
Human_Histology
.pdf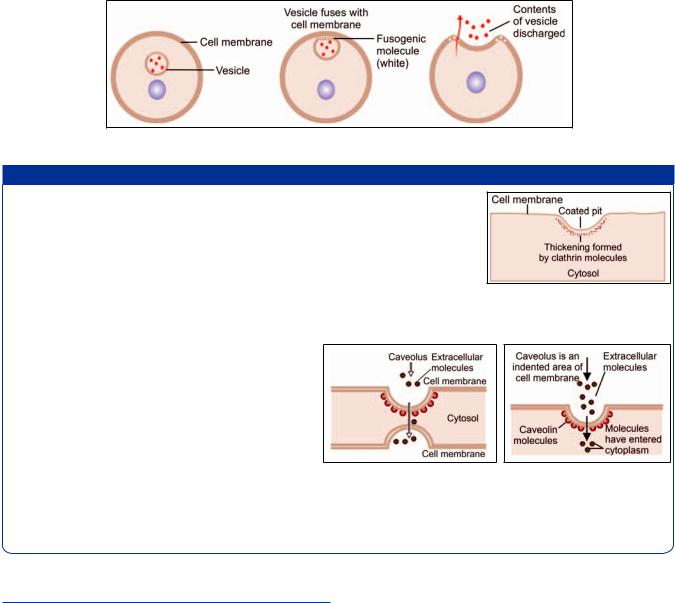
8Textbook of Human Histology
Fig. 2.6: Three stages in exocytosis. The fusogenic proteins facilitate adhesion of the vesicle to the cell membrane (Schematic representation)
Added Information
As endocytic vesicles are derived from cell membrane, and as exocytic vesicles fuse with the later, there is a constant transfer of membrane material between the surface of the cell and vesicles within the cell.
Areas of cell membrane which give origin to endocytic vesicles are marked by the presence of fusogenic proteins that aid the formation of endocytic vesicles. Fusogenic proteins also help in exocytosis by facilitating fusion of membrane surrounding vesicles with the cell membrane.
When viewed by EM, areas of receptor mediated endocytosis are seen as depressed areas called coated pits ! ! thickened because of the presence of a protein called clathrin. This protein forms a scaffolding around the developing vesicle and facilitates
its separation from the cell membrane. Thereafter, the clathrin molecules detach from the surface of the vesicle and return to the cell membrane.
The term transcytosis refers to a process where material is transferred right through the thickness of a cell. The! ! The transport takes place through invaginations of cell membrane called caveolae. A protein caveolin is associated with caveolae (Fig. 2.8). Caveolae differ from coated pits in that they are not transformed into vesicles. Caveolae also play a role in transport of extracellular molecules to the cytosol (without formation of vesicles) (Fig. 2.9).
Fig. 2.7: Coated pit as seen by electron microscope in cell membrane
(Schematic representation)
Fig. 2.9: How extracellular molecules enter the cytosol through caveolae. Endocytic vesicles are not formed. The process is called potocytosis (Schematic representation)
CONTACTS BETWEEN ADJOINING CELLS
In tissues in which cells are closely packed, the cell membranes of adjoining cells are separated over most of their extent by a narrow space (about 20 nm). This contact is sufficient to bind cells loosely together, and also allows some degree of movement of individual cells.
In some regions the cell membranes of adjoining cells come into more intimate contact. These areas can be classified as follows:
Classification of Cell Contacts
Unspecialized Contacts
These are contacts that do not show any specialized features on EM examination. At such sites adjoining cell membranes are held together by some glycoprotein
molecules, present in the cell membrane, called as cell adhesion molecules (CAMs). These molecules occupy the entire thickness of the cell membrane (i.e. they are transmembrane proteins). At its cytosolic end, each CAM is in contact with an intermediate protein (or link protein) (that appears to hold the CAM in place). Fibrous elements of the cytoskeleton are attached to this intermediate protein (and thus indirectly to CAMs). The other end of the CAM juts into the 20 nm intercellular space, and comes in contact with a similar molecule from the opposite cell membrane. In this way a path is established through which forces can be transmitted from the cytoskeleton of one cell to another (Fig. 2.10).
