
- •Radio Engineering for Wireless Communication and Sensor Applications
- •Contents
- •Preface
- •Acknowledgments
- •1 Introduction to Radio Waves and Radio Engineering
- •1.1 Radio Waves as a Part of the Electromagnetic Spectrum
- •1.2 What Is Radio Engineering?
- •1.3 Allocation of Radio Frequencies
- •1.4 History of Radio Engineering from Maxwell to the Present
- •2.2 Fields in Media
- •2.3 Boundary Conditions
- •2.4 Helmholtz Equation and Its Plane Wave Solution
- •2.5 Polarization of a Plane Wave
- •2.6 Reflection and Transmission at a Dielectric Interface
- •2.7 Energy and Power
- •3 Transmission Lines and Waveguides
- •3.1 Basic Equations for Transmission Lines and Waveguides
- •3.2 Transverse Electromagnetic Wave Modes
- •3.3 Transverse Electric and Transverse Magnetic Wave Modes
- •3.4 Rectangular Waveguide
- •3.4.1 TE Wave Modes in Rectangular Waveguide
- •3.4.2 TM Wave Modes in Rectangular Waveguide
- •3.5 Circular Waveguide
- •3.6 Optical Fiber
- •3.7 Coaxial Line
- •3.8 Microstrip Line
- •3.9 Wave and Signal Velocities
- •3.10 Transmission Line Model
- •4 Impedance Matching
- •4.1 Reflection from a Mismatched Load
- •4.2 Smith Chart
- •4.3 Matching Methods
- •4.3.1 Matching with Lumped Reactive Elements
- •4.3.4 Resistive Matching
- •5 Microwave Circuit Theory
- •5.1 Impedance and Admittance Matrices
- •5.2 Scattering Matrices
- •5.3 Signal Flow Graph, Transfer Function, and Gain
- •6.1 Power Dividers and Directional Couplers
- •6.1.1 Power Dividers
- •6.1.2 Coupling and Directivity of a Directional Coupler
- •6.1.3 Scattering Matrix of a Directional Coupler
- •6.1.4 Waveguide Directional Couplers
- •6.1.5 Microstrip Directional Couplers
- •6.2 Ferrite Devices
- •6.2.1 Properties of Ferrite Materials
- •6.2.2 Faraday Rotation
- •6.2.3 Isolators
- •6.2.4 Circulators
- •6.3 Other Passive Components and Devices
- •6.3.1 Terminations
- •6.3.2 Attenuators
- •6.3.3 Phase Shifters
- •6.3.4 Connectors and Adapters
- •7 Resonators and Filters
- •7.1 Resonators
- •7.1.1 Resonance Phenomenon
- •7.1.2 Quality Factor
- •7.1.3 Coupled Resonator
- •7.1.4 Transmission Line Section as a Resonator
- •7.1.5 Cavity Resonators
- •7.1.6 Dielectric Resonators
- •7.2 Filters
- •7.2.1 Insertion Loss Method
- •7.2.2 Design of Microwave Filters
- •7.2.3 Practical Microwave Filters
- •8 Circuits Based on Semiconductor Devices
- •8.1 From Electron Tubes to Semiconductor Devices
- •8.2 Important Semiconductor Devices
- •8.2.1 Diodes
- •8.2.2 Transistors
- •8.3 Oscillators
- •8.4 Amplifiers
- •8.4.2 Effect of Nonlinearities and Design of Power Amplifiers
- •8.4.3 Reflection Amplifiers
- •8.5.1 Mixers
- •8.5.2 Frequency Multipliers
- •8.6 Detectors
- •8.7 Monolithic Microwave Circuits
- •9 Antennas
- •9.1 Fundamental Concepts of Antennas
- •9.2 Calculation of Radiation from Antennas
- •9.3 Radiating Current Element
- •9.4 Dipole and Monopole Antennas
- •9.5 Other Wire Antennas
- •9.6 Radiation from Apertures
- •9.7 Horn Antennas
- •9.8 Reflector Antennas
- •9.9 Other Antennas
- •9.10 Antenna Arrays
- •9.11 Matching of Antennas
- •9.12 Link Between Two Antennas
- •10 Propagation of Radio Waves
- •10.1 Environment and Propagation Mechanisms
- •10.2 Tropospheric Attenuation
- •10.4 LOS Path
- •10.5 Reflection from Ground
- •10.6 Multipath Propagation in Cellular Mobile Radio Systems
- •10.7 Propagation Aided by Scattering: Scatter Link
