
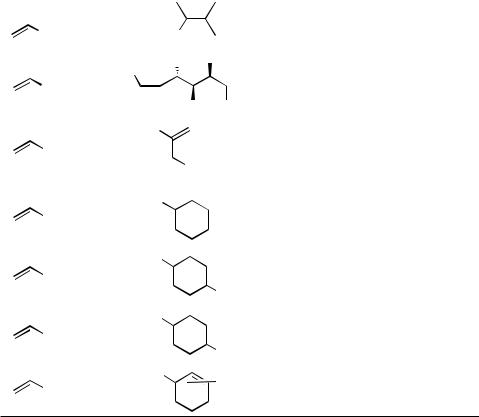
|
|
|
|
|
III.2.6 |
Pd-CATALYZED ALKENYL–ARYL |
375 |
|||
TABLE 9. (Continued ) |
|
|
|
|
|
|
|
|
|
|
|
|
|
|
|
|
|
|
|
|
|
Type of |
|
|
|
|
|
|
|
Yield |
|
|
Alkenyl |
|
|
|
|
|
|
(Selectivity) |
|
Ref- |
|
|
|
|
|
|
|
|
||||
M Electrophiles |
|
X |
|
Conditions |
|
% |
erence |
|||
|
|
|
|
|
|
|
|
|
|
|
|
|
Me |
Me |
|
|
|
|
|
||
I |
|
|
|
|
|
Pd(PPh3)4, THF |
|
|
|
|
ZnBr |
|
|
|
|
|
|
79 |
[187] |
||
|
|
|
|
|
|
|||||
|
|
|
|
|
|
|
|
|
|
|
|
|
BMPO |
OTBS |
|
|
|
|
|
||
α- |
I |
|
Cl2Pd(MeCN)2, |
|
|
|
|
|||
|
|
|
|
|
|
|
60 |
[177] |
||
SnBu3 |
|
|
|
|
|
DMF |
|
|||
|
|
|
|
|
|
|
|
|
||
|
|
|
CO2H |
|
|
|
|
|
||
(E )-α,β- |
|
|
|
|
|
|
|
|
|
|
|
I |
|
[ClPd(π-C3H5)]2, |
|
|
|
|
|||
SiMe3 |
|
|
|
|
|
|
100 |
|
[50] |
|
|
|
|
|
|
TASF, P(OEt)3, THF |
|
||||
|
TfO |
|
|
|
|
|||||
|
|
Cl2Pd(MeCN)2, |
|
|
|
|
||||
SnMe3 |
|
|
|
|
|
|
80 |
[184] |
||
|
|
|
|
|
|
|||||
|
|
|
|
|
DMF, 25 °C |
|
||||
|
|
|
|
|
tBu |
|
|
|
|
|
|
|
|
|
|
|
|
|
|
|
|
|
TfO |
|
Pd(PPh3)4, |
|
|
[188], |
||||
SnBu3 |
|
|
|
|
|
|
91 |
|||
|
|
|
|
|
|
|||||
|
|
|
|
|
LiCl, THF |
|
[189] |
|||
|
|
|
|
tBu |
|
|
||||
|
|
|
|
|
|
|
|
|
|
|
|
TfO |
Fe(CO)3 |
Cl2Pd(PPh3)2, |
|
|
|
|
|||
SnBu3 |
|
|
|
|
|
81 |
[190] |
|||
|
|
|
|
|
LiCl, NMP |
|
a The entries are arranged according to the structural types of alkenyl electrophiles summarized in Scheme 5. With the same category, they are arranged (i) according to the types of substituents, i.e., alkyl > alkenyl > aryl > alkynyl, and (ii) in the increasing order of priority determined by the Chan–Ingold–Prelog rule. Finally, the leaving groups and then the countercations are arranged as discussed in the text.
D.ii. (E)- -Sustituted Alkenylmetals
Alkenylmetals containing Mg, Zn, B, Al, Si, Sn, and Zr have been successfully coupled with alkenyl electrophiles, mainly halides and triflates. Alkenyl electrophiles of all eight possible structural types have been used in these coupling reactions as shown in Table 10.
