
Control of Particle Size by Feed Composition in the Nanolatexes (2014)
.pdf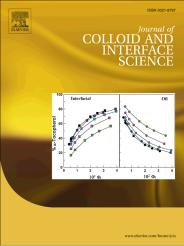
Accepted Manuscript
Control of Particle Size by Feed Composition in the Nanolatexes Produced via
Monomer-Starved Semicontinuous Emulsion Copolymerization
Shahriar Sajjadi |
|
|
PII: |
S0021-9797(14)00990-4 |
|
DOI: |
http://dx.doi.org/10.1016/j.jcis.2014.12.037 |
|
Reference: |
YJCIS 20080 |
|
To appear in: |
Journal of Colloid and Interface Science |
|
Received Date: |
12 |
November 2014 |
Accepted Date: |
12 |
December 2014 |
Please cite this article as: S. Sajjadi, Control of Particle Size by Feed Composition in the Nanolatexes Produced via Monomer-Starved Semicontinuous Emulsion Copolymerization, Journal of Colloid and Interface Science (2014), doi: http://dx.doi.org/10.1016/j.jcis.2014.12.037
This is a PDF file of an unedited manuscript that has been accepted for publication. As a service to our customers we are providing this early version of the manuscript. The manuscript will undergo copyediting, typesetting, and review of the resulting proof before it is published in its final form. Please note that during the production process errors may be discovered which could affect the content, and all legal disclaimers that apply to the journal pertain.
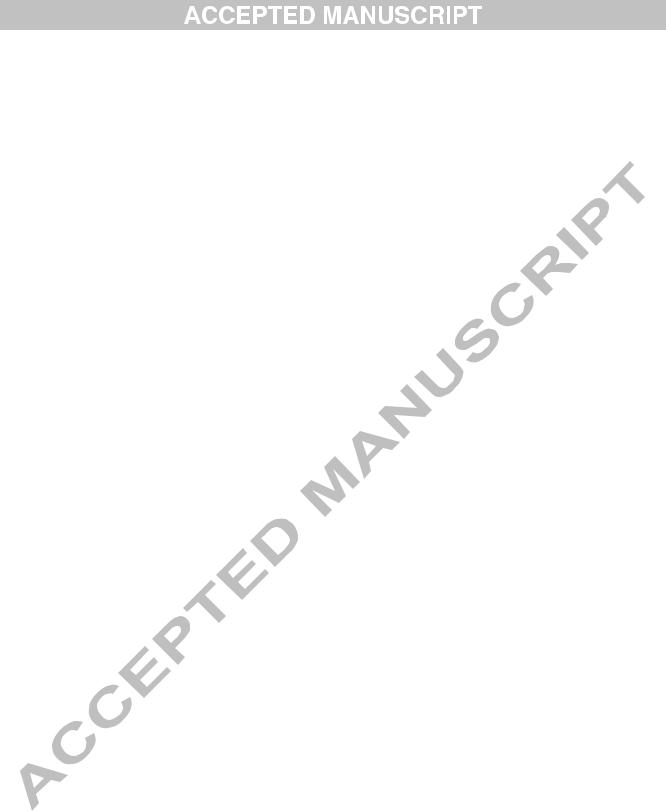
Control of Particle Size by Feed Composition in the Nanolatexes Produced via Monomer-
Starved Semicontinuous Emulsion Copolymerization
Shahriar Sajjadi, Department of Physics, King’s College London, Strand, London WC2R 2LS, UK.
Tel: 0044 02(0) 78482322; Fax: 0044 02(0) 78482932 ; Email: Shahriar.sajjadi-emami@kcl.ac.uk
ABSTRACT
Conventional batch and semicontinuous emulsion copolymerizations often produce large particles whose size cannot be easily correlated with the comonomer feed compositions, and are to some degree susceptible to composition drift. In contrast, we found that copolymer nanolatexes made via semicontinuous monomer-starved emulsion copolymerizations are featured with an average nanoparticle size being controlled by the feed composition, a high conversion achieved, and a high degree of particle composition uniformity. This was achieved because the rate of particle growth, during nucleation, was controlled by the rate of comonomer addition, and the copolymer composition, surfactant parking area on the particles, and nucleation efficiency by the comonomer feed composition. Two model systems, methyl methacrylate/styrene and vinyl acetate/butyl acrylate, with significant differences in water solubility were studied. Monomers were added to the aqueous solution of sodium dodecyl sulfate and potassium persulfate at a low rate to achieve high instantaneous conversions.
