
Principles and Applications of Asymmetric Synthesis
.pdf130a-ALKYLATION AND CATALYTIC ALKYLATION OF CARBONYL COMPOUNDS
38.Enders, D., Eichenauer, H. Angew. Chem. Int. Ed. Engl. 1979, 18, 397.
39.(a) Enders, D.; Hundertmark, T.; Lazny, R. Synlett 1998, 721. (b) Enders, D.; SchaÈfer, T.; Mies, W. Tetrahedron 1998, 54, 10232.
40.Related reviews and publications: (a) Enders, D.; Bhushan, V. Tetrahedron Lett. 1988, 29, 2437. (b) Enders, D.; Lohray, B. B. Angew. Chem. Int. Ed. Engl. 1987, 26,
351.(c) Synthesis of (R; R)-Statin: Enders, D.; Reinhold, U. Angew. Chem. Int. Ed. Engl. 1995, 34, 1219. (d) Synthesis of b-amino acids: Enders, D.; Wahl, H.; Bettary, W. Angew. Chem. Int. Ed. Engl. 1995, 34, 455.
41.Weber, T.; Edwards, J. P.; Denmark, S. E. Synlett 1989, 20.
42.Cornforth, J. W. Heterocycl. Compounds 1957, 5, 386.
43.Wehrmeister, H. L. J. Org. Chem. 1962, 27, 4418.
44.Allen, P.; Ginos, J. J. Org. Chem. 1963, 28, 2759.
45.Meyers, A. I.; Knaus, G.; Kamata, K. J. Am. Chem. Soc. 1974, 96, 268.
46.Meyers, A. I.; Knaus, G.; Kamata, K.; Ford, M. E. J. Am. Chem. Soc. 1976, 98,
47.Meyers, A. I.; Knaus, G. J. Am. Chem. Soc. 1974, 96, 6508.
48.Meyers, A. I.; Knaus, G.; Kendall, P. M. Tetrahedron Lett. 1974, 3495.
49.Meyers, A. I.; Knaus, G. Tetrahedron Lett. 1974, 1333.
50.Meyers, A. I.; Mihelich, E. D. J. Org. Chem. 1975, 40, 1186.
51.BystroÈm, S.; HoÈgberg, H.; Norin, T. Tetrahedron 1981, 37, 2249.
52.(a) Meyers, A. I.; Whitten, C. E. J. Am. Chem. Soc. 1975, 97, 6266. (b) Meyers, A. I.; Smith, R. K.; Whitten, C. E. J. Org. Chem. 1979, 44, 2250.
53.Oppolzer, W.; Moretti, R.; Thomi, S. Tetrahedron Lett. 1989, 30, 5603.
54.For reviews, see (a) Oppolzer, W.; Chapuis, S.; Bernardinelli, G. Helv. Chim. Acta 1984, 67, 1397. (b) Vandewalle, M.; Van der Eycken, J.; Oppolzer, W.; Vullioud, C.; Tetrahedron 1986, 42, 4035. (c) Davis, F. A; Towson, J. C.; Weismiller, M. C.; Lal, S.; Carroll, P. J. J. Am. Chem. Soc. 1988, 110, 8477.
55.Oppolzer, W.; Moretti, R.; Thomi, S. Tetrahedron Lett. 1989, 30, 6009.
56.Oppolzer, W.; Kingma, A. J. Helv. Chim. Acta 1989, 72, 1337.
57.Oppolzer, W. Pure Appl. Chem. 1990, 62, 1241.
58.Meyer, A. I.; Harre, M.; Garland, R. J. Am. Chem. Soc. 1984, 106, 1146.
59.Meyer, A. I.; Wanner, K. T. Tetrahedron Lett. 1985, 26, 2047.
60.Meyers, A. I.; Lefker, B. A. Tetrahedron Lett. 1987, 28, 1745.
61.(a) Romo, D.; Meyers, A. I. Tetrahedron 1991, 47, 9503. (b) Meyers, A. I.; Brengel, G. P. Chem. Commun. 1997, 1.