CAMs and intermediate proteins are of various types. Contacts between cells can be classified on the basis of the type of CAMs proteins present. The adhesion of some
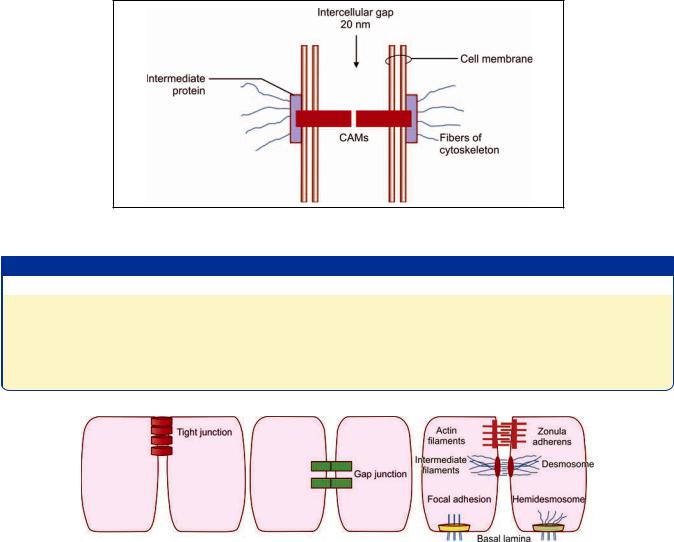
Chapter 2 Cell Structure 9
Fig. 2.10: Basic structure of an unspecialized contact between two cells (Schematic representation)
Table 2.1: Types of cell adhesion molecules (CAM)
Type of CAM |
Subtypes |
Present in |
||
Calcium dependent |
Cadherins (of various types) |
Most cells including epithelia |
||
|
|
Selectins |
Migrating cells, e.g. Leucocytes |
|
|
|
Integrins |
Between cells and intercellular substances. About 20 types of |
|
|
|
|
integrins, each attaching to a special extracellular molecule |
|
Calcium independent |
Neural cell adhesion molecule (NCAM) |
Nerve cells |
||
|
|
Intercellular adhesion molecule (ICAM) |
Leucocytes |
|
|
|
|
|
|
|
|
|
|
|
Fig. 2.11: Various types of specialized junctions (Schematic representation)
CAMs is dependent on the presence of calcium ions; while some others are not dependent on them (Table 2.1). Intermediate proteins are also of various types (catenins, vinculin, -actinin, etc.).
Specialized Junctional Structures
These junctions can be recognized by EM. The basic mode of intercellular contact in them is similar to that described above and involves CAMs, intermediate proteins and cytoskeletal elements.
Types of Specialized Junctions (Fig. 2.11)
Anchoring junctions or adhesive junctions bind cells together mechanically to their neighboring cells. They can be of the following types:
Adhesive spots (also called desmosomes or maculae adherens).
Adhesive belts or zonula adherens.
Adhesive strips or fascia adherens.
Modified anchoring junctions attach cells to extracellular material. Such junctions are seen as hemidesmosomes or as focal spots.
Occluding junctions (zonula occludens or tight junctions). Apart from holding cells together, these junctions form barriers to movement of material through intervals between cells.
Communicating junctions (or gap junctions). Such junctions allow direct transport of some substances from cell to cell.
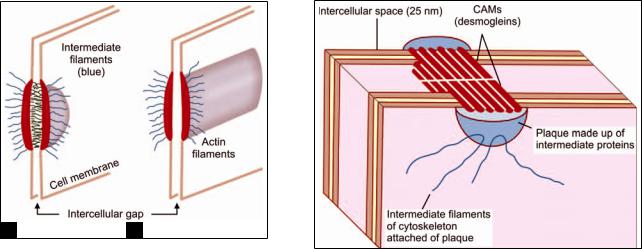
10 Textbook of Human Histology
A B
Figs. 2.12A amd B: (A) Electron microscope appearance of a desmosome; (B) Electron microscope appearance of zonula adherens (Schematic representation)
Fig. 2.13: Detailed structure of a desmosome (in the epidermis) (Schematic representation)
Anchoring Junctions
Adhesion Spots (Desmosomes, Macula Adherens)
These are the most common type of junctions between adjoining cells. Desmosomes are present where strong anchorage between cells is needed, e.g. between cells of the epidermis.
As seen by EM, a desmosome is a small circumscribed area of attachment (Figs. 2.12A and B). At the site of a desmosome, the plasma membrane (of each cell) is thickened because of the presence of a dense layer of proteins on its inner surface (i.e. the surface toward the cytoplasm).
The thickened areas of the two sides are separated by a gap of 25 nm. The region of the gap is rich in glycoproteins. The thickened areas of the two membranes are held together by fibrils that appear to pass from one membrane to the other across the gap. We now know that the fibrils seen in the intercellular space represent CAMs (Fig. 2.13).
The thickened area (or plaque) seen on the cytosolic aspect of the cell membrane is produced by the presence of intermediate (link) proteins. Cytoskeletal filaments attached to the thickened area are intermediate filaments. CAMs seen in desmosomes are integrins (desmogleins I, II). The link proteins are desmoplakins.
Adhesive Belts (Zonula Adherens)
In some situations, most typically near the apices of epithelial cells, we see a kind of junction called the zonula adherens or adhesive belt (Fig. 2.12B). This is similar to a desmosome in being marked by thickenings of the two plasma membranes, to the cytoplasmic aspects of which
fibrils are attached. However, the junction differs from a desmosome as follows:
Instead of being a small circumscribed area of attachment, the junction is in the form of a continuous band passing all around the apical part of the epithelial cell.
The gap between the thickenings of the plasma membranes of the two cells is not traversed by filaments. The CAMs present are cadherins. In epithelial cells, zona
adherens are located immediately deep to occluding junctions (see later).
Adhesive Strips (Fascia Adherens)
These are similar to adhesive belts. They differ from the latter in that the areas of attachment are in the form of short strips (and do not go all round the cell). These are seen in relation to smooth muscle, intercalated discs of cardiac muscle, and in junctions between glial cells and nerves.
Hemidesmosomes
These are similar to desmosomes, but the thickening of cell membrane is seen only on one side. At such junctions, the “external” ends of CAMs are attached to extracellular structures. Hemidesmosomes are common where basal epidermal cells lie against connective tissue.
The cytoskeletal elements attached to intermediate proteins are keratin filaments (as against intermediate filaments in desmosomes). As in desmosomes, the CAMs are integrins.
