
The Art of Genes How Organisms Make Themselves
.pdf
arranged as right-handed helices? How can a protein distinguish left from right?
Fig. 14.18 Structure of collagen.
Recall that every protein is made up of a chain of subunits, called amino acids. Now each amino acid in turn consists of a carbon atom with four different molecules or atoms attached to it,* (* One amino acid, glycine, is an exception to this rule because two of the atoms attached to the carbon are the same (hydrogen).)forming a tetrahedron (Fig. 14.19). But notice that this arrangement has no planes of reflection symmetry: the mirror image of an amino acid cannot be superimposed on the original whichever way we try and turn it. There are therefore two potential forms for each amino acid, an L-form and a R-form (Fig. 14.19). If these were present in a 50:50 mix, there would be no overall handedness to amino acids. However, at an early stage of evolution, only one form of amino acid, the L-form, became predominantly used by organisms and essentially all amino acids involved in protein synthesis ended up being of this type. Exactly how this came about is still a matter of debate, but one important consequence has been that proteins can be made in a consistent shape because the same form of amino acid is always used. In principle, the R-form could have been used just as successfully as the L-form, and it may have been largely a matter of chance which form survived, just as whether we drive on the left or right side of the road is a matter of historical accident. It doesn't matter which side we drive on so long as everyone else does the same. For some countries (e.g. England) the convention is to always drive on the left whereas in others (e.g. America) it is the right, but a mixed strategy of sometimes driving on the left or right would be disastrous. In a similar way, interactions between molecules during evolution may have ensured that only one type of amino acid eventually came to predominate in the living kingdom (according to one view, this choice may itself have been biased by a handedness in physics, involving the properties of subatomic particles).
Fig. 14.19 Structure of two forms of amino acid.
When L-amino acids are strung together to make proteins, they often form helices. And because the amino acids are handed, so are the helices that they form. In the case of collagen, three strings of amino acids wind around each other to form a right-handed spiral: each of the individual fibres of collagen shown in Fig. 14.18 is a string of L-amino acids. The handedness of collagen therefore reflects the handedness of its component amino acids (DNA is a right-handed double helix for a similar reason: its subunits are handed molecules).
By stringing together small handed molecules, the L-amino adds, we end up with a handed helical protein of collagen. The collection of handed collagen proteins can in turn give a handed twist to the cuticle, leading to a worm wandering around in circles in a consistent direction. The final handedness we see in the mutant worm can be traced to a basic molecular handedness, which in turn may have been set by chance events early on in the evolution of life.
Handed painting
The case of the turning worm shows how a handed twist of the body might follow from molecular handedness, but what about more complex arrangements, such as the left-right asymmetry in our internal organs? In 1995, Michael Levin, Cliff Tabin and colleagues, working at the Harvard Medical School in Boston, made a remarkable observation. They were studying some genes for hidden colours (master proteins), scents (signalling proteins) and sensitivities (receptor proteins) in the early chick embryo, when they noticed that some of these genes were expressed mainly on the left side of the embryo, whereas others were on the right. In other words, there was a left-right asymmetry in the distribution of these gene activities at an early stage of development. Similar types of observation were then made for other vertebrate embryos, such as those of mice and frogs. This means that there are left-right distinctions in the early map of hidden colours and their associated scents and sensitivities.
This asymmetry is related to the left-right differences of internal organs that develop later on. For example, there is a mutant type of mouse in which the organs are always in a mirror image of the normal arrangement (sims inversus). The early embryos of such mice also tend to have the left-right distinctions in their hidden map reversed, so that genes expressed normally on the left are now found to be expressed on the right. These sorts of observation have led to the following model.
In addition to distinctions along the three axes previously mentioned (head-tail, dorsal-ventral, inside-outside), an asymmetric pattern of hidden colours is also established very early on from left to right. This results in distinctive colour combinations in the left and right halves of the embryo's patchwork that can be further interpreted and elaborated, eventually leading to left-right distinctions in internal anatomy. Our internal left-right asymmetry traces back to an asymmetry in the patchwork of hidden colours.