62.(a) Wender, P. A.; Keenan, R. M.; Lee, H. Y. J. Am. Chem. Soc. 1987, 109, 4390.
(b) Arlt, D.; Jautelat, M.; Lantzsch, R. Angew. Chem. Int. Ed. Engl. 1981, 20,
63.Meyers, A. I.; Romine, J. L.; Fleming, S. A. J. Am. Chem. Soc. 1988, 110, 7245.
64.Corey, E. J.; Chaykovsky, M. J. Am. Chem. Soc. 1965, 87, 1353.
65.Fuji, K.; Node, M.; Nagasawa, H.; Naniwa, Y.; Terada, S. J. Am. Chem. Soc. 1986, 108, 3855.
66.Rajappa, S. Tetrahedron 1981, 37, 1453.
2.11 REFERENCES 131
67.(a) Severin, T.; Pehr, H. Chem. Ber. 1979, 112, 3559. (b) Corey, E. J.; Estreicher,
H.J. Am. Chem. Soc. 1978, 100, 6294. (c) Miyashita, M.; Yanami, T.; Kumazawa, T.; Yoshikoshi, A. J. Am. Chem. Soc. 1984, 106, 2149.
68.Fuji, K.; Node, M.; Nagasawa, H.; Naniwa, Y.; Terada, S. J. Am. Chem. Soc. 1986, 108, 3855.
69.Fuji, K. Chem. Rev. 1993, 93, 2037.
70.Seebach, D.; Sting, A. R.; Ho¨mann, M. Angew. Chem. Int. Ed. Engl. 1996, 35, 2708.
71.Fuji, K.; Kawabata, T. Chem. Eur. J. 1998, 4, 373.
72.Matsushita, M.; Maeda, H.; Kodama, M. Tetrahedron Lett. 1998, 39, 3749.
73.Maruoka, K.; Ooi, T.; Yamamoto, H. J. Am. Chem. Soc. 1989, 111, 6431.
74.Shioiri, T.; Ninomiya, K.; Yamada, S. J. Am. Chem. Soc. 1972, 94, 6203.
75.SchoÈllkopf, U. Pure Appl. Chem. 1983, 55, 1799.
76.Seebach, D.; Aebi, J. D. Tetrahedron Lett. 1983, 24, 3311.
77.William, R. M. in Hasser, A. ed. Advances in Asymmetric Synthesis, vol. 1, JAI Press, London, 1995, pp 45±94.
78.Corey, E. J.; Guzman-Perez, A. Angew. Chem. Int. Ed. Engl. 1998, 37, 388.
79.(a) Chinchilla, R.; Galindo, N.; NaÂjera, C. Tetrahedron Asymmetry 1998, 9, 2769.
(b) Carloni, A.; Porzi, G.; Sandri, S. Tetrahedron Asymmetry 1998, 9, 2987.
80.Fitzi, R.; Seebach, D. Angew. Chem. Int. Ed. Engl. 1986, 25, 345.
81.Gilday, J. P.; Gallucci, J. C.; Paquette, L. A. J. Org. Chem. 1989, 54, 1399.
82.Larcheveque, M.; Ignatova, E.; Cuvigny, T. Tetrahedron Lett. 1978, 3961.
83.(a) Fuji, K.; Node, M.; Tanaka, F. Tetrahedron Lett. 1990, 31, 6553. (b) Fuji, K.; Node, M.; Tanaka, F.; Hosoi, S. Tetrahedron Lett. 1989, 30, 2825.
84.Evans, D. A.; Takacs, J. M. Tetrahedron Lett. 1980, 21, 4233.
85.(a) Evans, D. A.; Ennis, M. D.; Mathre, D. J. J. Am. Chem. Soc. 1982, 104, 1737.
(b) Evans, D. A.; Urpi, F.; Somers, T. C.; Clark, J. S.; Bilodeau, M. T. J. Am. Chem. Soc. 1990, 112, 8215.