Keywords: Semicontinuous Emulsion copolymerization, copolymer nanoparticles, copolymer composition, nanolatxes, monomer-starved nucleation.
INTRODUCTION
Nanolatexes, which are dispersions comprised of particles smaller than 50 nm in diameter, have received extensive attentions in recent years [1-8]. With the advancement of nanotechnology, the demand for hybrid nanolatexes with different compositions is increasing [9-11]. Microemulsion polymerization is a well-established technique to produce nanolatexes, but uses a high concentration of surfactant/co-surfactant and produces low solids-content products [12-15]. Semicontinuous monomer-starved emulsion polymerization has been found as a powerful technique to produce high-solids content nanolatexes with an improved particle size uniformity [2,16] but using relatively low surfactant concentrations [1,2,4,6,17-23]. These nanolatexes have found applications for ultra-thin coatings and as intermediary in producing advanced functional particles and hybrid nanoclusters [7,24].
Several investigators attempted to explain the mechanistic of particle formation in such systems [25,26]. We have shown that for semicontinuous emulsion polymerization reactors of sparingly water-soluble monomers under starved conditions the number of particles (Np) can be given by [23]:
Np k1(as NA[S])Ri2 / 3Ra 2 / 3 |
(1) |
1
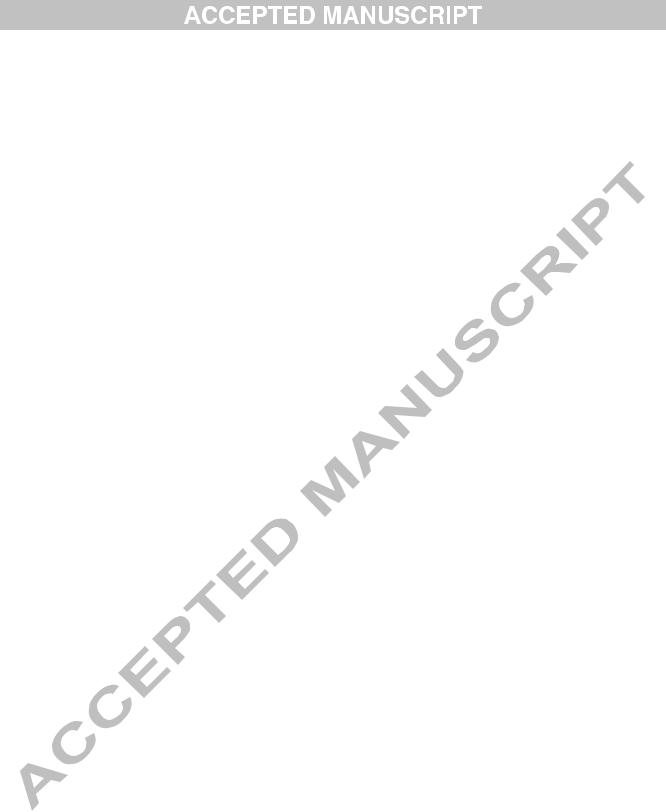
where k1 is a numerical constant, Ra is the rate of monomer addition, Ri the rate of radical entry into micelles and particles, as the adsorption area occupied by a molecule of emulsifier on the surface of polymer particles and [S] the concentration of emulsifier micelles per unit volume of water. The above equation indicates that it is theoretically possible to produce a large number of polymer particles with small size in a semicontinuous operation by decreasing Ra. The terms “micellar solution” and “microemulsion” have been interchangeably used in the polymer synthesis literature. A microemulsion is a thermodynamically stable dispersion of one liquid phase into another, similar to micelles, but stabilized by an interfacial film of surfactant/co-surfactant that usually has special features including a right geometry for packing at the interface [27], and ability to reduce the interfacial tension between bulk phases to ultra-low values to increase the effective volume for solubilisation [28,29]. In recent studies on monomer-starved semicontinuous emulsion polymerization, however, the term microemulsion has been widely used to convey micellar solution. Many of these works did not employ a cosurfactant and only used a conventional surfactant [8,13,15] such as sodium dedecyl sulfate, which is a standard surfactant for emulsion polymerization as in this current work. This suggests that Eq. 1 can broadly be applicable to 3-component microemulsion polymerizations reported in the literature.
Monomers with higher water solubility are usually considered to be capable of producing more particles because of their enhanced rate of radical entry and exit [30]. Nanolatexes made of partially water-soluble monomers are usually smaller in size than those made with sparingly water-soluble monomers [4,6]. We showed that monomers which produced similar particle size under conventional polymerization conditions, generated particle size in a distinct order of their water solubility in a system comprised of two-stratified layers of a monomer and water containing a surfactant and an initiator under diffusion-controlled conditions [31]. There are occasions that an opposite trend has also been observed [6,32].