Earlier systematic investigations on the Pdor Ni-catalyzed alkenyl–alkenyl crosscoupling addressed several fundamentally important issues[15][16][92] that are still critically important, such as stereochemistry, comparison of different metal countercations, comparison of Pdand Ni-catalysts, and hydrometallation–cross-coupling tandem process for the conversion of alkynes to stereoand regio-defined conjugated dienes. It should be recalled that palladium catalysts have been shown to be superior to nickel catalysts in terms of stereoselectivity in the alkenyl–alkenyl coupling, permitting nearly 100% retention of the stereochemistry of each alkenyl group. On the contrary, stereochemical scrambling has been observed to significant extents in the corresponding Ni-catalyzed reactions. Generally, alkenylmetals containing Zn and Mg display the highest intrinsic reactivity in a given

|
|
Reference |
Yield |
(Selectivity) |
% |
a |
|
|
Electrophiles |
|
Conditions |
Couplingof(E)Substituted--AlkenylmetalswithAlkenyl |
|
|
|
|
|
Typeof Alkenyl Electrophile X |
||
Pd-Catalyzed |
|
M |
TABLE10. |
|
R |
[191] |
[17] |
[192] |
[164] |
[164] |
− |
77(>97) |
95 |
70 |
90 |
3 |
3 |
3 |
, |
equiv)N(8 |
, |
equiv)N(8 |
Pd(PPh |
Pd(PPh |
|||||
|
|
|
2 |
|
2 |
|
|
|
|
) |
|
) |
|
, |
, |
, |
3 |
|
3 |
|
4 |
4 |
4 |
|
|
|
|
) |
) |
) |
|
|
|
|
Pd(PPh NaOH |
Pd(PPh THF |
Pd(PPh THF |
Cl |
Et |
Cl |
Et |
|
|
|
2 |
3 |
2 |
3 |
11 |
11 |
H |
H |
5 |
5 |
C |
C |
[193]
87
,BHA, |
EtOH |
3 |
|
4 |
|
) |
NaOEt, |
Pd(PPh |
OTHP
8
( )
[88]
58
, |
|
2 |
|
Pd(OAc) |
NaOH,THF |
13 H 6 C
[194],[195]
86(~100)
|
6 |
, |
H |
4 |
6 |
3 |
C |
) |
NaOEt, |
Pd(PPh |
13 H 6 C
[196] |
[15] |
86(>98) |
74(>98) |
|
+ |
|
2 |
|
) |
, |
3 |
3 |
Pd(PPh |
4 |
|
) |
Cl DIBAH |
Pd(PPh THFNaOH, |
|
|
2 |
Ph |
9 |
4 |
|
|
H |
|
C |
Br |
Br |
Br |
Cl |
Cl |
Br |
I |
Br |
Br |
I |
Vinyl |
|
|
|
(E)-β- |
|
|
|
|
|
|
|
|
|
|
|
|
|
|
2 |
Cl |
|
|
|
|
|
|
|
|
|
|
|
|
|
|
|
|
|
B(Sia) |
2 |
|
|
|
|
|
|
|
|
Cl |
|
|
|
|
|
|
|
Bu |
ZrCp |
|
|
ZnBr |
MgBr |
MgBr |
|
|
|
O |
|
O |
O B |
O |
|||||
2 |
CO |
|
O B |
O |
SiMe |
B |
Al |
|||||||||||
|
|
|
|
|
|
|
|
|
|
|
|
|
|
|
|
|
|
2 |
|
|
|
|
|
|
|
|
|
|
2 |
|
|
|
|
|
|
|
i |
|
|
Et |
|
|
|
|
|
|
|
|
|
|
|
|
|
|
|
|
|
|
2 |
|
|
|
|
|
|
|
|
|
|
|
|
|
|
|
|
() |
|
|
|
|
|
|
|
|
|
|
|
|
|
|
|
|
|
|
THPO |
THPO |
|
|
|
Me |
Me |
Et |
|
Bu |
Bu |
|
|
Bu |
|
C |
|||
|
|
|
|
|
|
|
|
|
|
|
|
|
|
|
|
|
|
11 |
|
|
|
|
|
|
|
|
|
|
|
|
|
|
|
|
|
|
H |
|
|
|
|
|
|
|
|
|
|
|
|
|
|
|
|
|
|
5 |
376
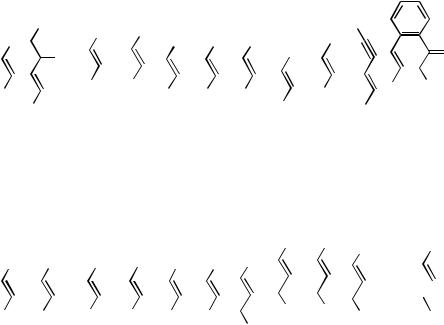
[17],[92] |
[197] |
[82] |
[198] |
91(>97) |
94(99.9) |
71(>99) |
80(>99) |
|
|
, |
, |
|
|
|
|
2 |
|
|
|
Pd(PPh THF |
Pd(PPh THF |