Focal Spots
These are also called focal adhesion plaques, or focal contacts. They represent areas of local adhesion of a cell to extracellular matrix. Such junctions are of a transient
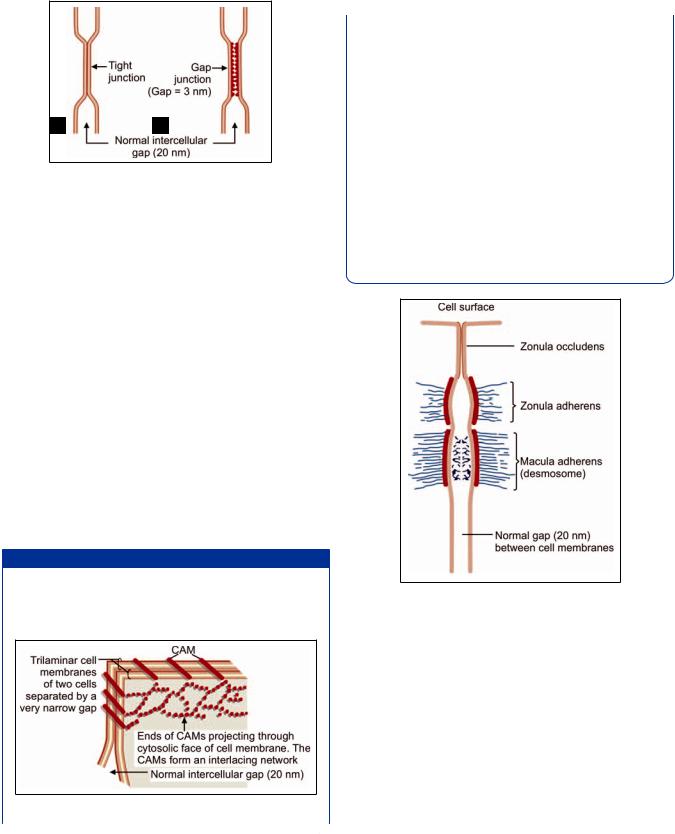
AB
Figs. 2.14A and B: (A) Zonula occludens as seen by electron microscope; (B) Gap junction as seen by electron microscope (Schematic representation)
nature (e.g. between a leucocyte and a vessel wall). Such contacts may send signals to the cell and initiate cytoskeletal formation.
The CAMs in focal spots are integrins. The intermediate proteins (that bind integrins to actin filaments) are-actinin, vinculin, and talin.
Occluding Junctions (Zonula Occludens)
Like the zonula adherens, the zonula occludens are seen most typically near the apices of epithelial cells. At such a junction the two plasma membranes are in actual contact (Fig. 2.14A).
These junctions not only bind the cells to each other but also act as barriers that prevent the movement of molecules into the intercellular spaces. For example, they prevent intestinal contents from permeating into the intercellular spaces between the lining cells by virtue of occluding junctions. Zonulae occludens are, therefore, also called tight junctions (Fig. 2.14A).
Added Information
Recent studies have provided a clearer view of the structure of tight junctions (Fig. 2.15). Adjoining cell membranes are united by CAMs that are arranged in the form of a network that “stitches” the two membranes together.
Fig. 2.15: Detailed structure of part of an occluding junction (Schematic representation)
Contd...
Chapter 2 Cell Structure 11
Contd...
Other functions attributed to occluding junctions are as follows:
These junctions separate areas of cell membrane that are specialized for absorption or secretion (and lie on the luminal side of the cell) from the rest of the cell membrane.
Areas of cell membrane performing such functions bear specialized proteins. Occluding junctions prevent lateral migration of such proteins.
In cells involved in active transport against a concentration gradient, occluding junctions prevent back diffusion of transported substances.
Apart from epithelial cells, zonulae occludens are also present between endothelial cells.
In some situations occlusion of the gaps between the adjoining cells may be incomplete and the junction may allow slow diffusion of molecules across it. These are referred to as leaky tight junctions.
Fig. 2.16: A junctional complex (Schematic representations)
Junctional Complex
Near the apices of epithelial cells the three types of junctions described above, namely zonula occludens, zonula adherens and macula adherens are often seen arranged in that order (Fig. 2.16). They collectively form a junctional complex. In some complexes, the zonula occludens may be replaced by a leaky tight junction, or a gap junction.