86.(a) Meyers, A. I. Pure Appl. Chem. 1979, 51, 1255. (b) Meyers, A. I.; Knaus, G.; Kamata, K.; Ford, M. E. J. Am. Chem. Soc. 1976, 98, 567.
87.Ahn, K. H.; Lim, A.; Lee, S. Tetrahedron Asymmetry 1993, 4, 2435.
88.Oppolzer, W. Tetrahedron 1987, 43, 1969.
89.Jeong, K.; Parris, K.; Ballester, P.; Rebek, J. Angew. Chem. Int. Ed. Engl. 1990, 29, 555.
90.Negrete, G. R.; Konopelski, J. P. Tetrahedron Asymmetry 1991, 2, 105.
91.Fraser, R. R.; Akiyama, F.; Banville, J. Tetrahedron Lett. 1979, 3929.
92.Meyers, A. J.; Williams, D. R.; Erickson, G. W.; White, S.; Druelinger, M. J. Am. Chem. Soc. 1981, 103, 3081.
93.Saigo, K.; Kasahara, A.; Ogawa, S.; Nohira, H. Tetrahedron Lett. 1983, 24, 511.
94.Enders, D.; Gatzweiler, W.; Dederichs, E. Tetrahedron 1990, 46, 4757.
95.Enders, D.; Zamponi, A.; Raabe, G. Synlett 1992, 897.
96.For formation of acetals, see Ott, J.; Ramos Tombo, G. M.; Schimd, B.; Venanzi,
L.M.; Wang, G.; Ward, T. R. Tetrahedron Lett. 1989, 30, 6151.
132a-ALKYLATION AND CATALYTIC ALKYLATION OF CARBONYL COMPOUNDS
97.Mori, A.; Fujiwara, J.; Maruoka, K.; Yamamoto, H. Tetrahedron Lett. 1983, 24, 4581.
98.Solladie-Cavallo, A.; Su¨ert, J.; Gordon, M. Tetrahedron Lett. 1988, 29, 2955.
99.(a) Seebach, D.; Imwinkelried, R.; Stucky, G. Angew. Chem. Int. Ed. Engl. 1986, 25, 178. (b) Schreiber, S. L.; Reagan, J. Tetrahedron Lett. 1986, 27, 2945.
100.Harada, T.; Egusa, T.; Kinugasa, M.; Oku, A. Tetrahedron Lett. 1998, 39, 5531.
101.Harada, T.; Egusa, T.; Oku, A. Tetrahedron Lett. 1998, 39, 5535.
102.Alexakis, A.; Mangeney, P. Tetrahedron Asymmetry 1990, 1, 477.
103.Oguni, N.; Omi, T. Tetrahedron Lett. 1984, 25, 2823.
104.Berger, S.; Langer, F.; Lutz, C.; Knochel, P.; Mobley, T. A.; Reddy, C. K. Angew. Chem. Int. Ed. Engl. 1997, 36, 1496.
105.Seebach, D.; Beck, A. K. Chimia 1997, 51, 293.
106.(a) Zhang, F.; Yip, C.; Cao, R.; Chan, A. S. C. Tetrahedron Asymmetry 1997, 8, 585. (b) Mori, M.; Nakai, T. Tetrahedron Lett. 1997, 38, 6233.
107.Ito, K.; Kimura, Y.; Okamura, H.; Katsuki, T. Synlett 1992, 573.
108.RamoÂn, D. J.; Yus, M. Tetrahedron Asymmetry 1997, 8, 2479.
109.Soai, K.; Yokoyama, S.; Hayasaka, T. J. Org. Chem. 1991, 56, 4264.
110.Kitamura, M.; Suga, S.; Kawai, K.; Noyori, R. J. Am. Chem. Soc. 1986, 108, 6071.
111.Behnen, W.; Mehler, T.; Martens, J. Tetrahedron Asymmetry 1993, 4, 1413.