This begs the question of what the left-right differences in the patchwork themselves depend upon. The answer is not yet known but, as we have seen with the twisting worm, whatever they trace back to must itself be handed. Like the worm, the most obvious candidates are the handed building blocks of life, such as the L-amino acids. But instead of these molecules contributing to the handed movement of the whole animal, we need to imagine that they lead to differences in hidden colour between the left and right halves within the embryo. For example, handed proteins (made of L-amino acids) might lead to a particular scent being preferentially transported from the left to the right side of the embryo. Once left-right differences are established for one type of scent molecule they can then be responded to and further elaborated. (Essentially this sort of model was proposed by Nigel Brown and Lewis Wolpert in 1990, and it has recently received some experimental support from the finding that one of the genes affecting handedness in mice codes for a protein, dynein, involved in molecular transport.) The details are still far from clear but the key point is that the handedness of the patchwork most probably reflects the handedness of basic
molecules, such as L-amino acids. Perhaps all forms of biological handedness, from twining plants and spiral shells to the arrangement of our visceral organs, trace back, in one way or another, to the handedness of molecular building blocks like amino acids.
Might the same principles also underlie the bias towards right-handedness in humans? An early theory of human handedness was that it reflected the internal arrangement of organs. We now know that this is not the case because the vast majority of people, including the ten per cent or so of left-handers, have the same internal anatomy, with the heart on the left and the liver on the right. Hand preference is therefore not correlated with visceral anatomy. Instead, the bias has more to do with the way our brain works. We tend to think of our brain as a single structure, but it is actually divided into left and right halves or hemispheres, which can function differently. For example, in most people, speech is localised to the left hemisphere whereas visuo-spatial abilities are concentrated in the right hemisphere. The hemispheres also control our basic body movements in a cross-wise fashion: the left hemisphere controls the right side of the body (e.g. right arm, right leg) whereas the right hemisphere controls the left side. Because of this, predominance of right-handedness is thought to be due to a dominating effect of the left hemisphere on manual activities.
Our hand preference may therefore reflect an asymmetry in the way our brain develops: there is a bias that leads to manual dominance more often developing in the left hemisphere than the right. Nobody knows what the origin of this bias is, but it seems to me that one possibility is that it stems from molecular handedness. Just as handed molecules might bias the development of certain organs towards one side of our body, so they might also bias the way our brain develops. We would expect the particular molecules involved to be different for brain and visceral left-right asymmetry because these can be inverted independently of each other. Furthermore, the bias in the case of the brain may be weaker because cross-cultural studies indicate that about one in ten people are left-handed, whereas only about one in ten thousand people have fully inverted visceral organs. Nevertheless, the ability to distinguish right from left in both cases might trace back to the same sort of molecular handedness. If so, it would mean that the handedness of internal painting, the elaboration of hidden colours, and the handedness of human painting would have the same basis.
To summarise, the most fundamental type of asymmetry is along the inside-outside axis. All organisms express this in some form or other. In multicellular organisms, asymmetry along this axis can be elaborated during development, to give a pattern of distinctive hidden colours from inside to outside. This may interact with patterns along two further axes to give an overall patchwork with bilateral symmetry. In some cases, there is an additional left-right pattern of hidden colours that can be superimposed to give the patchwork an overall handedness. As with other cases of biological handedness, this most probably traces back to the handed nature of molecular building blocks.
We have seen how the overall geometry and symmetry of multicellular organisms depends on the elaboration of hidden colours along various axes. However, there is another fundamental aspect to the geometry of many organisms: internal repetition. A striking feature of many plants and animals is that they contain many repetitive elements or themes, such as the numerous leaflike organs of a plant, the segments of an insect, or the vertebrae of a mammal. In the next chapter, I want to explore the nature of this internal repetition and show how it is related to the other patterns and asymmetries we have encountered.
