
- •1. Introduction
- •2. Experimental system and diagnostics
- •3. Experimental results
- •4. The model
- •4.1. The formulation and implementation of the model
- •4.2. The set of gas phase reactions
- •4.3. The set of surface reactions
- •5. Model results and discussion
- •5.1. Model results and comparison with the experimental data
- •5.2. The contribution of the reactions
- •6. Conclusions
- •Acknowledgments
- •References
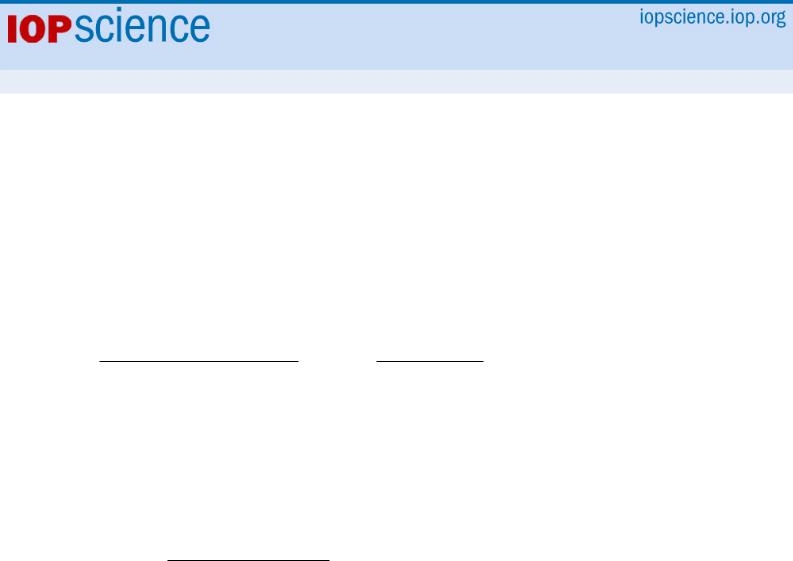
Home Search Collections Journals About Contact us My IOPscience
A global model for SF6 plasmas coupling reaction kinetics in the gas phase and on the surface of the reactor walls
This article has been downloaded from IOPscience. Please scroll down to see the full text article.
2009 J. Phys. D: Appl. Phys. 42 055209
(http://iopscience.iop.org/0022-3727/42/5/055209)
View the table of contents for this issue, or go to the journal homepage for more
Download details:
IP Address: 194.67.106.32
The article was downloaded on 23/11/2011 at 08:19
Please note that terms and conditions apply.

IOP PUBLISHING |
JOURNAL OF PHYSICS D: APPLIED PHYSICS |
J. Phys. D: Appl. Phys. 42 (2009) 055209 (15pp) |
doi:10.1088/0022-3727/42/5/055209 |
A global model for SF6 plasmas coupling reaction kinetics in the gas phase and on the surface of the reactor walls
George Kokkoris1, Apostolos Panagiotopoulos1, Andy Goodyear2,
Mike Cooke2 and Evangelos Gogolides1
1Institute of Microelectronics, NCSR Demokritos, Aghia Paraskevi, Attiki 15310, Greece
2Oxford Instruments Plasma Technology, Yatton, Bristol, UK
E-mail: gkok@imel.demokritos.gr and evgog@imel.demokritos.gr
Received 31 July 2008, in final form 15 December 2008
Published 19 February 2009
Online at stacks.iop.org/JPhysD/42/055209
Abstract
Gas phase and reactor wall-surface kinetics are coupled in a global model for SF6 plasmas. A complete set of gas phase and surface reactions is formulated. The rate coefficients of the electron impact reactions are based on pertinent cross section data from the literature, which are integrated over a Druyvesteyn electron energy distribution function. The rate coefficients of the surface reactions are adjustable parameters and are calculated by fitting the model to
experimental data from an inductively coupled plasma reactor, i.e. F atom density and pressure change after the ignition of the discharge. The model predicts that SF6, F, F2 and SF4 are the dominant neutral species while SF+5 and F− are the dominant ions. The fit sheds light on the interaction between the gas phase and the reactor walls. A loss mechanism for SFx radicals by deposition of a fluoro-sulfur film on the reactor walls is needed to predict the experimental data. It is found that there is a net production of SF5, F2 and SF6, and a net consumption of F, SF3 and SF4 on the reactor walls. Surface reactions as well as reactions between neutral species in the gas phase are found to be important sources and sinks of the neutral species.