Communicating Junctions (Gap Junctions)
At these junctions, the plasma membranes are not in actual contact (as in a tight junction), but lie very close to each other; the gap being reduced (from the normal 20 nm to 3 nm) (Fig. 2.14B). In transmission electronmicrographs
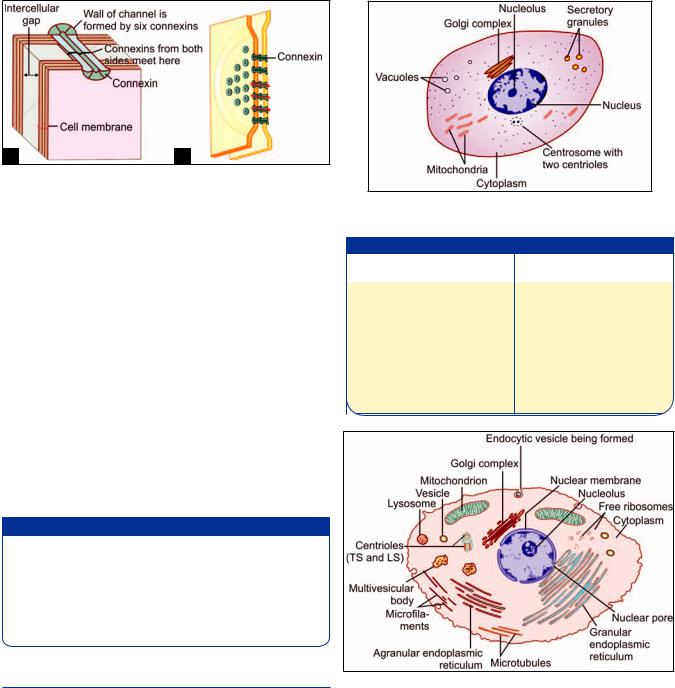
12 Textbook of Human Histology
A B
Figs. 2.17A and B: A communicating junction (gap junction). (A) As seen under electron microscope; (B) To show the constitution of one channel of a communicating junction (Schematic representation)
this gap is seen to contain bead-like structures (Fig. 2.17A). A minute canaliculus passing through each “bead” connects the cytoplasm of the two cells thus allowing the free passage of some substances (sodium, potassium, calcium, metabolites) from one cell to the other. Gap junctions are, therefore, also called maculae communicantes. They are widely distributed in the body.
The wall of each channel is made up of six protein elements (called nexins, or connexons). The “inner” ends of these elements are attached to the cytosolic side of the cell membrane while the “outer” ends project into the gap between the two cell membranes (Fig. 2.17B). Here they come in contact with (and align perfectly with) similar connexins projecting into the space from the cell membrane of the opposite cell to complete the channel.
Added Information
Changes in pH or in calcium ion concentration can close the channels of gap junctions. By allowing passing of ions they lower transcellular electrical resistance. Gap junctions form electrical synapses between some neurons.
The number of channels present in a gap junction can vary considerably.
CELL ORGANELLES
The cytoplasm of a typical cell contains various structures that are referred to as organelles. They include the endoplasmic reticulum (ER), ribosomes, mitochondria, the Golgi complex and various types of vesicles (Fig. 2.18).
The cell organelles can be membrane bound or without membrane (Table 2.2). The cytosol also contains a cytoskeleton made up of microtubules, microfilaments and intermediate filaments. Centrioles are closely connected with microtubules.
Fig. 2.18: Some features of a cell that can be seen with a light microscope (Schematic representation)
Table 2.2: Cell organelles
Membrane bound cell |
Non-membranous cell |
||
organelles |
organelles |
||
• |
Endoplasmic reticulum (ER) |
• |
Cytoskeleton including |
• |
Golgi complex |
|
" # ! |
• |
Mitochondria |
|
– Microtubules |
• |
Membrane bound vesicles |
|
" $ ! ! |
|
including |
• |
Ribosomes |
–Phagosomes
–Lysosomes
–Peroxisomes
–Exocytic vesicles
Fig. 2.19: Various organelles found in a typical cell
(Schematic representation)
Endoplasmic Reticulum
It is a network of interconnecting membranes enclosing channels or cisternae, that are continuous from outer nuclear envelope to outer plasma membrane (Fig. 2.19).
Because of the presence of the endoplasmic reticulum (ER) the cytoplasm is divided into two components, one within the channels and one outside them. The cytoplasm
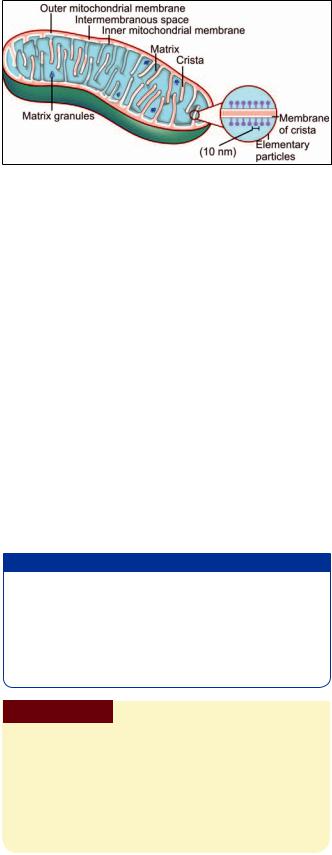
within the channels is called the vacuoplasm, and that outside the channels is the hyaloplasm or cytosol.
The proteins, glycoproteins and lipoproteins are synthesized in the ER. ER is very prominent in cells actively synthesizing proteins, e.g. immunoglobulin secreting plasma cells.
According to the EM appearance, the ER is generally classified into rough (granular) and smooth (agranular) varieties (see Fig. 2.19). The rough appearance of rough ER is due to ribosomes attached to cytoplasmic side of membrane which play an important role in protein synthesis. It is a prominent feature of cells synthesizing proteins. The lumen of rough ER is continuous with the perinuclear space (between the inner and outer nuclear membranes). It is also continuous with the lumen of smooth ER.
Smooth ER is responsible for further processing of proteins synthesized in rough ER. It is also responsible for synthesis of lipids, specially that of membrane phospholipids (necessary for membrane formation). Most cells have very little smooth ER. It is a prominent feature of cells processing lipids.