)] |
3 |
THF |
Ni(PPh THF |
[ClPd(π-C |
P(OEt)TBAF, |
||||
|
|
5 |
|
|
|
|
|
H |
|
|
|
, |
, |
3 |
|
|
, |
4 |
4 |
|
|
|
4 |
) |
) |
|
|
|
) |
3 |
3 |
|
|
|
3 |
C |
() |
7OAc |
OZnX |
Ph |
Cl |
9 |
|
|
|
|
|
H |
|
|
|
|
|
4 |
|
|
|
|
|
I |
|
|
|
Br |
Cl |
|
I |
|
|
|
|
[92]
95
, 4 ) 3 Pd(PPh THF
9 H 4 C
I
[199] |
[200] |
[201] |
74(99) |
66 |
50 |
|
|
6 |
|
6 |
, |
, |
H |
, |
H |
4 |
4 |
6 |
4 |
6 |
3 |
3 |
C |
3 |
C |
) |
) |
NaOEt, |
) |
NaOEt, |
Pd(PPh NaOH |
Pd(PPh |
Pd(PPh |
9 |
7 |
OH |
5 |
||
H |
H |
) |
4 |
3 |
2 |
C |
C |
(CH |
I |
I |
|
|
|
I |
(E)-β-
[92]
82(>98)
+ |
|
2 |
|
) |
|
3 |
|
Pd(PPh |
DIBAH |
Cl |
|
2 |
|
t-Bu
I
[202]
23(>98)
, |
O |
4 |
2 |
3 |
H |
) |
|
Pd(PPh |
NaOH, |
11 H 5 C
Br
Br
[203]
54
, 4 ) 3 Pd(PPh
( )
(Continued )
toulene
O
3
C 2 MeO
|
|
2 |
Cl |
|
Pr) |
ZrCp |
ZnBr |
SiMe(O |
2 |
|
|
11 |
11 |
13 |
H |
H |
H |
5 |
5 |
6 |
C |
C |
C |
AlBu |
ZnCl |
2 |
B(OH) |
||
2 |
|
|
13 |
17 |
17 |
H |
H |
H |
6 |
8 |
8 |
C |
C |
C |
( )
2 B(OH)
9 HO
( )
2 B(OH)
3
C 2 MeO
( )
2 B(OH)
3
C 2 MeO
( )
2 B(Sia)
2 THPO
3 SnBu
OTHP
17 H 8 C
377

TABLE 10. (Continued )
|
|
Reference |
Yield |
(Selectivity) |
% |
Conditions
Electrophile X
Alkenyl
Type of
M
R
[50] |
[176] |
78 |
77 |
, |
|
|
|
2 |
, |
|
|
)] |
3 |
THF |
Pd(PPh TBAF,THF, dioxane |
[ClPd(π-C |
P(OEt)TASF, |
||
5 |
|
|
|
H |
|
|
4 |
3 |
|
|
|
|
|
|
, |
|
|
|
) |
|
|
|
3 |
13 |
9 |
H |
H |
6 |
4 |
C |
C |
I |
I |
|
I |
|
2 |
|
] |
SiMe |
2 |
Sn[N(TMS) |
|
3 |
|
|
Ph |
[50] |
[184] |
32 |
71(86) |
, |
|
|
, |
|
2 |
, |
|
|
|
)] |
|
2 |
|
|
3 |
3 |
THF |
Pd(MeCN) |
DMF |
C[ClPd(π- |
P(OEt)TASF, |
Cl |
||
5 |
|
|
|
|
H |
|
|
|
|
|
|
|
2 |
|
Ph |
Ph |
I |
I |
3 |
3 |
SiMe |
SnMe |
Ph |
Ph |
[185]
53
, |
|
2 |
|
Pd(MeCN) |
DMF |
Cl |
|
2 |
|
|
9 |
|
H |
|
4 |
|
C |
Ph |
4 |
I |
BF |
+ |
– |
3 SnMe
Ph
[185] |
[193] |
69 |
87 |
73 |
|
|
− |
2 |
BHA, |
|
, |
|
EtOH |
Pd(MeCN) |
3 |
|
|
, |
|
|
4 |
|
Cl DMF |
) |
NaOEt, |
Pd(PPh |
||
2 |
|
|
|
4 |
|
|
OTHP |
|
9 |
|
|
|
|
H |
|
|
|
|
C |
|
) |
8 |
|
|
|
( |
|
Ph |
|
4 |
|
|
|
|
|
||
I |
BF |
Br |
|
|
+ |
– |
|
|
(Z)-β-
3 |
|
|
SnBu |
O B |
O |
Ph |
Et |
|
[200]
91
|
6 |
, |
H |
4 |
6 |
3 |
C |
) |
NaOEt, |
Pd(PPh |
OH
9 )(
Br
O |
O |
|
B |
7 |
|
H |
|
3 |
|
C |
|
378

[197]
88(99)
, 4 ) 3 Pd(PPh THF
Me 2 CO
8
OH |
() |
|
|
|
|
|
|
|
|
Br |
ZnBr
11 H 5 C
[199]
74(99)
3 |
THF |
, |
|
4 |
|
) |
|
Pd(PPh |
NaOH, |
9 H 4 C
I
2 B(OH)
17 H 8 C
[92]
55(>98)
+ |
|
2 |
|
) |
|
3 |
|
Pd(PPh |
DIBAH |
Cl |
|
2 |
|
9 H 4 C
I
2 Bu i Al
17 H 8 C
[200] |
[204] |
82 |
54 |
|
6 |
, |
|
|
2 |
|
|
, H |
) |
THF |
|
3 |
C |
Pd(PPh |
|
4 |
6 |
3 |
|
) |
NaOEt, |
|
NaOH, |
Pd(PPh |
Cl |
||
|
|
2 |
|
|
|
11 |
|
|
|
|
H |
|
OTBS |
5 |
|