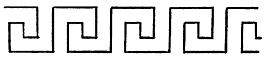
Chapter 15 Themes and variations
While I was at school in Liverpool, I was sometimes given 'lines' to write out as a form of punishment. Following some misdemeanour, I would be told to write a sentence like 'I must not use my ruler as a catapult' one hundred times. I would begin by writing the phrase at the top of a page and then write it again and again on the lines beneath, continuing down until the page was covered. In performing this task, a strange sort of satisfaction would start to emerge. There was something gratifying about seeing the same series of pen strokes running regimentally down the page, forming a regular pattern. In fact it was often quicker to form this pattern early on, by writing each word in turn as a vertical series. Written out in this way, the sentences lost their meaning and the exercise became one of making an almost abstract pattern of regular marks.
It seems that simply repeating something many times can lead to a certain type of symmetry. The same principle underlies the appeal of many friezes and wallpaper patterns. Even a basic design can be rendered quite attractive by the simple act of repetition (Fig. 15.1).
Fig. 15.1 Simple repetitive design.
As we shall see, repetition is also a fundamental feature of the living world. In this chapter I want to look at how repetition can arise during the development of organisms and how it relates to the types of symmetry covered in previous chapters.
Translational symmetry
The type of symmetry displayed when a sentence or design is repeated is known as translational symmetry. Remember that the symmetry of an object can be defined by the number of different transformations that can be applied to it that leave it unchanged (Chapter 13). We have already come across rotational symmetries, in which an object is left unchanged when it is turned; and reflection symmetries, in which appearances are preserved following reflection in a plane. An object with translational symmetry doesn't change when you move or shift it along in a straight line by a certain amount. Imagine, for example, that the design in Fig. 15.1 carried on for an infinite distance to the left and right. If you were to shift the pattern along by one length of the repeating unit, you would end up with exactly the same pattern again. In other words, you would not be able to tell that such a transformation, called a translation, had been applied by simply looking at the outcome. Similarly, an infinitely long page of written 'lines' would not be altered by shifting it up or down by one line. Strictly speaking, only infinite patterns show this sort of symmetry. If the repeating pattern is of a limited length you could tell that it had been shifted along by observing the movement of its ends. Nevertheless, for our purposes it will be useful to apply translational symmetry in a looser sense to include finite as well as infinite repeating patterns. After all, you can always imagine converting a finite pattern into an infinite one by extending it indefinitely.
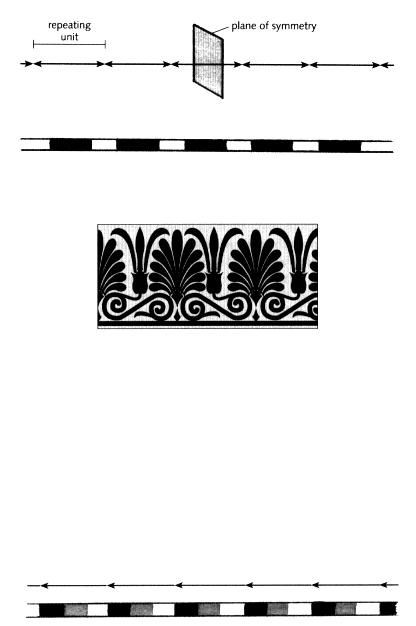
With this notion of translational symmetry in mind, we can distinguish between two basic types of repeating pattern. Look at the simple linear design at the top of Fig. 15.2. As well as showing translational symmetry along the horizontal axis, the pattern has a vertical plane of reflection symmetry running down the middle. That is to say, the left and right halves of the design are mirror images of each other. If the pattern was repeated indefinitely, there would be a similar plane at regular intervals all the way along (the interval between the planes of symmetry would be half the length of the repeating unit). Such patterns can be said to show no polarity because they look the same either way round. A band of two alternating colours also has this sort of symmetry, with the mirror planes running down the middle of each colour block (Fig. 15.2, bottom). Another good example is the Greek palmette motif shown in Fig. 15.3 (taken from Hermann Weyl's book on symmetry).
Fig. 15.2 Translational symmetry with no polarity
Fig. 15.3Palmette design showing translational symmetry with no polarity.