Products synthesized by the ER are stored in the channels within the reticulum. Ribosomes and enzymes are present on the “outer” surfaces of the membranes of the reticulum.
Ribosomes
As discussed, ribosomes are present in relation to rough endoplasmic reticulum. They may also lie free in the cytoplasm. They may be present singly in which case they are called monosomes; or in groups which are referred to as polyribosomes (or polysomes). Each ribosome consists of proteins and RNA (ribonucleic acid) and is about 15 nm in diameter. The ribosome is made up of two subunits small (40S) and large (60S) classified on the basis of their sedimentation rates. Ribosomes play an essential role in protein synthesis.
Mitochondria
Mitochondria can be seen with the light microscope in specially stained preparations (see Fig. 2.18). They are so called because they appear either as granules or as rods (mitos = granule; chondrium = rod).
The number of mitochondria varies from cell to cell being greatest in cells with high metabolic activity (e.g. in secretory cells and sperms). Erythrocytes do not contain mitochondria.
Mitochondria vary in size, most of them being 0.5–2 μm in length. Mitochondria are large in cells with a high oxidative metabolism.
A schematic presentation of some details of the structure of a mitochondrion (as seen by EM) is shown
Chapter 2 Cell Structure 13
Fig. 2.20: Structure of a mitochondrion (Schematic representation)
in Figure 2.20. The mitochondrion is bounded by a smooth outer membrane within which there is an inner membrane; the two being separated by an intermembranous space. The inner membrane is highly folded on itself forming incomplete partitions called cristae. The space bounded by the inner membrane is filled by a granular material called the matrix. This matrix contains numerous enzymes. It also contains some RNA and dioxyribonucleic acid (DNA). These are believed to carry information that enables mitochondria to duplicate themselves during cell division. An interesting fact, discovered recently, is that all mitochondria are derived from those in the fertilized ovum and are entirely of maternal origin.
Mitochondria are of great functional importance. It is the power house of the cell. It contains many enzymes including some that play an important part in Kreb’s cycle [tricarboxylic acid (TCA) cycle]. Adenosine triphosphate (ATP) and guanosine triphosphate (GTP) are formed in mitochondria from where they pass to other parts of the cell and provide energy for various cellular functions.
Added Information
The enzymes of the TCA cycle are located in the matrix of mitochondria, while enzymes associated with the respiratory chain and ATP production are present on the inner mitochondrial membrane. Enzymes for conversion of ADP toATP are located in the intermembranous space. Enzymes for lipid synthesis and fatty acid metabolism are located in the outer membrane.
Clinical Correlation
Mitochondrial Abnormalities
Mitochondrial DNA can be abnormal. This interferes with mitochondrial and cell functions, resulting in disorders referred to as mitochondrial cytopathy syndromes. The features (which differ in intensity from patient to patient) includes muscle weakness, degenerative lesions in the brain and high levels of lactic acid. The condition can be diagnosed by EM examination of muscle biopsies. The mitochondria show characteristic para-crystalline inclusions.
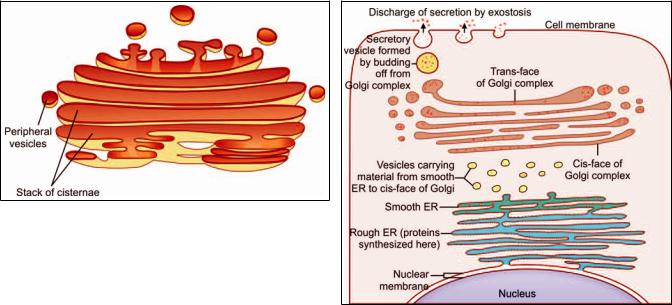
14 Textbook of Human Histology
Fig. 2.21: Structure of the Golgi complex (Schematic representation)
Golgi Complex
The Golgi complex (Golgi apparatus, or merely Golgi) was known to microscopists long before the advent of electron microscopy. In light microscopic preparations suitably treated with silver salts, the Golgi complex can be seen as a small structure of irregular shape, usually present near the nucleus (see Fig. 2.18).
When examined with the EM, the complex is seen to be made up of network of flattened smooth membranes similar to those of smooth ER (Fig. 2.21). The membranes form the walls of a number of flattened sacs that are stacked over one another. Toward their margins the sacs are continuous with small rounded vesicles. The cisternae of the Golgi complex form an independent system. Their lumen is not in communication with that of ER. Material from ER reaches the Golgi complex through vesicles.
From a functional point of view the Golgi complex is divisible into three regions (Fig. 2.22). The region nearest the nucleus is the cis face (or cis Golgi). The opposite face (nearest the cell membrane) is the trans face (also referred to as trans Golgi). The intermediate part (between the cis face and the trans face) is the medial Golgi.
Material synthesized in rough ER travels through the ER lumen into smooth ER. Vesicles budding off from smooth ER transport this material to the cis face of the Golgi complex. Some proteins are phosphorylated here. From the cis face all these materials pass into the medial Golgi. Here sugar residues are added to proteins to form proteincarbohydrate complexes (glycoproteins).
Finally, all material passes to the trans face, which performs the function of protein sorting, packaging and secretion. The membranes of the Golgi complex contain appropriate enzymes for the functions performed by them. As proteins pass through successive sacs of Golgi they undergo a process of purification.