3 |
|
C |
|
7 |
|
|
|
H |
|
|
|
C |
|
|
|
|
|
|
|
|
|
|
|
Br |
|
Br |
[205] |
[92] |
40−50 |
36(97) |
|
|
+ |
|
6 |
, |
|
2 |
|
, |
H |
) |
4 |
6 |
3 |
3 |
C |
Pd(PPh |
) |
NaOEt, |
Cl DIBAH |
Pd(PPh |
||
|
|
2 |
|
|
Ph2 |
|
() |
|
|
9 |
|
|
|
H |
|
4 |
|
Br |
|
C |
|
||
|
I |
|
|
α-
|
B(OH) |
B(Thx) |
BX |
Al |
|
2 |
|
|
|
|
|
|
|
2 |
|
|
|
2 |
Bu |
|
|
|
|
i |
|
|
7 |
|
|
|
|
() |
|
|
) |
9 |
C |
|
|
( HO |
2 |
|
|
|
MeO |
|
t-Bu |
[196] |
[82] |
80(~100) |
31(>99) |
|
|
, |
, |
|
|
|
2 |
|
|
Pd(PPh |
NaOEt,C |
)] |
3 |
|
[ClPd(π-C |
P(OEt)TBAF, |
THF |
||
|
6 |
5 |
|
|
, |
H |
H |
|
|
4 |
6 |
3 |
|
|
) |
|
|
|
|
3 |
|
|
|
|
|
5 |
|
H |
|
6 |
|
C |
|
2 |
Ph |
CH |
Br |
Br |
|
|
|
2 |
|
|
|
Pr) |
|
|
|
i |
|
|
|
SiMe(O |
O B |
O |
||
9 |
|
13 |
|
H |
|
H |
|
4 |
|
6 |
|
C |
|
C |
[206] |
(Continued) |
58
,
4 ) 3 THF Pd(PPh Cs,
H O
TfO
3 SnBu
Me
C 2 MeO
379

TABLE 10. (Continued )
|
|
Reference |
Yield |
(Selectivity) |
% |
Conditions
Electrophile X
Alkenyl
Type of
M
R
[177] |
[177] |
50 |
82 |
, |
|
, |
|
2 |
|
2 |
|
Pd(MeCN) |
DMF |
Pd(MeCN) |
DMF |
Cl |
Cl |
||
2 |
|
2 |
|
H |
H |
2 |
2 |
CO |
CO |
2 |
2 |
CH |
CH |
I |
I |
[192]
93
, 4 ) 3 Pd(PPh THF
3 CH
Br
81(~100) [194],[196]
|
6 |
, |
H |
4 |
6 |
3 |
C |
) |
NaOEt, |
Pd(PPh |
|
3 |
3 |
CH |
CH |
|
|
Br |
β,β- |
cis-α,β- |
[91],[138]
76
3 |
dioxane |
, |
|
4 |
|
) |
K |
Pd(PPh |
|
|
, |
|
4 |
|
PO |
|
3 |
TfO
3 |
|
|
|
ZnBr |
|
|
|
|
|
|
|
SnBu |
SnBu |
CO |
|
O B |
O |
O |
B |
O |
|||
|
3 |
|
|
|
|
|
|
|
|
|
|
|
|
Et |
|
|
|
|
|
|
|
|
|
|
|
2 |
|
|
|
|
|
|
|
|
|
OEt |
|
|
|
|
9 |
|
|
|
|
|
|
|
|
|
|
|
|
|
|
|
|||
|
|
|
|
|
|
|
|
|
|
||
EtO |
Ph |
|
|
|
H |
|
9 |
|
|
||
|
|
|
C |
|
C |
|
|
||||
|
|
|
|
|
4 |
|
H |
|
|
||
|
|
|
|
|
|
|
|
4 |
|
|
[53] |
[180] |
80(100) |
81(>98) |
Pd/C,10% |
3 |
, |
DMF |
AsPhCuI, |
Pd(PPh |
||
|
|
4 |
|
|
|
) |
|
|
|
3 |
OH |
|
2 |
|
CH |
3 |
TfO |
CH |
I |
|
trans-α,β- |
|
SnBu |
ZnCl |
3 |
|
|
9 |
Ph |
H |
C |
|
|
4 |
380
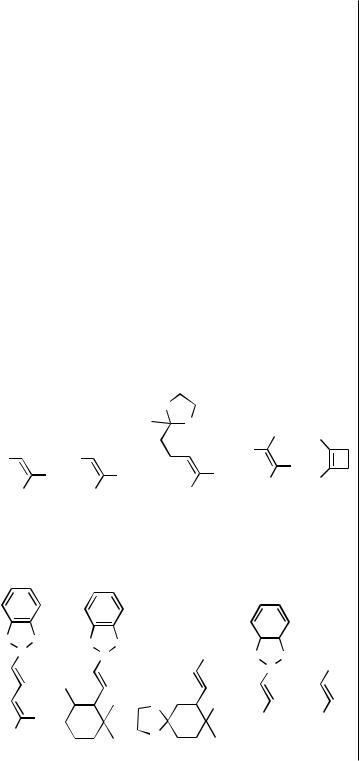
[207] |
[180] |
51 |
45(>96) |
|
|
6 |
, |
, |
H |
4 |
4 |
6 |
3 |
3 |
C |
) |
) |
NaOEt, |
Pd(PPh NaOEt |
Pd(PPh |
3 |
|
OH |
|
|
2 |
|
|
CH |
3 |
CH |
3 |
|
CH |
|
CH |
|
Br |
|
I |
[208] |
[91],[138] |
52 |
97 |
3 |
C |
3 |
dioxane |
|
6 |
|
|
, |
H |
, |
|
4 |
6 |
4 |
|
) |
NaOEt, |
) |
K |
Pd(PPh |
Pd(PPh |
||
|
|
|
, |
|
|
|
4 |
|
|
|
PO |
|
|
|
3 |
O
O |
|
3 |
3 |
CH |
|
|
CH |
3 |
|
CH |
CH |
|
3 |
|
Br |
|
TfO |
α,β,β-
[139]
78(>97)
, 4 ) 3 Pd(PPh THF
I
C 3 H
|
|
|
|
|
|
|
|
2 |
|
|
|
|
|
|
O |
B |
O |
O |
B |
O |
|
|
B(Sia) |
|
|
|
O |
|
|
|
O |
|
i |
|||||||||||
|
|
|
|
|
|
|
|
|
|
|
|
|
|
2 |
|
|
|
|
|
|
|
|
|
|
|
|
|
|
Bu |
|
|
|
|
|
|
|
|
|
OBn |
|
|
B |
Al |
|
|
|
CH |
