et al. J. Med. Food 12 (1) (2009) 118–123.
[167]Z. Chen, et al. J. Mater. Chem. B 2 (36) (2014) 6030–6043.
[168]A. Grandjean-Laquerriere, et al. Biomaterials 27 (17) (2006) 3195–3200.
320
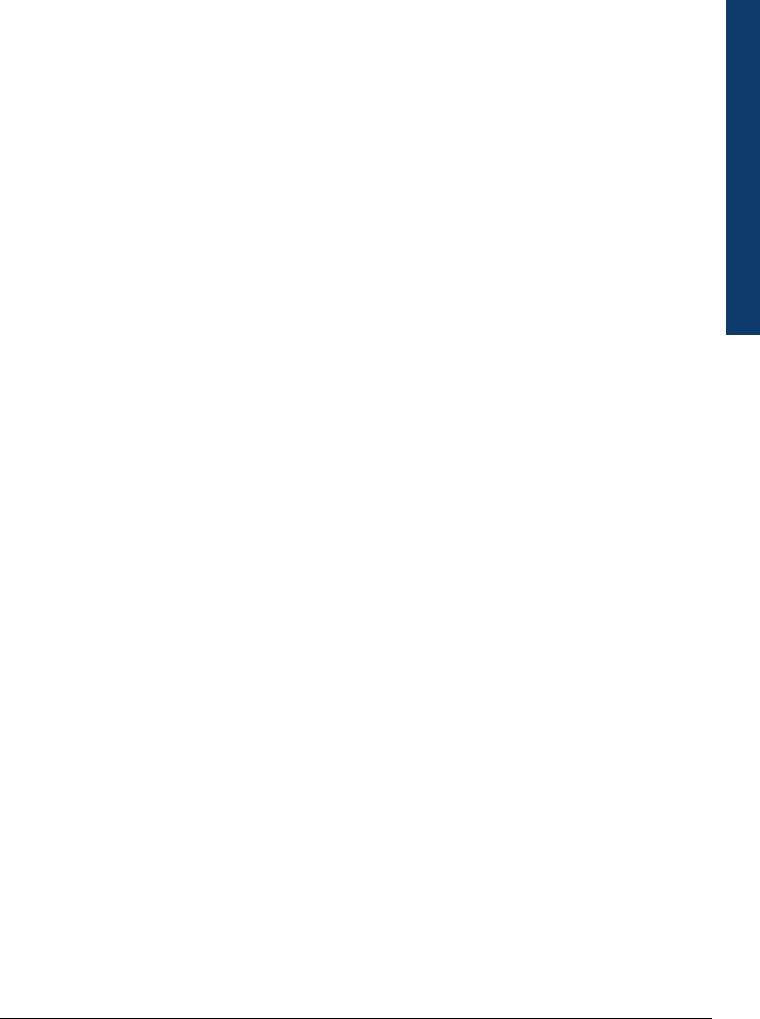
Materials Today Volume 19, Number 6 July/August 2016 |
RESEARCH |
[169] R.M. Day, A.R. Boccaccini, J. Biomed. Mater. Res. A 73 (1) (2005) 73–79. |
[186] R.J. OKeefe, et al. Lab. Invest. 76 (4) (1997) 457–465. |
[170] H. Haase, L. Rink, Biometals 20 (3–4) (2007) 579–585. |
[187] Q. Zhang, et al. Biol. Chem. 283 (19) (2008) 13491–13499. |
[171] F. Velard, et al. Acta Biomater. 9 (2) (2013) 4956–4963. |
[188] D.J. Prockop, J.Y. Oh, Mol. Ther. 20 (1) (2012) 14–20. |
[172] P. Connell, et al. J. Am. Coll. Nutr. 16 (5) (1997) 411–417. |
[189] J. Kim, P. Hematti, Exp. Hematol. 37 (12) (2009) 1445–1453. |
[173] A. Zoli, et al. Clin. Rheumatol. 17 (5) (1998) 378–382. |
[190] J.H. Ylostalo, et al. Stem Cells 30 (10) (2012) 2283–2296. |
[174] P. Scuderi, Cell Immunol. 126 (2) (1990) 391–405. |
[191] K. Nemeth, et al. Nat. Med. 15 (1) (2009) 42–49. |
[175] E. Shorr, A.C. Carter, Bull. Hosp. Jt. Dis. 13 (1) (1952) 59–66. |
[192] L.A. Ortiz, et al. Proc. Natl. Acad. Sci. U. S. A. 104 (26) (2007) 11002–11007. |
[176] C. Wu, et al. Acta Biomater. 8 (2012) 3805–3815. |
[193] J.Y. Oh, et al. Proc. Natl. Acad. Sci. U. S. A. 107 (39) (2010) 16875–16880. |
[177] Y.F. Zhang, et al. J. Mater. Chem. B 1 (41) (2013) 5711–5722. |
[194] Y. Miki, et al. J. Steroid Biochem. Mol. Biol. 131 (3–5) (2012) 68–75. |
[178] M. Yamaguchi, M.N. Weitzmann, Mol. Cell. Biochem. 359 (1–2) (2012) 399–407. |
[195] J.J. Moon, et al. Adv. Mater. 24 (28) (2012) 3724–3746. |
[179] C. Cardemil, et al. PLoS ONE 8 (12) (2013) e84932. |
[196] V.E. Wagner, J.D. Bryers, J. Biomed. Mater. Res. A 69A (1) (2004) 79–90. |
[180] E. Buache, et al. Acta Biomater. 8 (8) (2012) 3113–3119. |
[197] Z. Chen, et al. J. Mater. Chem. B (2014). |
[181] P. Romer, et al. Ann. Anat. 194 (2) (2012) 208–211. |
[198] G. Wu, et al. Biomaterials 31 (29) (2010) 7485–7493. |
[182] Z. Chen, et al. Biomaterials 61 (2015) 126–138. |
[199] H. Nyangoga, et al. Acta Biomater. 6 (8) (2010) 3310–3317. |
[183] A.K. Malekshah, et al. Indian J. Exp. Biol. 44 (3) (2006) 189–192. |
[200] J.S. Son, et al. J. Biomed. Mater. Res. A 99 (4) (2011) 638–647. |
[184] J. Zhu, et al. Blood 109 (9) (2007) 3706–3712. |
[201] E. Gentleman, et al. Biomaterials 31 (14) (2010) 3949–3956. |
[185] Y.L. Wu, et al. Autoimmunity 41 (3) (2008) 183–194. |
[202] C. Wu, et al. Biomaterials 71 (2015) 35–47. |
RESEARCH: Review
321