Fig. 2.22: The role of the Golgi complex in formation of secretory vacuoles (Schematic representation)
Membrane Bound Vesicles
The cytoplasm of a cell may contain several types of vesicles. The contents of any such vesicle are separated from the rest of the cytoplasm by a membrane which forms the wall of the vesicle.
Vesicles are formed by budding off from existing areas of membrane. Some vesicles serve to store material. Others transport material into or out of the cell, or from one part of a cell to another. Vesicles also allow exchange of membrane between different parts of the cell.
Details of the appearances of various types of vesicles will not be considered here. However, the student must be familiar with their terminology given below.
Phagosomes
Solid “foreign” materials, including bacteria, may be engulfed by a cell by the process of phagocytosis. In this process, the material is surrounded by a part of the cell membrane. This part of the cell membrane then separates from the rest of the plasma membrane and forms a free floating vesicle within the cytoplasm. Such membrane bound vesicles, containing solid ingested material are called phagosomes (also see lysosomes).
Pinocytotic Vesicles
Some fluid may also be taken into the cytoplasm by a process similar to phagocytosis. In the case of fluids, the process is called pinocytosis and the vesicles formed are called pinocytotic vesicles.
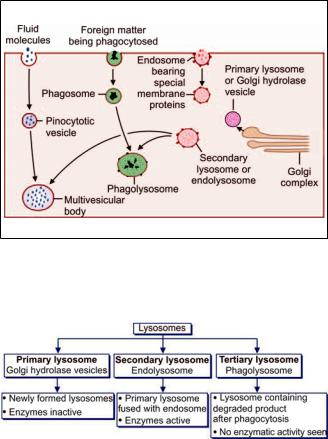
Chapter 2 Cell Structure 15
Exocytic Vesicles
Just as material from outside the cell can be brought into the cytoplasm by phagocytosis or pinocytosis, materials from different parts of the cell can be transported to the outside by vesicles. Such vesicles are called exocytic vesicles, and the process of discharge of cell products in this way is referred to as exocytosis (or reverse pinocytosis).
Secretory Granules
The cytoplasm of secretory cells frequently contains what are called secretory granules. These can be seen with the light microscope. With the EM, each “granule” is seen to be a membrane bound vesicle containing secretion. The appearance, size and staining reactions of these secretory granules differ depending on the type of secretion. These vesicles are derived from the Golgi complex.
Other Storage Vesicles
Fig. 2.23: Schematic representation to show how lysosomes, phagolysosomes, and multivesicular bodies are formed
Flowchart 2.1: Types of lysosomes
Materials such as lipids, or carbohydrates, may also be stored within the cytoplasm in the form of membrane bound vesicles.
Lysosomes
These membrane bound vesicles contain enzymes that can destroy unwanted material present within a cell. Such material may have been taken into the cell from outside (e.g. bacteria); or may represent organelles that are no longer of use to the cell. The enzymes present in lysosomes include (amongst others) proteases, lipases, carbohydrases and acid phosphatase as many as 40 different lysosomal enzymes have been identified.
Lysosomes belong to what has been described as the acid vesicle system. The vesicles of this system are covered by membrane which contains H+ATPase. This membrane acts as a H+ pump creating a highly acid environment within the vesicle (up to pH 5). The stages in the formation of a lysosome are as follows:
Acid hydrolase enzymes synthesized in ER reach the Golgi complex where they are packed into vesicles (Fig. 2.23). The enzymes in these vesicles are inactive because of the lack of an acid medium (these are called primary lysosomes or Golgi hydrolase vesicles) (Flowchart 2.1).
These vesicles fuse with other vesicles derived from cell membrane (endosomes). These endosomes possess the membrane proteins necessary for producing an acid medium. The product formed by fusion of the two vesicles is an endolysosome (or secondary lysosome) (Flowchart 2.1).
H+ ions are pumped into the vesicle to create an acid environment. This activates the enzymes and a mature lysosome is formed.
Lysosomes help in “digesting” the material within phagosomes as follows. A lysosome, containing appropriate enzymes, fuses with the phagosome so that the enzymes of the former can act on the material within the phagosome. These bodies consisting of fused phagosomes and lysosomes are referred to as phagolysosomes (Fig. 2.23).
In a similar manner lysosomes may also fuse with pinocytotic vesicles. The structures formed by such fusion often appear to have numerous small vesicles within them and are, therefore, called multivesicular bodies (Fig. 2.23).
After the material in phagosomes or pinocytotic vesicles has been “digested” by lysosomes, some waste material may be left. Some of it is thrown out of the cell by exocytosis. However, some material may remain within the cell in the form of membrane bound residual bodies.
Lysosomal enzymes play an important role in the destruction of bacteria phagocytosed by the cell. Lysosomal enzymes may also be discharged out of the cell and may influence adjoining structures.