- •10.8 Propagation via Ionosphere
- •11 Radio System
- •11.1 Transmitters and Receivers
- •11.2 Noise
- •11.2.1 Receiver Noise
- •11.2.2 Antenna Noise Temperature
- •11.3 Modulation and Demodulation of Signals
- •11.3.1 Analog Modulation
- •11.3.2 Digital Modulation
- •11.4 Radio Link Budget
- •12 Applications
- •12.1 Broadcasting
- •12.1.1 Broadcasting in Finland
- •12.1.2 Broadcasting Satellites
- •12.2 Radio Link Systems
- •12.2.1 Terrestrial Radio Links
- •12.2.2 Satellite Radio Links
- •12.3 Wireless Local Area Networks
- •12.4 Mobile Communication
- •12.5 Radionavigation
- •12.5.1 Hyperbolic Radionavigation Systems
- •12.5.2 Satellite Navigation Systems
- •12.5.3 Navigation Systems in Aviation
- •12.6 Radar
- •12.6.1 Pulse Radar
- •12.6.2 Doppler Radar
- •12.6.4 Surveillance and Tracking Radars
- •12.7 Remote Sensing
- •12.7.1 Radiometry
- •12.7.2 Total Power Radiometer and Dicke Radiometer
- •12.8 Radio Astronomy
- •12.8.1 Radio Telescopes and Receivers
- •12.8.2 Antenna Temperature of Radio Sources
- •12.8.3 Radio Sources in the Sky
- •12.9 Sensors for Industrial Applications
- •12.9.1 Transmission Sensors
- •12.9.2 Resonators
- •12.9.3 Reflection Sensors
- •12.9.4 Radar Sensors
- •12.9.5 Radiometer Sensors
- •12.9.6 Imaging Sensors
- •12.10 Power Applications
- •12.11 Medical Applications
- •12.11.1 Thermography
- •12.11.2 Diathermy
- •12.11.3 Hyperthermia
- •12.12 Electronic Warfare
- •List of Acronyms
- •About the Authors
- •Index
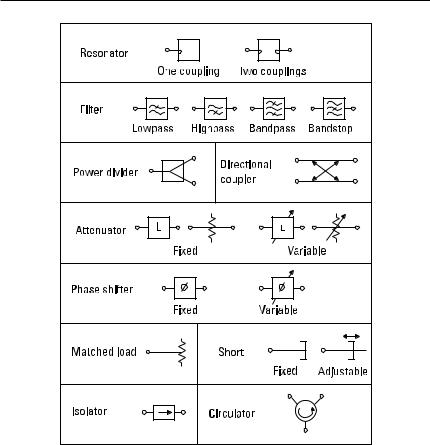
116 Radio Engineering for Wireless Communication and Sensor Applications
Figure 6.1 Standardized symbols for passive devices.
3.Quasioptical components handle waves propagating in a beam in free space. The dimensions of quasioptical components are larger than a wavelength. They are used in millimeter-wave and submilli- meter-wave systems.
6.1Power Dividers and Directional Couplers
Power dividers and directional couplers are components that split the input signal into two or more output ports. They may also be used as power combiners. Power dividers usually are three-port devices. Directional couplers have four ports and can separate waves propagating into opposite directions on the line. Directional couplers are used in impedance measurement and
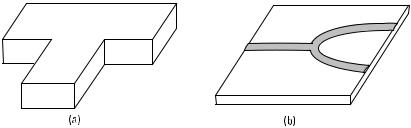
Passive Transmission Line and Waveguide Devices |
117 |
for taking a sample of a signal. Hybrids are 3-dB directional couplers with either a 90° or 180° phase difference between their output signals. Hybrids are needed in many mixers, modulators, and demodulators.
6.1.1 Power Dividers
The T-junctions shown in Figure 6.2 are simple power dividers. However, all the ports of a lossless three-port circuit cannot be matched. We can prove this by considering the properties of the scattering matrices. A passive, reciprocal, and matched three-port would have a scattering matrix, as
|
0 |
S12 |
S13 |
4 |
|
[S ] = |
S12 |
0 |
S23 |
(6.1) |
|
|
3S13 |
S23 |
0 |
|
As we stated in Chapter 5, the scattering matrix of a lossless circuit is unitary, from which it follows that
| S12 |2 |
+ | S13 |2 |
= 1 |
(6.2) |
|
| S12 |2 |
+ | S23 |2 |
= 1 |
(6.3) |
|
| S13 |2 |
+ | S23 |2 |
= 1 |
(6.4) |
|
S13* S23 |
= 0 |
(6.5) |
||
S23* S12 |
= 0 |
(6.6) |
||
S12* S13 |
= 0 |
(6.7) |
Figure 6.2 T-junctions: (a) a waveguide junction; (b) a microstrip line junction.