Nucleation becomes more complicated when two monomers are involved in a copolymerization. However, if one of the monomers is highly soluble in water, such as acrylic and methacrylic acids, and vinylidine chloride [33], that monomer usually governs the nucleation. For other copolymerization systems, such as those with a pair of a sparingly water-soluble monomer and a relatively hydrophilic monomer, the variation in the number of particles (Np) with the feed composition is not straightforward. For example, Nomura et al. reported no variations in Np with the feed composition for styrene (St) and methyl methacrylate (MMA) emulsion copolymerization system [34].
Data reported by Forcada and Asua showed that for the same copolymerization system with sodium dodecyl sulfate (SDS) as surfactant, Np increased with the weight fraction of MMA in the feed (fMMA) if fMMA < 0.50 but remained constant for fMMA ≥ 0.50 [35]. Ouzineb et al. reported that for the copolymerization of MMA/BA (butyl acrylate) in the presence of SDS and Triton X-450 as surfactants the number of particles was not sensitive towards the comonomer feed and solely determined by the least soluble monomer; Np was almost equal to those produced by the corresponding batch homopolymerization of BA [36]. Kong et al. [37] reported that in batch vinyl acetate/butyl acrylate (VA/BA) copolymerization with aerosol as surfactant, the Np was found to increase with increasing VA content, but decreased when 100% VA was used in the feed. El-Aasser et al. [38] reported that the average particle size of batch VA/BA copolymerization using aerosol surfactant was independent of the copolymer feed. Ahmad et al. reported a reverse order for styrene/2-hyroxyethyl methacrylate copolymerization in which particle number
2
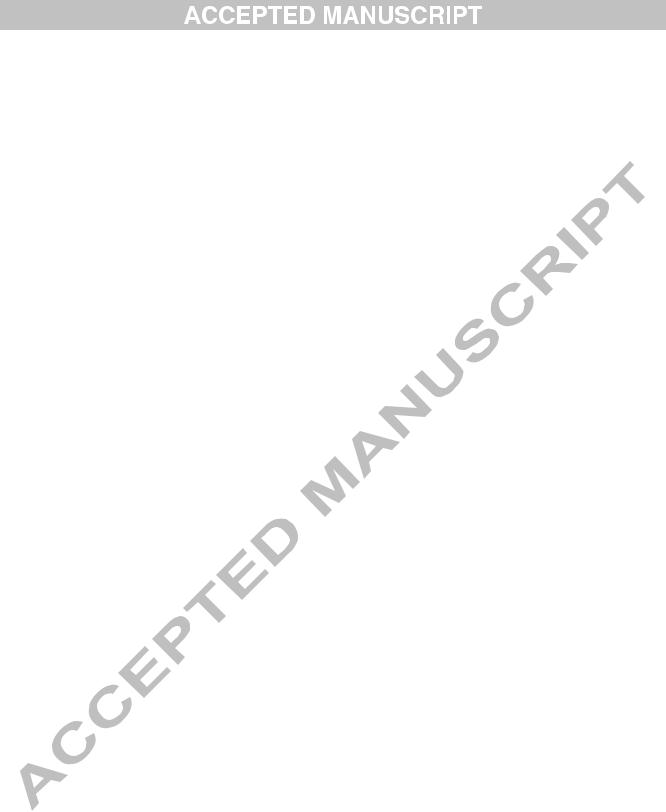
decreased with increasing concentration of the hydrophilic monomer (particle size increased with increasing concentration of the hydrophilic monomer) [39].
Similar behavior has also been observed for conventional semicontinuous emulsion copolymerizations. This is because conventional semicontinuous emulsion copolymerization are usually preceded with an in-situ seeding stage. This implies that nucleation in such systems occurs batchwise and under monomer-flooded conditions, despite the fact the subsequent polymerization may occur under monomer-starved conditions. For example, Chern et al. showed that for butyl acrylate (BA) – methyl methacrylate (MMA) semicontinuous emulsion copolymerization, Np could not be correlated to the MMA concentration in the water phase [40]. However, conventional semicontinuous emulsion copolymerization reactions are very efficient in maintaining uniform copolymer properties including molecular weight and copolymer composition [41,42].