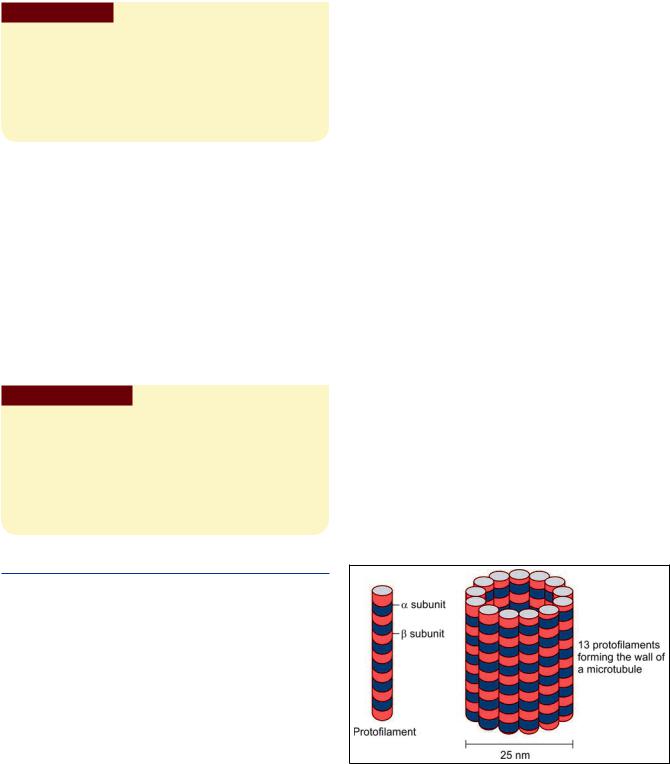
16Textbook of Human Histology
Note: Lysosomes are present in all cells except mature erythrocytes. They are a prominent feature in neutrophil leucocytes.
Clinical Correlation
Gen that are normally present in lysosomes. As a result some molecules cannot be degraded, and accumulate in lysosomes. Examples of such disorders are lysosomal glycogen storage disease, in which there is abnormal accumulation of glycogen, and Tay-Sach’s disease, in which lipids accumulate in lysosomes and lead to neuronal degeneration resulting in seizures, muscle rigidity and blindness.
Peroxisomes
These are similar to lysosomes in that they are membrane bound vesicles containing enzymes. The enzymes in most of them react with other substances to form hydrogen peroxide, which is used to detoxify various substances by oxidizing them. The enzymes are involved in oxidation of very long chain fatty acids. Hydrogen peroxide resulting from the reactions is bactericidal. Some peroxisomes contain the enzyme catalase which converts the toxic hydrogen peroxide to water, thus preventing the latter from accumulating in the cell.
Peroxisomes are most prominent in cells of the liver and in cells of renal tubules.
Pathologial Correlation
Adrenoleukodystrophy (Brown-Schilder’s disease) characterized by progressive degeneration of liver, kidney, and brain is a of very long chain fatty acids by peroxisomes.
Zellweger syndrome is characterized by formation of empty peroxisomes or peroxisomal ghosts inside the cells due to a defect in transportation of proteins into the peroxisomes.
Primary hyperoxaluria is due to the defective peroxisomal metabolism of glyoxyalate derived from glycine.
THE CYTOSKELETON
The cytoplasm is permeated by a number of fibrillar elements that collectively form a supporting network. This network is called the cytoskeleton. Apart from maintaining cellular architecture the cytoskeleton facilitates cell motility (e.g. by forming cilia), and helps to divide the cytosol into functionally discrete areas. It also facilitates transport of some constituents through the cytosol, and plays a role in anchoring cells to each other.
The elements that constitute the cytoskeleton consist of the following:
Microfilaments
Microtubules
Intermediate filaments.
Microfilaments
These are about 5 nm in diameter. They are made up of the protein actin. Individual molecules of actin are globular (G-actin). These join together (polymerize) to form long chains called F-actin, actin filaments, or microfilaments.
Actin filaments form a meshwork just subjacent to the cell membrane. This meshwork is called the cell cortex (the filaments forming the meshwork are held together by a protein called filamin). The cell cortex helps to maintain the shape of the cell. The meshwork of the cell cortex is labile. The filaments can separate (under the influence of actin severing proteins), and can reform in a different orientation. That is how the shape of a cell is altered.
Microvilli contain bundles of actin filaments, and that is how they are maintained. Filaments also extend into other protrusions from the cell surface.
Microtubules
Microtubules are about 25 nm in diameter (Fig. 2.24). The basic constituent of microtubules is the protein tubulin (composed of subunits and ). Chains of tubulin form protofilaments. The wallof a microtubule is made up of thirteen protofilaments that run longitudinally (Fig. 2.24). The tubulin protofilaments are stabilized by microtubule associated proteins (MAPs).
Microtubules are formed in centrioles (see below) which constitute a microtubule organizing center
The roles played by microtubules are as follows:
As part of the cytoskeleton, they provide stability to the cell. They prevent tubules of ER from collapsing.
Microtubules facilitate transport within the cell. Some proteins (dynein, kinesin) present in membranes of vesicles, and in organelles, attach these to microtubules, and facilitate movement along the tubules. Such transport is specially important in transport along axons.