The second type of repeating pattern has no such planes of symmetry. For example, if the pattern shown at the top of Fig. 15.4 is reflected in any vertical plane, the direction of the arrows will be reversed, allowing you to tell that the transformation has been applied. It therefore has a lower degree of symmetry than the previous examples because there are fewer ways of transforming it into itself. Such patterns can be said to exhibit polarity. A banding pattern of three different colours, shown at the bottom of Fig. 15.4, has this sort of symmetry because any reflection at right angles would invert the order of the colours. Other examples are the design in Fig. 15.1 and written 'lines' on a page.
Fig. 15.4 Translational symmetry with polarity

All cases of translational symmetry fall into one or other category, either having polarity or not. A stack of similar coins oriented in the same way, say heads up and tails down, would have translational symmetry with polarity; each coin would be related to the next by a vertical shift of one coin's thickness, and the direction each coin was facing would be inverted by reflection in any plane at right angles to this (a horizontal plane in this case). By contrast, a stack of double-headed coins (coins with heads on both sides), would form a pattern showing translational symmetry without polarity.
A good example of translational symmetry in the living world is provided by the segments of centipedes and millipedes (Fig. 15.5). You can see from the orientation of the legs that these repeating patterns also have polarity: if you were to reflect the animal in a plane across it, the segments would end up pointing the opposite way. Leaves on most plants are also arranged in a repetitive way, although they usually display a more complicated pattern than pure translational symmetry. In some plants the leaves are arranged as a spiral or helix up the stem. To get from one leaf to the next you need to shift up the stem (translation) and move part way round its circumference (rotation). That is to say, the pattern remains unchanged following a combined translation and rotation. In other cases, as with the begonia shown in Fig. 15.6, subsequent leaves are related by a translation up the stem followed by reflection in a longitudinal plane. Most types of leaf arrangement involve a combination of translation, rotation and/or reflection symmetry (they also show polarity because the upper surface of a leaf is usually quite different from the lower surface).
Fig. 15.5 Centipede (Scolopendrid) showing translational symmetry of segments.
Fig. 15.6 Arrangement of leaves on a begonia plant, in which subsequent leaves are related by a combined translation along the stem followed by a reflection in a longitudinal plane
All these examples involve repetition of the same or very similar units. But there is also a broader category of patterns in which repeating units vary to some extent from one to another. Suppose, for example, that instead of identical coins we stack a mixed set of coins of different
denominations. The coins can still be stacked with the same polarity (heads facing up and tails down) but there is now no translational symmetry because each coin is distinct from its neighbour. Furthermore, each coin may have a slightly different thickness so that there is no standard length for shifting them up or down. Nevertheless, all the units in the stack share some features bec ause they are all easily recognisable as coins. One way of describing the situation would be to say that there is a basic theme that is repeated up the stack— the general notion of a coin— but there are also important variations on this— the particular denominations.
The same point can be illustrated with a queue of people. If all the people in a queue are identical and stand at perfectly repeated intervals, the queue would show translational symmetry. But in real queues, each person is different from the next, so there is no strict symmetry of this type. Nevertheless, we can still recognise a repetitive structure in the queue, which might be expressed by saying there is a basic theme— the general notion of a person— that is repeated along the queue, together with numerous variations upon it— the particular characteristics of each individual in the queue. In all these cases a basic type of unit, such as a coin or person, is repeated in a linear sequence but the repeating units are not identical.
A fruit fly can also be thought of as exhibiting variations on a theme. We talk about a fruit fly as being made up of repeating segments even though they are clearly not all the same: some have legs, some also have wings, yet others have no appendages. It is as if the fly displays a repeated theme, the segment, combined with variations upon it. The same might be said about the different whorls of organs in a flower, or the fore and hind limbs of a vertebrate: they are all examples of variations on a theme. In previous chapters we have looked at how such variations might arise. We saw, for example, that the variations between the segments of a fly reflect a series of hidden colours (various types of green) that vary from head to tail. But I said nothing about what the segments have in common, the nature of the underlying theme. I now want to look at this problem, but before doing so it will help to distinguish between two types of theme.