Particle formation in copolymerizations is complicated because of the contribution of different properties associated with the comonomers. This includes variation in the rate of reaction with the comonomer feed composition. One important feature of semicontinuous monomer-starved emulsion polymerization is that the rate of reaction can be controlled by the rate of monomer addition. This may indicate that the variation in the rate of polymerization with the comonomer feed composition and its subsequent effect on nucleation becomes of secondary importance, suggesting a more straightforward relation between copolymer feed composition and number of particles. The objective of this study was to investigate the effect of copolymer feed composition on the particle size and number, and consequently on the kinetics of nanolatexes, in the semicontinuous emulsion copolymerization of model systems. Not only semicontinuous monomer-starved emulsion polymerization as means to produce copolymer nanolatexes has been scarcely reported in the literature [10,43,44], there has been little attention paid so far to the effects of comonomer feed composition on the number of particles. In this paper, MMA/St and VA/BA emulsion copolymerizations in a semicontinuous reactor with a neat comonomer feed were studied. MMA and VA, compared to ST and BA, have higher water solubility.
EXPERIMENTAL
Materials: The experiments were performed using the ingredients given in Table 1. St, MMA, VA, and BA were obtained from Aldrich and vacuum distilled before use. The initiator, potassium persulfate (KPS), and the emulsifier, sodium dodecyl sulfate (SDS) (> 99.0 % purity), obtained from Merck and Sigma Chemical Co., respectively, were used as received. Sodium bicarbonate (SBc; Sigma-Aldrich) was used as a buffer.
Procedures: Polymerizations were carried out in a 1-L jacketed glass reactor, equipped with a 4-blade baffle, a flat blade turbine and an adjustable cooling temperature system. The temperature of the reactor content was controlled within 0.50 C of the set reaction temperature. Stirring speed was kept constant at 350 rpm. The reactor vessel and the feed were kept under the blanket of nitrogen. The aqueous micellar solution of SDS containing the initiator was placed inside the vessel, and then the monomer(s) was fed to the reactor using a reciprocating pump at a constant rate. The feed rate showed 2.5 % variation from the set value during feeding. The average value was used for calculations. The feeding rates were kept constant at 1.0 and 0.7 g/min for MMA/St and VA/BA copolymerization systems, respectively.
3
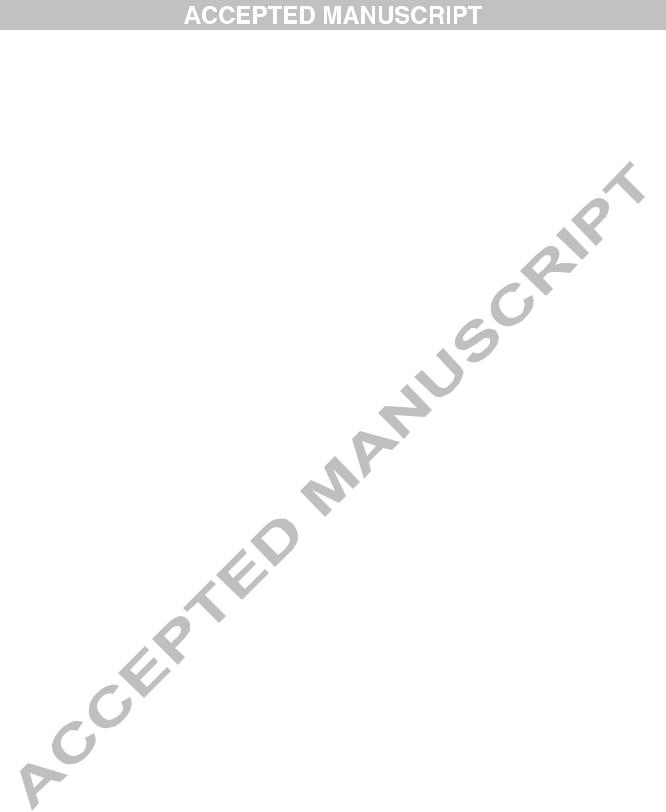
Table 1. Recipe used for MMA/St and VA/BA semicontinuous microemulsion copolymerization.