|
|
|
|
O |
2 |
H |
|
|
H |
||
|
|
|
|
|
|
|
CH |
|
|
|||||
|
|
3 |
|
|
|
|
|
|
|
|
|
|
|
|
|
|
|
|
|
|
|
|
|
|
9 |
|
|
9 |
|
R |
|
|
|
|
|
|
O |
|
|
4 |
|
|
4 |
|
|
|
|
|
|
|
|
|
C |
|
|
C |
The entries are arranged according to the structural types of the alkenyl electrophiles summerized in Scheme 5. With the same category, they are arranged according to
a
arrangedaccordingtothepriority |
thetext. |
theentriesare |
asdiscussedin |
groupinthealkenylmetalreagents, |
thenthecountercationsarearranged |
thesubstituentsinthealkenylmetalsasintheprevioustables.Foragivenalkenyl |
orderofthesubstituentsinthealkenylelectrophiles.Finally,theleavinggroupsand |
381

382 |
III Pd-CATALYZED CROSS-COUPLING |
alkenyl–alkenyl coupling, but the reaction of alkenylmagnesium derivatives has been associated with various undesirable features including low chemoselectivity and low product yields. Although generally less reactive, alkenylmetals containing Al and Zr are attractive reagents, since they are often readily accessible via hydrometallation and carbometallation of alkynes and since highly satisfactory results have in fact been obtained in many cases. As is well known, various (E)- -substituted alkenylmetals are most generally and reliably obtainable by hydroboration of alkynes. This advantage, however, is offset to considerable extents by the low intrinsic reactivity of alkenylboranes in Pd-catalyzed cross-coupling. Despite the development of the Suzuki protocol for alkenyl–alkenyl coupling, the overall synthetic scope of B-based methods appears to be more limited than that of Zn-based methods. Another widely used metal for Pd-catalyzed alkenyl–alkenyl coupling is Sn. Its intrinsically low reactivity and toxicity are two main limitations associated with Sn. In many favorable cases, its reactions are often as favorable as those of other favorable metals mentioned above. In more demanding cases, however, Sn has often been shown to be significantly inferior to Zn, B, and some other metals,[209]–[211] as illustrated later. Another complication associated with Sn is the capricious and often difficult-to-predict courses of hydrostannation of alkynes.[26] On the other hand, Sn as well as B has been very useful in achieving intramolecular alkenyl–alkenyl coupling, which often requires the generation of cyclization precursors containing both a nucleophilic alkenylmetal moiety and an electrophilic alkenyl moiety.[212] Other less frequently used metals in Pd-catalyzed crosscoupling include Li, Cd, Si, and Cu; Si[25] and Cu[28] have provided some promising results. It is entirely possible that they may become widely used metal countercations in the future.