112.Soai, K. Watanabe, M. J. Chem. Soc. Chem. Commun. 1990, 43.
113.Soai, K.; Watanabe, M.; Koyano, M. J. Chem. Soc. Chem. Commun. 1989, 534.
114.Wallbaum, S.; Martens, J. Tetrahedron Asymmetry 1993, 4, 637.
115.Wally, H.; Widhalm, M.; Weissensteiner, W.; SchloÈgl, K. Tetrahedron Asymmetry 1993, 4, 285.
116.Kitamura, M.; Okata, S.; Suga, S.; Noyori, R. J. Am. Chem. Soc. 1989, 111, 4028.
117.Watanabe, M.; Araki, S.; Butsugan, Y. J. Org. Chem. 1991, 56, 2218.
118.Ho, D. E.; Betancort, J. M.; Woodmansee, D. H.; Larter, M. L.; Walsh, P. J.
Tetrahedron Lett. 1997, 38, 3867.
119.Pritchett, S.; Woodmansee, D. H.; Davis, T. J.; Walsh, P. J. Tetrahedron Lett. 1998, 39, 5941.
120.Ito, Y. N.; Ariza, X.; Beck, A. K.; BohaÂe, A.; Ganter, C.; Gawley, R. E.; KuÈhnle, F. N. M.; Tuleja, J.; Wang, Y. M.; Seebach, D. Helv. Chim. Acta 1994, 77, 2071.
121.Prasad, K. R. K.; Joshi, N. N. J. Org. Chem. 1997, 62, 3770.
122.Zhang, F.; Chan, A. S. C. Tetrahedron Asymmetry 1997, 8, 3651.
123.Cram, D. J.; Helgeson, R. C.; Peacock, S. C.; Kaplan, L. J.; Domeier, L. A.; Moreau, P.; Koga, K.; Mayer, J. M.; Chao, Y.; Siegel, M. G.; Ho¨man, D. H.; Sogah, G. D. Y. J. Org. Chem. 1978, 43, 1930.
124.Cotton, F. A.; Wilkinson, G. Advanced Inorganic Chemistry, 4th ed., Wiley, New York, 1980, 342.
125.Chan, A. S. C.; Zhang, F.; Yip, C. J. Am. Chem. Soc. 1997, 119, 4080.
126.Gibson, C. L. J. Chem. Soc. Chem. Commun. 1996, 645.
127.Kang, J.; Lee, J.; Kin, J. J. Chem. Soc. Chem. Commun. 1994, 2009.
128.Soai, K.; Suzuki, T.; Shono, T. J. Chem. Soc. Chem. Commun. 1994, 317.
2.11 REFERENCES 133
129.Wirth, T. Tetrahedron Lett. 1995, 36, 7849.
130.Jin, M.; Ahn, S.; Lee, K. Tetrahedron Lett. 1996, 37, 8767.
131.Williams, D. R.; Fromhold, M. G. Synlett 1997, 523.
132.Bringmann, G.; Breuning, M. Tetrahedron Asymmetry 1998, 9, 667.
133.Brunel, J.; Constantieux, T.; Legrand, O.; Buono, G. Tetrahedron Lett. 1998, 39, 2961.
134.Kossenjans, M.; Martens, J. Tetrahedron Asymmetry 1998, 9, 1409.
135.Watanabe, M.; Hashimoto, N.; Araki, S.; Butsugan, Y. J. Org. Chem. 1992, 57, 742.
136.Kimura, K.; Sugiyama, E.; Ishizuka, T.; Kunieka, T. Tetrahedron Lett. 1992, 33, 3147.
137.Cho, B. T.; Chun, Y. S. Tetrahedron Asymmetry 1998, 9, 1489.