Formulation |
Initial |
Feed |
|
charge |
(g) |
|
(g) |
|
MMA+St a |
0 a |
150 |
DDI |
600 |
- |
SDS |
3.00 |
- |
KPS |
0.75 |
- |
SBc |
- |
- |
VA+BA b |
0 b |
250b |
DDI |
500 |
- |
SDS |
5.00 |
- |
KPS |
0.27 |
- |
SBc |
0.27 |
- |
|
|
|
a Most reactions were carried out with 0 g monomer, (R/F)M = 0, in the initial charge. However, the reaction with the initial charge ratio of (R/F)M = 0.20 started with 30 g (20 wt% of the total monomers) of monomers and the remainder of the monomers (120 g) was added to the reactor semibatchwise after 15 min (pre-period time) from the start of reaction (i.e., addition of initiator). Two reaction temperatures of 50 and 70 C were used for (R/F)M = 0. The reaction with (R/F)M = 0.20 was carried out at 50 C only. The feeding rates was kept constant at 1.0 g/min.
b Most reactions were carried out with 0 g monomer, (R/F)M = 0, in the initial charge. The reaction with the initial charge of (R/F)M = 0.10 was carried out with 25 g of the monomers. The remainder of monomers (225 g) was added into the reactor at a given feed rate after 10 min (pre-period time) from the start of reaction. All reactions were carried out at the temperature of 50 C. The feeding rates was kept constant at 0.7 g/min.
Measurements: The monomer conversions in the reactor were measured gravimetrically. The instantaneous conversion at a given time, xi, is defined as the weight ratio of the polymer formed in the reactor to the total amount of monomer fed into the reactor by that time plus the initial charge, if any. Overall conversion, x0, is defined as the weight ratio of polymer in the reactor to the total monomer in the recipe. The (steady-state) rate of polymerizations was directly calculated from the slope of the overall conversion-time curves.
Particle size measurements were carried out using dynamic light scattering (DLS; Malvern) at an angle of 90 degree and at room temperature. The z-average (Dz) diameter of unswollen particles was directly obtained from the DLS measurements. The number of particles (Np ) was calculated as follows:
N p ( |
6xi |
Ra t |
|
(2) |
||
p Dv |
3 ) |
|||||
|
where Dv is the volume average of particles, Rat is the weight of monomers added to the reactor by the time t and p is the average density of the copolymer obtained by p F1 1 F2 2 where F1 and F2 are cumulative copolymer composition, and 1 and 2 are density of polymers 1 and 2, respectively. The values of 1.044, 1.04, 1.11, 1.178 g/cm3 were considered as polymer density for PS, PBA, PVA, and PMMA, respectively. To avoid underestimation of Np, the Dz value, obtained by DLS, was converted to the volume-average diameter (Dv) required in Eq.2, by applying a conversion factor obtained by analyzing the particles sizes of selected samples with a transmission electron microscope (TEM, Nippon). Three of the final latex particles were examined by TEM and the
4
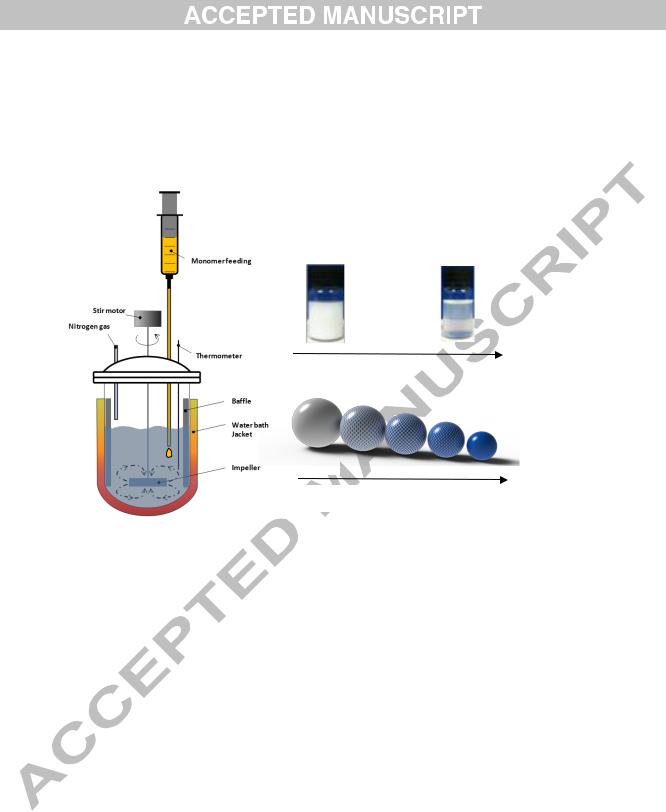
corresponding Dz and Dv values were calculated according to the procedure explained elsewhere [2]. The ratio of
Dv/Dz = 0.92 0.02 was used to convert the Dz obtained by DLS to the Dv required. Surface tensions were measured using Du-Nouy ring method.