The following specific examples are presented to further support the above-mentioned generalizations.
The use of alkenylzincs as excellent organometallic partners in Pd-catalyzed alkenyl– alkenyl coupling has amply been demonstrated.[180],[192],[197],[213] In most cases, reactions proceed smoothly to produce the desired products in excellent yields and high stereoselectivities, as exemplified by the synthesis of methyl dimorphecolates shown in Scheme 46.[197]
Pent |
I |
|
|
(CH2)7CO2Me |
|
|
|
I |
|
|
OH |
|
|
|
|
|
3% Pd(PPh3)4 |
Pent |
|
|
|||
|
1. 2 t-BuLi |
|
|
||||
|
|
|
|
|
CO2Me |
||
|
2. ZnBr2 |
|
|
OH |
|
|
|
Pent |
I |
|
|
|
|
OH 94%, ~100% E,E |
|
|
|
(CH2)7CO2Me |
|
|
|||
|
ZnBr |
|
|
|
OH |
||
|
3% Pd(PPh3)4 |
|
|
||||
|
|
Pent |
|
CO2Me |
|||
|
|
|
|
|
|
||
|
|
|
|
|
|
88%, 99% E, Z
Scheme 46
Even though Sn and B may have been the two most frequently used metals in Pdcatalyzed alkenyl–alkenyl coupling over the past two decades, it has been demonstrated with increasing frequency that a few of the metals associated with Negishi coupling, especially Zn and Zr in some cases, are more advantageous, leading to decidedly more satisfactory results. For example, the Stille coupling between (E)-Bu3SnCHRCHSnBu3 and (E )- -bromomethylacrylate gives no more than 15% of the desired product even with the use of triphenylarsine as ligand of the Pd catalyst.[47] In sharp contrast, the corresponding coupling with (E )-Bu3SnCHRCHZnCl, generated in situ by stepwise transmetallations, proceeds rapidly to produce the desired product in 95% yield (Scheme 47).[209]

|
|
|
III.2.6 |
Pd-CATALYZED ALKENYL–ARYL |
383 |
||
|
|
|
CO2Me |
|
CO2Me |
|
|
|
SnBu3 |
|
Br |
Bu3Sn |
|
||
|
|
|
|
<15% |
|
||
Bu3Sn |
|
|
|
|
|
||
|
Pd2(dba)3, AsPh3 |
|
|
||||
|
1. n-BuLi |
|
THF, 50 °C |
|
|
|
|
|
2. ZnCl2 |
|
CO2Me |
|
|
|
|
|
|
|
|
|
|||
|
|
|
Br |
|
CO2Me |
|
|
|
ZnCl |
|
|
|
|
|
|
Bu3Sn |
|
|
|
Bu3Sn |
95% |
|
|
|
Pd(PPh3)4 |
|
|||||
|
|
|
|
|
|
||
|
|
|
0 °C, 15 min |
|
|
|
|
|
|
|
Scheme 47 |
|
|
|
The use of Zr in the hydrometallation–cross-coupling tandem process has been particularly attractive. Even in some highly demanding cases where polyconjugated enynes are used in cross-coupling reactions, the hydrozirconation–cross-coupling tandem reaction proceeds very smoothly to lead to satisfactory results as demonstrated in the synthesis of xerulin[223] (Scheme 48). Alkenylzirconium reagent, generated in situ by hydrogen transfer hydrozirconation of TBS-protected propargyl alcohol, is reacted with 1,1-dibromo alkene to stereoselectively produce trans-cross-coupled product[225] (Scheme 48).