Explicit and implicit themes
In 1957, Pablo Picasso produced a series of paintings inspired by one of the masterpieces of art, Las Meninas (The Maids of Honour), painted about three hundred years earlier by Diego Velázquez. Velázquez's painting shows a girl of the Spanish royal family, attended by her maids of honour (Fig. 15.7, top). The girl has come to watch the Court painter, Velázquez himself, at work in his studio. Picasso was fascinated by the picture and produced a series of paintings based upon it. Some of Picasso's paintings are based on the whole of Velázquez's picture (Fig. 15.7, bottom); others concentrate on a detail such as the girl alone (Fig. 15.8). Once you know the history of the paintings, you can almost see the original Velázquez within them, even though on the surface they look so different.
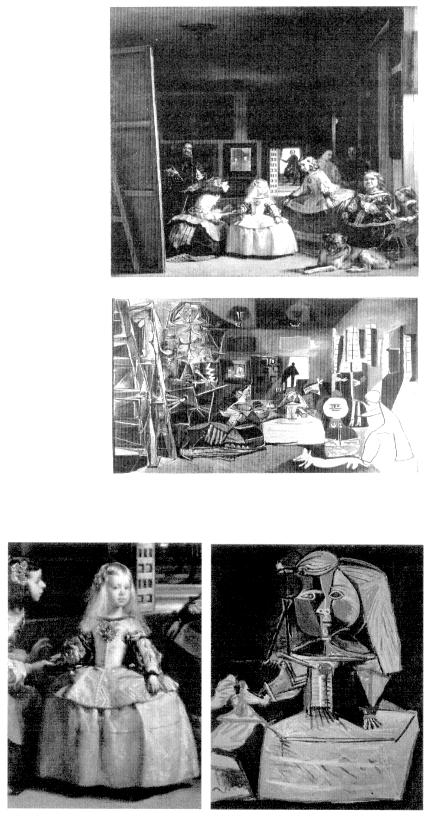
Fig. 15.7Las Meninas by Velázquez, above (1656, Prado, Madrid) compared to version by Picasso, below (1957, Museo Picasso, Barcelona).
Fig. 15.8Detail from Las Meninas by Velázquez, left (1656, Prado, Madrid) compared to picture by Picasso, right (1957, Museo Picasso, Barcelona).
This series of paintings by Picasso might be said to represent variations on a theme. The theme is the original painting by Velázquez whereas the variations are the different versions of it that Picasso came up with. Although the theme and its variations are all paintings, there is no problem in distinguishing between them. The theme dearly preceded the variations and was their source of inspiration rather than the other way round.
But there is also another theme that unites Picasso's paintings: they have a common style. They are all examples of Picasso's art and vision. In a sense this is a more important theme because anyone can look at a Velázquez and produce a series of paintings, but only Picasso could have produced the works that we see in his Las Meninas series. Yet defining this theme, Picasso's style, is not so easy. You might look at many examples of paintings by Picasso and recognise some unity, but it would be difficult to say precisely what they all have in common, what makes them all identifiable as works of Picasso.
Picasso's Las Meninas series illustrates two basic types of theme. On the one hand, there is the theme provided by the Velázquez. We can point to Velázquez's original painting as distinct from the variations based upon it. We might say that in this case the theme is quite explicit and precedes the variations. By contrast, the other type of theme, the stylistic unity of Picasso, is implicit. It seems to be locked within each of the variations rather than being a separate painting we can point to. Unlike an explicit theme, there is no implied direction here: any two Picasso paintings are equivalent expressions of Picasso's style, rather than one being derived from the other.
The leaf
Much confusion about the nature of repetition in the living world has come from confounding implicit with explicit themes. A good illustration of this concerns Goethe's idea that all the appendages of a plant are equivalent to leaves. Recall that he proposed that all the organs of a plant were variations on a theme: sepals, petals, stamens, carpels and foliage leaves may look different from each other but they share an underlying similarity. Goethe wanted a term to describe the theme that unified the various organs of a plant and he chose to use the word leaf:
It goes without saying that we must have a general term to indicate this variously metamorphosed organ, and to use in comparing the manifestations of its form; we have hence adopted the word leaf. But when we use this term, it must be with the reservation that we accustom ourselves to relate the phenomena to one another in both directions. For we can just as well say that a stamen is a contracted petal, as we can say of a petal that it is a stamen in a State of expansion. And we can just as well say that a sepal is a contracted stem-leaf ... as that a stem-leaf is a sepal.