(b)
(a)
(c)
Increasing water solubility of co-monomer
Fig. 1. a) A schematic for the experimental set up, and changes in b) the physical appearance of nanolatexes and c) size and composition of particles with increasing water-solubility of the comonomer used in polymerization.
Copolymer composition was measured according to the following procedure. The concentration of unreacted monomers in the samples was measured using gas liquid chromatography (Perkins) to calculate copolymer composition. The polymers were precipitated with a mixture of methanol and acetone (including some amount of aluminum sulfate in the case of VA polymerization to facilitate polymer precipitation) containing a small amount of hydroquinone to prevent further polymerization and then filtered off with a glass crucible (no. 4). The individual monomer conversion was determined by measuring the content of each monomer in the filtrate by gas liquid chromatography, and used to check the overall conversion measured gravimetrically. Dioxane (Aldrich) was used as internal standard. By a simple mass balance, the cumulative copolymer composition was obtained. The precipitated polymers were dried in a vacuum oven to obtain the overall monomer conversion, as an alternative to the gravimetrical method already mentioned.
3. RESULTS
Experiments were conducted according to the formulations presented in Table 1. The experimental set up and concept behind these experiments are shown in Fig. 1. Before a monomer molecule can participate in a polymerization reaction it must cross the droplet-water interface and diffuse through the aqueous phase into the
5
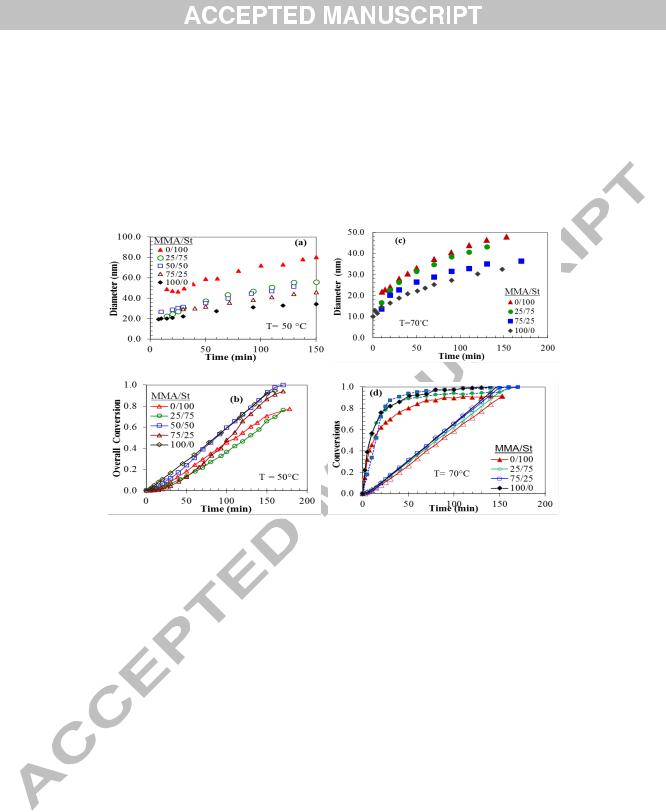
polymer-monomer particles [45]. The added monomer was first dissolved in the water phase and solubilised in the micellesIn most semicontinuous runs with no initial monomer charge, the transparent aqueous micellar solution gradually turned into a bluish one, with the addition of the monomers and progress of reaction, indicating formation of nanoparticles. The solution then could turn into an opaque one with further addition of monomers, depending on the polymerization conditions.
Fig. 2. Time variations in a) volume average diameter of particles and b) overall conversion at 50○C, and in c) volume average diameter of particles and d) conversions at 70○C for different MMA/St feed compositions. Closed and open symbols represent instantaneous and overall conversion, respectively.
3.1 Kinetic Data and Particle Size
MMA/St system: This represents copolymerization systems with a mild reactivity difference or composition drift. Styrene (St) and methyl methacrylate (MMA) have reactivity ratios of rS = 0.52 and rMMA = 0.46, respectively [34]. The least water-soluble monomer, St, is therefore the most reactive monomer.
The effect of feed composition on the size of particles is shown in Fig. 2a. Particles as small as 20 nm in diameter were produced in the early reactions, which reached around 30-70 nm by the end of reaction, depending on the feed composition.