(Some figures in this article are in colour only in the electronic version)
1. Introduction
The plasma of sulfur hexafluoride (SF6) has been widely used for Si etching in a variety of applications. During the BOSCH [1] or gas chopping [2] or time multiplexed deep etching process [3] in micro-electro-mechanical systems (MEMS) fabrication, SF6 is used with C4F8 (or other fluorocarbon gases) to produce deep Si structures. It is also used with O2 in cryogenic [4, 5] or noncryogenic processes [6]. Besides deep Si etching, SF6 is used in cases where isotropy is desired, for example during the release of suspended structures [7], e.g. bridges and cantilevers. It is also used for the morphological modification of polymeric surfaces [8]. SF6 plasma is also used for the production of the beam in neutral beam etching systems [9–11].
There have been experimental studies for SF6 plasmas which focus on the electron impact reactions [12–16]. An open issue is the paths and the pertinent cross sections for the
neutral dissociation of SF6 [12] and SFx (x < 6) radicals. Iio et al [16] measured the cross sections for electron impact dissociation of SF6 into SF, SF2 and SF3. Christophorou and Olthoff [12] deduced cross sections for the total dissociation into neutral fragments which were seen to have a different energy dependence from the sum of the cross sections for dissociation reported by Iio et al [16].
There have also been experimental studies [17–23] which focus on the characterization of the gas phase of SF6, SF6/Ar and SF6/O2 plasmas, e.g. the measurement of the densities of charged species, in several systems and conditions.
Concerning the computational studies for low pressure SF6 or SF6/O2 plasmas, a number of works [17, 24–29] have been published. Kline [24] treated both electron and chemical kinetics of SF6 plasmas. He considered a plug flow reactor model and assumed multi-step dissociation of SF6 (SF6 → SF5 → SF4 → . . .). Surface processes were included only for Si surfaces; for the rest of the surfaces,
0022-3727/09/055209+15$30.00 |
1 |
© 2009 IOP Publishing Ltd Printed in the UK |
J. Phys. D: Appl. Phys. 42 (2009) 055209 |
G Kokkoris et al |
reflection coefficients for neutral species were considered. Anderson et al [26] developed a zero-dimensional (0D) model to describe SF6/O2 discharges. They considered several singlestep dissociations of SF6 to SF6−x radicals and estimated the relative rate coefficients. Concerning the surface processes, they considered that SF5, SF3 and F are lost at the surfaces with a probability. Ryan and Plumb [25] developed a 0D model for SF6/O2 plasmas. Emphasis was placed on the gas phase reactions between neutral species, and no surface reactions on the walls were considered. Meyyappan [28] used a 0D model to study pulsed power SF6 plasma. Concerning the wall-surface reactions, it was mentioned that the reactor walls were significantly coated with products containing sulfur and a constant sticking coefficient was considered for all neutral species due to lack of pertinent data. Riccardi et al [17] developed a plug flow model to describe SF6 capacitively coupled plasma (CCP). They considered both multi-step and single-step dissociation reactions of SF6. They considered a simple surface model where F atoms stuck on the surface with a probability. Rauf et al [27] reported a set of gas phase reactions for SF6; they used a 2D simulation tool to study the BOSCH process. They considered single-step dissociation paths of SF6 to SF3 and SF2, following Iio et al [16] and assumed a branching 2/3 to SF3 and 1/3 to SF2. Regarding the surface reactions, sticking probabilities on the walls and on the wafer were assumed for F atoms and SFx radicals, and surface production of F2 was also considered. Finally, computational work has also been done in the fields of glow corona discharges [30] and arc plasmas [31].