Fig. 2.24: Schematic representation to show how a microtubule is constituted
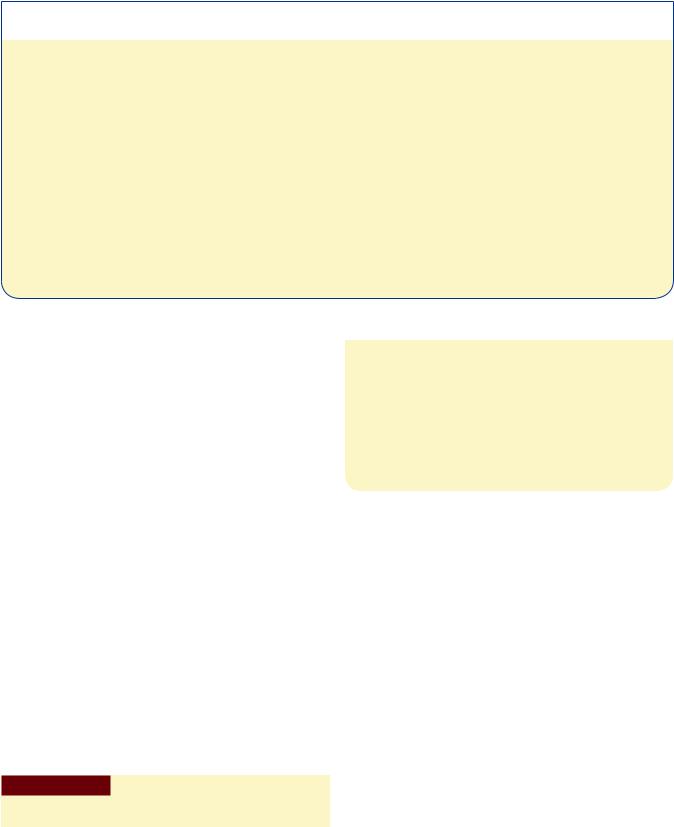
|
|
|
|
|
|
Chapter 2 Cell Structure 17 |
|
|
|
|
|
|
|
||
|
|
|
|
||||
|
|
|
Microtubules |
|
|||
Shape |
Double-stranded linear helical |
% & |
Long non-branching chains |
||||
|
arrangement |
|
|
|
|
|
|
Diameter |
5 nm |
10 nm |
25 nm |
|
|||
Basic protein |
Monomer of G-actin (protein) |
' ! ! |
Dimers of - and -tubulin |
||||
subunit |
|
|
& ! |
|
|
|
|
|
|
|
protein, desmin, laminin, and vimentin |
|
|
|
|
Location in cell |
• |
Forms a network just |
• |
Extend across cytoplasm connecting |
• |
Mitotic spindle |
|
|
|
subjacent to cell membrane |
|
desmosomes and hemidesmosomes |
• |
Core of cilia |
|
|
• |
Core of microvilli |
• |
The nuclear lamina |
|
|
|
|
• |
Contractile elements of |
• |
In skin epithelium (in the form of keratin) |
|
|
|
|
|
muscles |
|
|
|
|
|
|
|
|
|
|
|
||
Major |
Provide essential components |
Provide mechanical strength and link cells |
• |
Provide network for movement of |
|||
functions |
to contractile elements of |
together |
|
organelles within cell |
|||
|
muscle cells (sarcomeres) |
|
|
• |
Facilitate transport of organelles |
||
|
|
|
|
|
|
within the cell |
|
|
|
|
|
|
• |
Provide movement for cilia |
|
|
|
|
|
|
|
|
|
In dividing cells microtubules form the mitotic spindle.
Cilia are made up of microtubules (held together by other proteins).
Intermediate Filaments
These are so called as their diameter (10 nm) is intermediate between that of microfilaments (5 nm) and of microtubules (25 nm). The proteins constituting these filaments vary in different types of cells.
They include cytokeratin (in epithelial cells), neurofilament protein (in neurons), desmin (in muscle), glial fibrillary acidic protein (in astrocytes), Laminin (in the nuclear lamina of cells), and vimentin (in many types of cells).
The roles played by intermediate filaments are as follows:
Intermediate filaments link cells together. They do so as they are attached to transmembrane proteins at desmosomes. The filaments also facilitate cell attachment to extracellular elements at hemidesmosomes.
In the epithelium of the skin the filaments undergo modification to form keratin. They also form the main constituent of hair and nails.
The neurofilaments of neurons are intermediate filaments. Neurofibrils help to maintain the cylindrical shape of axons.
The nuclear lamina consists of intermediate filaments. Table 2.3 summarizes the characteristics of the three
types of cytoskeleton filaments.
Clinical Correlation
Kartagener syndrome: It is characterized by defect in the organization of microtubules that can immobilize the cilia of
Contd...
Contd...
respiratory epithelium resulting in the inability of the respiratory system to clear accumulated secretions.
Alzheimer's disease: Defect in the proper assembly of interThe disease is characterized by accumulation of tangles inside ments may cause disruption of cytoskeleton architecture and subsequent neuronal cell death leading to dementia (memory loss).
Centrioles
All cells capable of division (and even some which do not divide) contain a pair of structures called centrioles. With the light microscope, the two centrioles are seen as dots embedded in a region of dense cytoplasm which is called the centrosome. With the EM, the centrioles are seen to be short cylinders that lie at right angles to each other. When we examine a transverse section across a centriole (by EM) it is seen to consist essentially of a series of microtubules arranged in a circle. There are nine groups of tubules, each group consisting of three tubules (triplets) (Fig. 2.25).
Centrioles play an important role in the formation of various cellular structures that are made up of microtubules. These include the mitotic spindles of dividing cells, cilia, flagella, and some projections of specialized cells (e.g. the axial filaments of spermatozoa). It is of interest to note that cilia, flagella and the tails of spermatozoa, all have the 9 + 2 configuration of microtubules that are seen in a centriole.