Fig. 2b depicts conversion-time histories. The rate of polymerizations varied in the early stage with fMMA, but approached the rate of monomer addition rather soon. The results from Fig. 2b indicate that the rate of polymerization (Rp) is not tightly controlled by the rate of monomer addition (Ra), as can be inferred from the slope of xo-t curves in the early stage of polymerizations. This can also be found out by the pattern in which Dv varied with time for St polymerization; particles initially decreased in size before rising again. There are several possible ways to approach the equality of type Rp = Ra. One method is to alter process variables such as decreasing monomer feed rate, as a means to control Rp. Another method employs alteration in formulation variables, such as an increase in the initiator concentration as well as in reaction temperature.
6
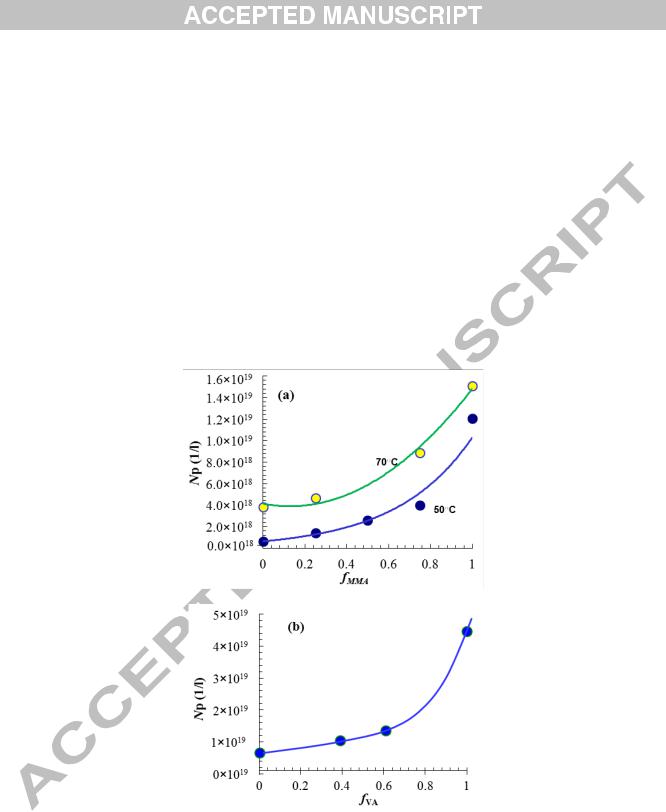
In order to increase the rate of reaction, we conducted few experiments under the same conditions but at the reaction temperature of 70○C. The results for particles average size and conversions for these experiments are given in Fig. 2c and 2d, respectively. The end of feeding can be inferred from the conversion-time graphs at the points where both overall and instantaneous conversions converge (Fig. 2d). For the runs at the higher temperature, the rate of reaction was tightly controlled by the rate of addition from the beginning to the end of reaction so that Rp ≈
Ra, as seen in Fig. 2d. The variations in surface tension of latexes with time indicated that particle nucleation occurred in the presence of micelles (examples of the time evolution of surface tension and Np have been shown in Fig. 5d and 5g for MMA/St and VA/BA systems, respectively, and are discussed later). The average size of particles started from 10 nm in diameter and continuously increased with time, indicating that they were formed under starved conditions. The average diameter of particles reached 32 nm for PMMA and 50 nm for PSt. PMMA nanolatex appeared to be bluish, whereas PSt latex was rather milky, with other latexes ranging in between (see Fig. 1c).
Fig. 3. Variations in Np with (a) fMMA and (b) fVA. For MMA/St system, the results for two temperatures of 50 and 70○C are shown. For VA/BA system, the reaction temperature is 50○C.
Fig. 3a shows variations in Np with fMMA for different reaction temperatures (Fig. 3b is discussed later). We find from this figure that the number of particles is more dependent on the feed composition under starved conditions, than under flooded conditions as reported in the literature. Previous reports on MMA/St batch copolymerization point to almost a constant Np with fMMA.
VA/BA system: Vinyl acetate (VA) and n-butyl acrylate (BA) represent copolymerization systems with very disparate reactivity ratios: rVA= 0.08; rBA= 7.2 [31]. A polymer chain ending in vinyl acetate is more willing to add
7

BA, while a polymer chain ending with BA will also add BA monomer. Thus, the batch copolymerization of VA and BA yields a BA-rich core and a VA-rich shell latex. This is why semicontinuous emulsion polymerization process is generally used for industrial production of this latex. VA and BA also have a wide difference in solubility in water (2.50 and 0.14 g/100g, respectively), which makes them good models for this study.