TBS
Cp2ZrHCl
Br + ClCp2Zr TBS
|
5% Cl2Pd(PPh3)2 |
|
|
|
|
|
|
|
|
|
|
|
TBS |
95% |
|||
|
10% DIBAL |
|
|
|
|
|
|
|
|
|
|
|
|||||
|
|
|
|
|
|
|
|
|
|
|
|
||||||
|
|
|
|
|
|
|
|
|
|
|
|||||||
|
|
|
|
|
|
|
|
|
|
|
|
|
|
||||
|
|
|
|
|
|
|
|
|
|
|
|
|
|
|
|
|
|
|
[223] |
|
|
|
|
|
|
|
|
5% Cl2Pd(PPh3)2 |
|
|
|
||||
|
|
i-BuZrCp2Cl |
|
|
|
|
|
|
|
|
Br |
||||||
ZO |
|
ZO |
ZrCp2Cl |
10% DIBAL [225] |
ZO |
OZ |
|||||||||||
|
[224] |
|
|
||||||||||||||
|
|
|
|
||||||||||||||
|
|
|
|
|
|
|
|
|
Br |
|
|
|
|||||
|
|
|
|
|
90% |
|
|
Br |
OZ |
|
91%, >98% stereoselective |
||||||
|
|
|
|
|
|
|
|
|
|
|
Scheme 48
The superiority of alkenylzirconiums to other alkenylmetals has further been demonstrated in the synthesis of papulacandin D (Sect. III.2.18). The initial use of Stille coupling to synthesize the chain moiety resulted in less than 30% of desired product. However, the related coupling by using in situ generated alkenylzirconium gave the desired product in 82% yield[211] (Scheme 49).
|
|
|
|
OZ |
|
|
1. Cp2ZrHCl |
OTES |
O |
|
|
|
|||||||
|
|
|
|
|
|
|
2. A |
|
|
|
|
|
|
|
OMe |
||||
|
|
|
|
|
|
|
|
|
|
|
|
|
|||||||
|
|
|
|
|
|
|
3. Cl2Pd(PPh3)2 |
82% |
|
|
|
||||||||
|
|
|
|
|
|
|
|
|
|
|
|
|
|||||||
|
|
|
1. Cp2ZrHCl |
|
|
DIBAL |
|
|
|
|
|
|
|
|
|
||||
|
|
|
|
|
|
|
|
|
|
|
|
|
|
||||||
|
|
|
|
|
|
|
|
|
|
|
|
|
|
|
|
|
|
||
|
|
|
2. I2 |
OTBS |
|
|
|
|
|
|
|
OTBS |
|
|
|
O |
|||
|
|
|
|
|
|
|
|
|
|
|
|
|
|||||||
|
|
|
|
|
|
|
|
|
|
|
|
|
|
||||||
|
|
|
|
|
I |
Cl2Pd(CH3CN)2 |
30% |
|
|
|
|
|
OMe |
||||||
|
|
|
|
|
|
|
|
|
|
||||||||||
|
|
|
|
|
|
|
|
|
|
|
|
||||||||
|
|
|
|
|
|
|
|
(B) |
|
|
|
|
|
|
|
||||
|
|
|
|
|
|
|
|
|
|
|
|
|
|
|
|
|
|||
(E)-BrCH |
|
CHCO2Me (A) |
|
|
|
|
|
|
(E)-Bu3SnCH |
|
CHCO2Me |
(B) |
|||||||
|
|
|
|
|
|
|
|
||||||||||||
|
|
|
|
|
|
|
|
Scheme 49

384 |
III Pd-CATALYZED CROSS-COUPLING |
Although further comparisons of alkenylzirconiums to other alkenylmetals in Pdcatalyzed cross-coupling are desirable, the results discussed above clearly indicate that alkenylzirconiums are among the best alkenylmetals in alkenyl–alkenyl coupling, and the hydrozirconation–cross-coupling tandem process provides a convenient and satisfactory route to conjugated dienes and polyenes.
Although the intrinsic reactivity of alkenyl boranes in Pd-catalyzed cross-coupling is rather low, the use of a base remarkably enhances their reactivity as demonstrated by Suzuki and co-workers.[194]–[196],[200] Compared with other organometals containing Li, Mg, Zn, Al, Cu, and Zr, alkenylborons are very stable, even to alcohols and H2O. Thus, a wide variety of functional groups can be tolerated in their coupling reactions, as shown in Scheme 50.
