
- •Radio Engineering for Wireless Communication and Sensor Applications
- •Contents
- •Preface
- •Acknowledgments
- •1 Introduction to Radio Waves and Radio Engineering
- •1.1 Radio Waves as a Part of the Electromagnetic Spectrum
- •1.2 What Is Radio Engineering?
- •1.3 Allocation of Radio Frequencies
- •1.4 History of Radio Engineering from Maxwell to the Present
- •2.2 Fields in Media
- •2.3 Boundary Conditions
- •2.4 Helmholtz Equation and Its Plane Wave Solution
- •2.5 Polarization of a Plane Wave
- •2.6 Reflection and Transmission at a Dielectric Interface
- •2.7 Energy and Power
- •3 Transmission Lines and Waveguides
- •3.1 Basic Equations for Transmission Lines and Waveguides
- •3.2 Transverse Electromagnetic Wave Modes
- •3.3 Transverse Electric and Transverse Magnetic Wave Modes
- •3.4 Rectangular Waveguide
- •3.4.1 TE Wave Modes in Rectangular Waveguide
- •3.4.2 TM Wave Modes in Rectangular Waveguide
- •3.5 Circular Waveguide
- •3.6 Optical Fiber
- •3.7 Coaxial Line
- •3.8 Microstrip Line
- •3.9 Wave and Signal Velocities
- •3.10 Transmission Line Model
- •4 Impedance Matching
- •4.1 Reflection from a Mismatched Load
- •4.2 Smith Chart
- •4.3 Matching Methods
- •4.3.1 Matching with Lumped Reactive Elements
- •4.3.4 Resistive Matching
- •5 Microwave Circuit Theory
- •5.1 Impedance and Admittance Matrices
- •5.2 Scattering Matrices
- •5.3 Signal Flow Graph, Transfer Function, and Gain
- •6.1 Power Dividers and Directional Couplers
- •6.1.1 Power Dividers
- •6.1.2 Coupling and Directivity of a Directional Coupler
- •6.1.3 Scattering Matrix of a Directional Coupler
- •6.1.4 Waveguide Directional Couplers
- •6.1.5 Microstrip Directional Couplers
- •6.2 Ferrite Devices
- •6.2.1 Properties of Ferrite Materials
- •6.2.2 Faraday Rotation
- •6.2.3 Isolators
- •6.2.4 Circulators
- •6.3 Other Passive Components and Devices
- •6.3.1 Terminations
- •6.3.2 Attenuators
- •6.3.3 Phase Shifters
- •6.3.4 Connectors and Adapters
- •7 Resonators and Filters
- •7.1 Resonators
- •7.1.1 Resonance Phenomenon
- •7.1.2 Quality Factor
- •7.1.3 Coupled Resonator
- •7.1.4 Transmission Line Section as a Resonator
- •7.1.5 Cavity Resonators
- •7.1.6 Dielectric Resonators
- •7.2 Filters
- •7.2.1 Insertion Loss Method
- •7.2.2 Design of Microwave Filters
- •7.2.3 Practical Microwave Filters
- •8 Circuits Based on Semiconductor Devices
- •8.1 From Electron Tubes to Semiconductor Devices
- •8.2 Important Semiconductor Devices
- •8.2.1 Diodes
- •8.2.2 Transistors
- •8.3 Oscillators
- •8.4 Amplifiers
- •8.4.2 Effect of Nonlinearities and Design of Power Amplifiers
- •8.4.3 Reflection Amplifiers
- •8.5.1 Mixers
- •8.5.2 Frequency Multipliers
- •8.6 Detectors
- •8.7 Monolithic Microwave Circuits
- •9 Antennas
- •9.1 Fundamental Concepts of Antennas
- •9.2 Calculation of Radiation from Antennas
- •9.3 Radiating Current Element
- •9.4 Dipole and Monopole Antennas
- •9.5 Other Wire Antennas
- •9.6 Radiation from Apertures
- •9.7 Horn Antennas
- •9.8 Reflector Antennas
- •9.9 Other Antennas
- •9.10 Antenna Arrays
- •9.11 Matching of Antennas
- •9.12 Link Between Two Antennas
- •10 Propagation of Radio Waves
- •10.1 Environment and Propagation Mechanisms
- •10.2 Tropospheric Attenuation
- •10.4 LOS Path
- •10.5 Reflection from Ground
- •10.6 Multipath Propagation in Cellular Mobile Radio Systems
- •10.7 Propagation Aided by Scattering: Scatter Link
- •10.8 Propagation via Ionosphere
- •11 Radio System
- •11.1 Transmitters and Receivers
- •11.2 Noise
- •11.2.1 Receiver Noise
- •11.2.2 Antenna Noise Temperature
- •11.3 Modulation and Demodulation of Signals
- •11.3.1 Analog Modulation
- •11.3.2 Digital Modulation
- •11.4 Radio Link Budget
- •12 Applications
- •12.1 Broadcasting
- •12.1.1 Broadcasting in Finland
- •12.1.2 Broadcasting Satellites
- •12.2 Radio Link Systems