The effects of feed composition on conversion and size of particles for VA/BA system are shown in Fig. 4. The particles changed little in size in the early reaction during nucleation, but started growing later in the course of reaction. The smallest particles detected was around 16 nm in diameter for VA and 25 nm for BA monomer. The final size of particles for PVA and PBA nanolatexes was 24 and 48 nm, respectively, with the size of latexes from copolymerization runs falling within this range. No significant reduction in Np occurred in the course of particle nucleation and growth (examples of Np-t curves are shown in Fig. 5), indicating that particle surface coverage did not fall below the critical value in the course of feeding to allow for particle coagulation to occur [46]. The rate of polymerization was the same for all runs except for the run fVA = 0.69, which was slightly smaller. Fig.3b shows variations in Np with fVA. Despite a small difference between Rp for VA homopolymerization (0/100) and those of other runs, the number of particles showed a consistent increase with fVA. The reports on VA/BA batch copolymerizations reveal insignificant variations in Np with fVA [31,32].
(a)
(b)
Fig. 4. Variations in (a) conversions and (b) particle size for VA/BA system (Ra = 0.7 g/min). Closed and open symbols in figure (a) represent overall and instantaneous conversion, respectively.
3.2 Copolymer Composition and Particles Uniformity
In this section, we study the composition drift of the copolymers formed. Conventional semicontinuous emulsion polymerization is usually started with a seeding batch stage, which includes some monomer in the initial charge,
8
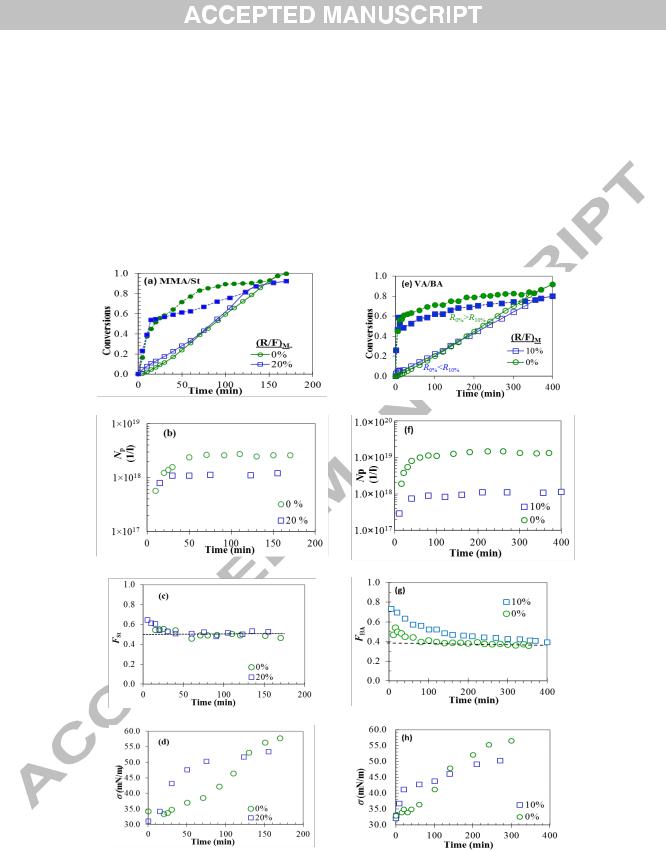
during which seed particles are formed. The weight ratio of the initial monomer charge in the reactor to the total
monomer in the feed, (R/F)M, can vary from zero or a small value (micellar solution) to a big proportion of the whole monomer in the recipe (emulsion). In order to demonstrate the difference in composition drift of nanolatexes
resulting from monomer-starved semicontinuous emulsion polymerization, indicated by (R/F)M=0, and latexes made by conventional semicontinuous emulsion polymerizations, indicated by (R/F)M>0, we conducted a couple of experiments with an initial amount of monomers in the reactor charge for the seeding stage and allowed a pre-
period time during which seed particles were formed. The conditions for these experiments are explained in the
caption of Table 1.
Fig. 5. Variations in (a) and (e) conversions, (b) and (f) number of particles, (c) and (g) copolymer composition, and (d) and (h) surface tension with time for S/MMA and VA/BA systems, respectively. FSt and FBA represent polymer composition for MMA/St and VA/BA systems, respectively. (abcd; MMA/St= 50/50 and efgh ; VA/BA=61/39). Closed and open symbols in figures (a) and (e) represent instantaneous and overall conversion, respectively. The reactions temperature is 50○C.
Fig. 5 shows variations in conversions (xi and xo), Np, F2, and (surface tension) with time for the two runs in comparison with the runs carried out with (R/F)M = 0, which means that no monomer was placed in the initial
9