B(OH)2 |
I |
|
|
|
|
|
Pd(PPh3)4 |
HO |
|
||||
HO(CH2)9 |
NaOEt, C6H6 |
66% |
|
|||
|
[200] |
|
|
|||
|
OTBS |
|
||||
|
|
|
|
|||
|
|
I |
|
HO2C(CH2)3 OTBS |
OTBS |
|
MeO2C(CH2)3 OTBS |
|
C5H11 |
||||
|
Pd(PPh3)4 |
|
||||
|
|
aq. LiOH, THF |
|
|
||
B(Sia)2 |
[214] |
85% |
C5H11 |
Scheme 50
However, the chemoselectivity in Pd-catalyzed cross-coupling of alkenylborons would suffer from the strongly basic condition and elevated reaction temperatures, when substrates with sensitive functional groups are employed. This limitation has been overcome, to some extent, by the use of aqueous solution of TlOH in place of NaOH or KOH as demonstrated by Kishi and co-workers in the synthesis of palytoxin.[215] The dramatic rate enhancement of Suzuki coupling by TlOH allows the reaction to proceed smoothly even at 0 °C. The utility of this method has been demonstrated in other natural product syntheses as well[216]–[220] (Sect. III.2.18).
As discussed earlier, the low intrinsic reactivity and high chemical stability of alkenyltins can be exploited in devising intramolecular alkenyl–alkenyl coupling[212] particularly in the synthesis of cyclic natural products (Sect III.2.18). Macrocarbocycles including cyclic ketones and macrolactones have been synthesized by this coupling reaction as shown in Scheme 51.
|
|
|
|
|
|
O |
|
|
Bu3SnCHBr |
|
|
|
|
|
|
|
O |
Pd2(dba)3 |
|
|
|
|
|
O |
|||||||||
|
|
|
|
|
|
|
|
|
|
|
LiI, CrCl2 |
|
|
|
|
|
|
|
|
|
AsPh3, NMP |
|
|
|
|
|
|
|
|||||
|
|
|
|
|
|
|
|
|
|
|
|
|
|
|
|
|
|
|
|
|
|
|
|
|
|||||||||
I |
|
|
O |
|
|
THF, DMF |
I |
|
|
|
|
|
|
70 °C, 18 h |
|
|
|
|
|
|
|
||||||||||||
|
|
|
|
|
|
|
|
|
|
|
|
|
|
|
|
|
|
|
|
|
|
|
|
||||||||||
|
|
|
|
|
|
|
|
|
60% |
|
|
|
|
|
|
|
Bu3Sn |
[221] |
|
|
|
|
|
96% |
|||||||||
|
|
|
|
|
|
|
|
|
|
|
|
|
|
|
|
|
|||||||||||||||||
|
|
|
|
|
|
|
H |
|
|
|
|
|
|
|
|
||||||||||||||||||
|
|
|
|
|
|
|
|
|
|
|
|
|
|
|
|
|
|
|
|
|
|
|
|
||||||||||
|
|
|
SnBu3 |
LDA, PhN(Tf)2 |
|
|
|
SnBu3 |
Pd(PPh3)4 |
|
|
|
|
|
|
|
|||||||||||||||||
|
|
|
|
|
|
|
|
|
|
|
|
|
|||||||||||||||||||||
|
|
|
|
|
|
|
|
|
HMPA, THF |
|
|
|
|
|
|
|
|
|
|
|
|
|
|
(CH2)n |
|||||||||
|
|
|
|
|
|
|
|
|
|
|
|
|
|
|
|
|
|
|
|
|
|
|
|||||||||||
|
|
|
|
|
|
|
|
|
|
|
|
|
|
|
|
|
|
LiCl, THF |
|
|
|
|
|
||||||||||
|
|
|
O |
−78 °C |
|
|
|
O |
TfO |
|
|
|
|
|
|
|
|||||||||||||||||
|
|
|
|
|
|
|
|
|
|
|
|
|
|||||||||||||||||||||
|
|
|
O |
|
|
|
|
|
|
|
|
[222] |
|
|
|
|
|
|
|
|
|
||||||||||||
O |
|
|
(CH2)n |
O |
|
|
|
(CH2)n |
|
|
O |
|
|
|
O |
||||||||||||||||||
|
|
|
|
|
|
56−57% |
|
|
|
||||||||||||||||||||||||
|
|
|
|
|
|
|
|
|
|
|
|
|
|
||||||||||||||||||||
n = 5, 6, 7, 8 |
|
|
|
|
|
|
|
|
|
|
|
|
|
|
|
|
|
|
|
|
|
||||||||||||
|
|
|
|
|
|
|
|
|
|
|
|
|
|
|
|
|
|
|
|
|
|
Scheme 51