- •12.2.1 Terrestrial Radio Links
- •12.2.2 Satellite Radio Links
- •12.3 Wireless Local Area Networks
- •12.4 Mobile Communication
- •12.5 Radionavigation
- •12.5.1 Hyperbolic Radionavigation Systems
- •12.5.2 Satellite Navigation Systems
- •12.5.3 Navigation Systems in Aviation
- •12.6 Radar
- •12.6.1 Pulse Radar
- •12.6.2 Doppler Radar
- •12.6.4 Surveillance and Tracking Radars
- •12.7 Remote Sensing
- •12.7.1 Radiometry
- •12.7.2 Total Power Radiometer and Dicke Radiometer
- •12.8 Radio Astronomy
- •12.8.1 Radio Telescopes and Receivers
- •12.8.2 Antenna Temperature of Radio Sources
- •12.8.3 Radio Sources in the Sky
- •12.9 Sensors for Industrial Applications
- •12.9.1 Transmission Sensors
- •12.9.2 Resonators
- •12.9.3 Reflection Sensors
- •12.9.4 Radar Sensors
- •12.9.5 Radiometer Sensors
- •12.9.6 Imaging Sensors
- •12.10 Power Applications
- •12.11 Medical Applications
- •12.11.1 Thermography
- •12.11.2 Diathermy
- •12.11.3 Hyperthermia
- •12.12 Electronic Warfare
- •List of Acronyms
- •About the Authors
- •Index

40 Radio Engineering for Wireless Communication and Sensor Applications
modes. Every mode has its own propagation characteristics: velocity, attenuation, and cutoff frequency. Because different modes propagate at different velocities, signals may be distorted due to the multimode propagation. Using the waveguide at low enough frequencies, so that only one mode—the dominant or fundamental mode—can propagate along the waveguide, prevents this multimode distortion.
3.2 Transverse Electromagnetic Wave Modes
In lossless and, with a good approximation, in low-loss two-conductor transmission lines, as in coaxial lines, fields can propagate as transverse electromagnetic (TEM) waves. TEM waves have no longitudinal field components. TEM waves may propagate at all frequencies, so the TEM mode has no cutoff frequency.
When Ez = 0 and Hz = 0, it follows from (3.5) through (3.8) that the x - and y -components of the fields are also zero, unless
g 2 + v 2me = 0 |
(3.13) |
Therefore, the propagation constant of a TEM wave is
g = ± jv √ |
|
= ± j |
2p |
= ± jb |
(3.14) |
|
me |
||||||
l |
||||||
|
|
|
|
|
The velocity v p is independent of frequency, assuming that the material parameters e and m are independent of frequency:
v p = |
v |
= |
1 |
(3.15) |
||
|
|
|
||||
b |
√me |
|||||
|
|
|
Thus, there is no dispersion, and the TEM wave in a transmission line propagates at the same velocity as a wave in free space having the same e and m as the insulator of the transmission line. The wave equations for a TEM wave are
=2 |
E = 0 |
(3.16) |
xy |
|
|
=2 |
H = 0 |
(3.17) |
xy |
|
|

Transmission Lines and Waveguides |
41 |
The fields of a wave propagating along the z direction satisfy the equation
E x |
= − |
E y |
= h |
(3.18) |
|
Hy |
Hx |
||||
|
|
|
where h = √m /e is the wave impedance.
Laplace’s equations, (3.16) and (3.17), are valid also for static fields. In electrostatics, the electric field may be presented as the gradient of the scalar transverse potential:
E (x , y ) = −=xy F(x , y ) |
(3.19) |
Because = × =f = 0, the transverse curl of the electric field must vanish in order for (3.19) to be valid. Here this is the case, because
=xy × E = −jvm Hz uz = 0 |
(3.20) |
where uz is a unit vector pointing in the direction of the positive z -axis. Gauss’ law in a sourceless space states that = ? D = e=xy ? E = 0. From this and (3.19) it follows that F(x , y ) is also a solution of Laplace’s equation, or
=2 |
F(x , y ) = 0 |
(3.21) |
xy |
|
|
The voltage of a two-conductor line is
|
2 |
|
|
|
V 12 = |
E |
E ? d l = F1 |
− F2 |
(3.22) |
|
||||
|
1 |
|
|
|
where F1 and F2 are the potentials of the conductors. From Ampe`re’s law, the current of the line is
I = RH ? d l |
(3.23) |
G |
|
where G is a closed line surrounding the conductor.