138.(a) Tomioka, K. Synthesis 1990, 541. (b) Oguni, N.; Matsuda, Y.; Kaneko, T. J. Am. Chem. Soc. 1988, 110, 7877. (c) Kitamura, M.; Okada, S.; Suga, S.; Noyori, R. J. Am. Chem. Soc. 1989, 111, 4028. (d) Oppolzer, W.; Radinov, R. N. Tetrahedron Lett. 1988, 29, 5645. (e) Yoshioka, M.; Kawakita, T.; Ohno, M. Tetrahedron Lett. 1989, 30, 1657. (f ) Zhang, X.; Guo, C. Tetrahedron Lett. 1995, 36, 4947.
(g) Noyori, R.; Suga, S.; Kawai, K.; Okada, S.; Kitamura, M. Pure Appl. Chem. 1988, 60, 1597. (h) Smaardijk, A. A.; Wynberg, H. J. Org. Chem. 1987, 52, 135. (i) Corey, E. J.; Yuen, P. W.; Hannon, F. J.; Wierda, D. A. J. Org. Chem. 1990, 55, 784. ( j) Gi¨els, G.; Dreisbach, C.; Kragl, U.; Weigerding, M.; Waldmann, H.; Wandrey, C. Angew. Chem. Int. Ed. Engl. 1995, 34, 2005.
139.Dosa, P. I.; Fu, G. C. J. Am. Chem. Soc. 1998, 120, 445.
140.(a) RamoÂn, D. J.; Yus, M. Tetrahedron 1998, 54, 5651. (b) RamoÂn, D. J.; Yus, M.
Tetrahedron Lett. 1998, 39, 1239.
141.Nakamura, M.; Hirai, A.; Sogi, M.; Nakamura, E. J. Am. Chem. Soc. 1998, 120, 5846.
142.Reetz, M. T.; Kyung, S.; Bolm, C.; Zierke, T. Chem. Ind. 1986, 824.
143.(a) Narasaka, K.; Yamada, T.; Minamikawa, H. Chem. Lett. 1987, 2073. (b) Minamikawa, H.; Hayakawa, S.; Yamada, T.; Iwasawa, N.; Narasaka, K. Bull. Chem. Soc. Jpn. 1988, 61, 4379.
144.Hayashi, M.; Matsuda, T.; Oguni, N. J. Chem. Soc. Chem. Commun. 1990, 1364.
145.Mori, A.; Ohno, H.; Nitta, H.; Tanaka, K.; Inoue, S. Synlett 1991, 563.
146.(a) Hayashi, M.; Inoune, T.; Miyamoto, Y.; Oguni, N. Tetrahedron 1994, 50, 4385.
(b) Hayashi, M.; Miyamoto, Y.; Inoue, T.; Oguni, N. J. Org. Chem. 1993, 58, 1515. (c) Hayashi, M.; Tamura, M.; Oguchi, N. Synlett 1992, 663. (d) E¨enberger, F.; Heid, S. Tetrahedron Asymmetry 1995, 6, 2945. (e) Cainelli, G.; Giacomini, D.; TrereÂ, A.; Galletti, P. Tetrahedron Asymmetry 1995, 6, 1593. (f ) Klempier, N.; Pichler, U.; Griengl, H. Tetrahedron Asymmetry 1995, 6, 845. (g) Belokon, Y.; Ikonnikov, N.; Moscalenko, M.; North, M.; Orlova, S.; Tararov, V.; Yashkina, L.
Tetrahedron Asymmetry 1996, 7, 851. (h) North, M. Synlett 1993, 807.
147.Hayashi, M.; Miyamoto, Y.; Inoue, T.; Oguni, N. J. Chem. Soc. Chem. Commun. 1991, 1752.
148.Bolm, C.; MuÈller, P. Tetrahedron Lett. 1995, 36, 1625.
149.Mori, M.; Imma, H.; Nakai, T. Tetrahedron Lett. 1997, 38, 6229.
134 a-ALKYLATION AND CATALYTIC ALKYLATION OF CARBONYL COMPOUNDS
150.Sigman, M. S.; Jacobsen, E. N. J. Am. Chem. Soc. 1998, 120, 5315.