Despite the facts that the importance of surface reactions has been pointed out [24, 28] and the interaction with the reactor walls affects the reproducibility of the plasma etching processes [32] and the nanoscale roughness developed on the etched surfaces ( [33, 34] for Si etching), there is a lack of detailed description of the reactions on the wall surface. In this work, a global type [35] model for SF6 plasmas is developed and couples gas phase and wall-surface reaction kinetics. The coupling quantifies the effect of wall-surface reactions on the species densities in the gas phase. The detailed set of surface reactions of the model comes to fill the pertinent lack in the literature and to investigate the surface reactions of SFx radicals which are not well understood [28]. Recombination, sticking and deposition processes are taken into account. The model consists of mass balances for neutral and charged species in the gas phase, as well as for adsorbed species on the wall surfaces, and a power balance. The computational framework we used is the same as in a recent work for C4F8 plasmas [36].
The coefficients for the gas phase reactions are calculated either by integrating cross sections from the literature over a Druyvesteyn electron energy distribution function (EEDF) (electron impact reactions), or come directly from literature sources (non-electron impact reactions). The coefficients for the surface reactions are adjustable parameters of the model and are calculated by fitting the model to experimental measurements conducted in an inductively coupled plasma (ICP) reactor, i.e. F atom density and pressure change after the ignition of the discharge. The fit is done with a modified Levenberg–Marquardt algorithm [37].
The model allows the calculation of derived outputs such as the pressure rise, i.e. the change in the pressure after plasma ignition when the pressure controller of the reactor is off, and the effective sticking or surface loss coefficients of the neutral species. In addition, a study of the relative contribution of each reaction to the production and consumption of the species is performed in order to find the major sources and sinks of the species.
The paper is structured as follows: in section 2, information for the experimental system and diagnostics is included. In the next section, the experimental results are presented. The formulation and the implementation of the model are described in section 4.1. Sections 4.2 and 4.3 include the sets of gas phase and surface reactions. The results of the model are discussed in section 5.1. The major sources and sinks and the effective sticking coefficients of the species are calculated in sections 5.2 and 5.3. The summary of a sensitivity analysis is included in section 5.4 and the conclusions are summarized in section 6.
2. Experimental system and diagnostics
The experiments were conducted in an ICP reactor of Oxford Instruments Plasma Technology (Plasmalab System 100 with ICP 380 source [38]) used for multipurpose etching experiments. The reactor consists of an alumina cylindrical source tube (ICP tube) and a second cylindrical aluminium chamber (main or diffusion chamber). The ICP tube has a radius of 19 cm and its height is 21 cm. The radius of the main chamber is 19 cm and its height is 17 cm. The radius of the electrode table is 10.5 cm. The system is described in detail elsewhere [38].
The feed of the reactor was 100 sccm SF6 and 10 sccm Ar, which was used as an actinometer.
The purity of the SF6 gas was greater than 99.97% (electronic grade). The impurities of the parent gas, such as water vapour, join reactions in the gas phase as well as reactions on the wall surfaces producing several by-products [39] and may affect the regime of high pressure discharges [40] when they are present in high concentrations. However, due to the very small concentration of these impurities (e.g. 8 ppm for water vapour) and the low pressure of our experiments, we considered that they could not affect the pressure or the densities of the species in the gas phase.
During the measurements, the throttle valve between the chamber and the pumping system was at a fixed position, i.e. the pressure controller was off; thus, the pressure during the discharge changed compared with that before the ignition of the plasma (pressure with plasma off, pOFF) due to the dissociation of the parent gas. No power was applied at and no wafer was placed on the aluminium electrode. The walls of the diffusion chamber of the reactor were heated to approximately 30–40 ◦C by embedded heaters, but the ICP tube could have been hotter, as there was no temperature control. A conditioning SF6 plasma was initially run. Then, two series of measurements were carried out at constant pOFF and at constant source power. In particular, the pressure rise, i.e. the difference in the pressure after plasma ignition compared with pOFF, and the actinometric
2