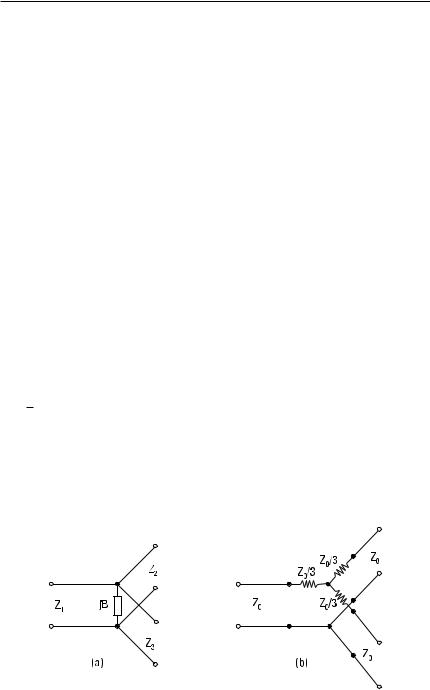
118 Radio Engineering for Wireless Communication and Sensor Applications
According to (6.5) through (6.7) at least two of the parameters S12 , S13 , and S23 are zero. However, this is in contradiction with at least one of (6.2) through (6.4). Thus, such a circuit cannot exist.
Let us consider the matching problem of a lossless T-junction with an equivalent circuit shown in Figure 6.3(a). The parallel susceptance B represents the reactive fields produced by the discontinuity of the junction. The characteristic impedances of the ports are Z 1 , Z 2 , and Z 3 . Let us assume that B = 0. We then choose Z 2 = Z 3 = 2Z 1 and assume that the ports are terminated with load impedances equal to their characteristic impedances. Now port 1 is matched: The input impedance is Z 1 and the power fed to port 1 is split evenly to the loads of ports 2 and 3. However, ports 2 and 3 are not matched because their input impedance is 2Z 1 /3 (Z 1 and 2Z 1 in parallel) instead of 2Z 1 .
All ports of a three-port divider can be matched by using resistive elements. Figure 6.3(b) shows a matched power divider containing lumped resistors. One half of the power coupled to the input port is absorbed in these resistors.
The isolation of the output ports of these power dividers is poor since a part of the power reflected from a mismatched output load is coupled to the other output port. The Wilkinson power divider shown in Figure 6.4 avoids this disadvantage. All the ports are matched to a characteristic impedance Z 0 if the quarter-wave-long sections have a characteristic impedance of √2Z 0 , and the output ports 2 and 3 are connected with a lumped resistor having a resistance 2Z 0 . This circuit is also lossless if the ports are terminated with a matched load, that is, with an impedance of Z 0 .
For all the power dividers discussed so far, the ratio of output powers equals 1. However, this ratio can be chosen freely by modifying the parameters of the circuits.
Figure 6.3 Equivalent circuits of power dividers: (a) lossless T-junction and (b) resistive power divider.
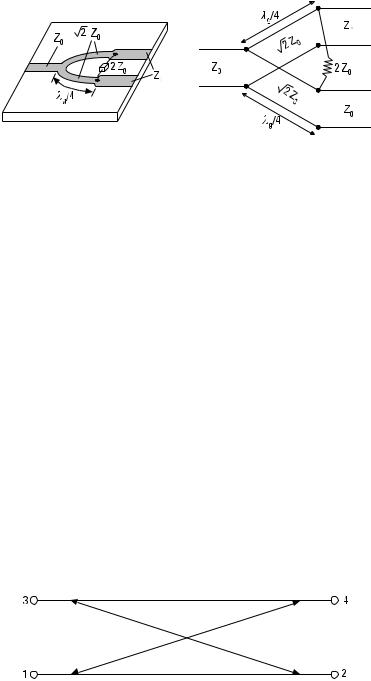
Passive Transmission Line and Waveguide Devices |
119 |
||
|
|
|
|
|
|
|
|
Figure 6.4 Wilkinson power divider.
6.1.2 Coupling and Directivity of a Directional Coupler
Let us consider the directional coupler shown in Figure 6.5 and assume that it is ideal. If a wave is fed from a signal source into port 1, it will couple to ports 2 and 4 but not at all to port 3. Similarly, if port 2 is the input port, ports 1 and 3 are output ports and port 4 is an isolated port. An ideal directional coupler is also lossless, and all of its ports can be matched. If all the ports are terminated with a matched load, the input reflection coefficients are zero. In practice, these properties can be achieved only approximately.
Let us assume that the power coupled into the input port 1 is P 1 and the powers coupled from ports 2, 3, and 4 to the matched terminations are P 2 , P 3 , and P 4 , respectively. The coupling C and directivity D in decibels are defined as
C = 10 log |
P 1 |
|
(6.8) |
P 4 |
|||
D = 10 log |
P 4 |
|
(6.9) |
P 3 |
Usually, most of the input power couples to the termination of the main line at port 2. The coupling describes the power coupled from the main line to the side line and is designed to a value that depends on the
Figure 6.5 Directional coupler.