Goethe was using the word leaf in an unusual way, to refer to the implicit theme behind all organs. There was no implied direction: a comparison between any pair of organs, like a stamen and a petal, or a sepal and a foliage leaf, could always be made both ways. This equivalence could be summarised by saying that they are variations on the same theme, all different types of leaf. Unfortunately, in choosing the word leaf to denote the underlying theme, he caused major confusion because we also use leaf to indicate one of the variations, namely foliage leaves. By saying that all organs were essentially leaves, he did not mean to imply that leaves, in the narrow sense of green foliage leaves, were an explicit theme upon which everything was based. He was
using leaf in a more implicit sense, as a theme common to foliage leaves and floral organs. His notion of leaf was more like the stylistic unity of Picasso's Las Meninas paintings than the distinct Velázquez that preceded and inspired them.
Even though he was very careful to point this out, it did not stop people misinterpreting his theory. Many botanists believed Goethe to be saying that the different parts of a flower were derived from leaves in the common sense of foliage (this mistake is still sometimes made even today). The green leaf of a flowering plant was taken to be the template that everything else was based upon. After Goethe died, this same misinterpretation surfaced again but in a slightly different way, under the guise of evolution. As people became convinced of Darwin's theory of evolution in the late nineteenth century, they started to consider what the ancestors of flowering plants might have looked like. If the floral organs were indeed modified foliage leaves, as they thought Goethe was saying, it would seem that leaves came first and flower parts evolved from them secondarily. The implication was that ancestral plants must have had only foliage, and flowers then evolved through the modification of this ancient greenery.
The trouble is that if these ancestors had only had foliage leaves, they would have been completely sterile. A rose leaf cannot produce sperm or egg cells, no matter how long you wait. Nobody was around to witness the ancestors of flowering plants but it is extremely unlikely that they were sexually sterile. Sex is one of the oldest and most ubiquitous inventions of evolution and greatly predates the arrival of flowering plants. It is therefore highly improbable that the early ancestors of flowering plants had only sterile organs. They are more likely to have been similar to modern fern plants. Ferns do not have flowers but they do reproduce sexually. They achieve this through the production of sexual spores from their green fronds or 'leaves'. These spores will eventually give rise to sperm and egg cells which can fuse to produce the next generation of fern plants. In other words, the fronds on a fern resemble both foliage leaves, being green and spread out to collect the energy from the sun; and flowers, being the route to sexual reproduction. In flowering plants there is a separation between organs involved in nourishment (foliage) and sex (flowers). It seems likely that this is a later specialisation, and that both functions were carried out by the single type of organ in their early ancestors.
Some botanists therefore concluded that Goethe must have been wrong when he said that the parts of the flower were modified or metamorphosed leaves. It seemed to imply that ancestral plants were just sterile foliage, which was obviously incorrect. As the botanist F. O. Bower said in 1911: 'There is no need now for any theory of metamorphosis to explain the origin of the Flower. As first stated by Goethe it was a theory of mysticism, which was doomed to dissolution.' But this is because Goethe's notion of leaf was taken to be an explicit theme from which other organs were derived. Goethe was actually using leaf to denote an implicit theme that was simply manifested in different ways in the various parts of the plant. According to this viewpoint, the leaves of ancestral plants would have represented yet other variations on the same theme.
Even when taken on his own terms, though, Goethe's view seems to lead to an impasse. What exactly is a leaf, this common theme that is continually being depicted by the plant? There seems to be no meaningful way to pursue the problem other than by saying it is that which is common to all plant organs. This just seems to be evading the issue. It was for such reasons that Goethe's ideas were often considered to be mystical idealism rather than serious science.
Is there any way of getting beyond this impasse? Although the stylistic unity of Picasso's paintings is difficult to describe, we know where its origin lies: with Picasso himself. The implicit