42 Radio Engineering for Wireless Communication and Sensor Applications
3.3Transverse Electric and Transverse Magnetic Wave Modes
A wave mode may have also longitudinal field components in addition to the transverse components. Transverse electric (TE) modes have Ez = 0 but a nonzero longitudinal magnetic field Hz . Transverse magnetic (TM) modes have Hz = 0 and a nonzero Ez .
From (3.4) it follows for a TE mode
=2 |
H |
z |
= −( g2 |
+ v 2me ) H |
z |
= −k |
2 |
H |
z |
(3.24) |
xy |
|
|
|
|
c |
|
|
Correspondingly, for a TM mode
=2 |
E |
z |
= −( g2 + v2me ) E |
z |
= −k |
2 |
E |
z |
(3.25) |
xy |
|
|
|
c |
|
|
The coefficient k c is solved from (3.24) or (3.25) using the boundary conditions set by the waveguide. For a given waveguide, an infinite number of k c values can usually be found. Each k c corresponds to a propagating wave mode. We can prove that in the case of a waveguide such as a rectangular or circular waveguide, in which a conductor surrounds an insulator, k c is always a positive real number.
The propagation constant is
g = √ |
|
|
k 2c − v 2me |
(3.26) |
If the insulating material is lossless, v 2me is real. The frequency at which v 2me = k2c is called the cutoff frequency:
f c = |
|
k c |
(3.27) |
||||
|
|
|
|
|
|||
2p√me |
|||||||
|
|
||||||
The corresponding cutoff wavelength is |
|
||||||
l c = |
2p |
|
(3.28) |
||||
k c |
|||||||
|
|
|
At frequencies below the cutoff frequency, f < f c , no wave can propagate. The field attenuates rapidly and has an attenuation constant
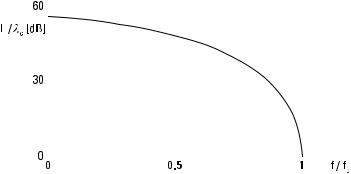
Transmission Lines and Waveguides |
43 |
|||||||
|
|
|
|
|
|
|
|
|
|
2p |
|
|
f |
2 |
|
||
g = a = |
√1 − S |
D |
(3.29) |
|||||
l c |
f c |
At frequencies much below the cutoff frequency, f << f c , the attenuation constant is a = 2p /lc or 2p nepers (54.6 dB) per cutoff wavelength. Figure 3.2 shows the attenuation at frequencies below the cutoff frequency.
At frequencies higher than the cutoff frequency, f > f c , waves can propagate and the propagation constant g is complex. In a lossless line, g is imaginary:
|
l |
√ |
|
|
|
|
|
|
|
S f |
D |
|
|||
|
2p |
|
|
|
f c |
2 |
|
g = jbg = j |
|
|
1 − |
|
|
|
(3.30) |
where l is the wavelength in free space composed of the same material as the insulator of the line. The wavelength in the line is
l g = |
2p |
= |
|
|
l |
|
|
(3.31) |
|
|
|
|
|
||||
|
√1 |
|
|
|
||||
|
bg |
− ( f c /f ) |
2 |
|||||
|
|
|
|
|
|
Figure 3.3 shows how the wavelength l g depends on frequency. The phase velocity (see Section 3.9) is
|
v p = |
|
|
v |
|||||
|
|
|
|
|
(3.32) |
||||
|
|
|
|||||||
|
|
|
|
||||||
|
|
√1 − ( f c /f )2 |
|||||||
|
|
|
|
|
|
|
|
|
|
|
|
|
|
|
|
|
|
|
|
|
|
|
|
|
|
|
|
|
|
|
|
|
|
|
|
|
|
|
|
|
|
|
|
|
|
|
|
|
|
|
|
|
|
|
|
|
|
|
|
|
|
|
|
|
|
|
|
|
|
|
|
|
|
|
|
|
|
|
|
Figure 3.2 Attenuation of TE and TM waves below the cutoff frequency in a lossless waveguide.