151.Belokon', Y. N.; Caveda-Cepas, S.; Green, B.; Ikonnikov, N. S.; Khrustalev, V. N.; Larichev, V. S.; Moscalenko, M. A.; North, M.; Orizu, C.; Tararov, V. I.; Tasinazzo, M.; Timofeeva, G. I.; Yashkina, L. V. J. Am. Chem. Soc. 1999, 121, 3968.
152.Sikorshi, J. A.; Miller, M. J.; Braccolino, D. S.; Cleary, D. G.; Corey, S. D.; Font, J. L.; Gruys, K. J.; Han, C. Y.; Lin, K. C.; Pansegrau, Ream, J. E.; Schnur, D.; Shah, A.; Walker, M. C. Phosphorous Sulfur Silicon 1993, 76, 115.
153.Stowasser, B.; Budt, K.; Li, J.; Peyman, A.; Ruppert, D. Tetrahedron Lett. 1992, 33, 6625.
154.Kametani, T.; Kigasawa, K.; Hiiragi, M.; Wakisaka, K.; Haga, S.; Sugi, H.; Tanigawa, K.; Suzuki, Y.; Fukawa, K.; Irino, O.; Saita, O.; Yamabe, S. Hetereocycles 1981, 16, 1205.
155.Meier, C.; Laux, W. H. G. Tetrahedron Asymmetry 1995, 6, 1089.
156.Pogatchnik, D. M.; Wiemer, D. F. Tetrahedron Lett. 1997, 38, 3495.
157.Davis, F. A.; Chen, B. C. Chem. Rev. 1992, 92, 919.
158.Rath, N. P.; Spilling, C. D. Tetrahedron Lett. 1994, 35, 227.
159.(a)Yokomatsu, T.; Yamagishi, T.; Shibuya, S. Tetrahedron Asymmetry 1993, 4, 1779. (b) Yokomatsu, T.; Yamagishi, T.; Shibuya, S. Tetrahedron Asymmetry 1993, 4, 1783.
160.Sasai, H.; Suzuki, T.; Arai, S.; Arai, T.; Shibasaki, M. J. Am. Chem. Soc. 1992, 114, 4418.
161.Sasai, H.; Bougauchi, M.; Arai, T.; Shibasaki, M. Tetrahedron Lett. 1997, 38, 2717.
162.Sasai, H.; Arai, T.; Satow, Y.; Houk, K. N.; Shibasaki, M. J. Am. Chem. Soc. 1995, 117, 6194.
163.Groaning, M. D.; Rowe, B. J.; Spilling, C. D. Tetrahedron Lett. 1998, 38, 5485.
164.Smith III, A. B.; Yager, K. M.; Taylor, C. M. J. Am. Chem. Soc. 1995, 117, 10879.

Principles and Applications of Asymmetric Synthesis
Guo-Qiang Lin, Yue-Ming Li, Albert S.C. Chan
Copyright ( 2001 John Wiley & Sons, Inc.
ISBNs: 0-471-40027-0 (Hardback); 0-471-22042-6 (Electronic)
CHAPTER 3
Aldol and Related Reactions
3.1INTRODUCTION
Chapter 2 provided a general introduction to the a-alkylation of carbonyl compounds, as well as the enantioselective nucleophilic addition on carbonyl compounds. Chiral auxiliary aided a-alkylation of a carbonyl group can provide high enantioselectivity for most substrates, and the hydrazone method can provide routes to a large variety of a-substituted carbonyl compounds. While a- alkylation of carbonyl compounds involves the reaction of an enolate, the well known aldol reaction also involves enolates.
Aldol reactions refer to the condensation of a nucleophilic enolate species with an electrophilic carbonyl moiety along with its analogs. These reactions are among those transformations that have greatly simpli®ed the construction of asymmetric C±C bonds and, thus, satis®ed the most stringent requirements for asymmetric organic synthesis methodology. Numerous examples of asymmetric aldol reactions can be found for syntheses of both complex molecules and small optically active building blocks.1
Acyclic stereocontrol has been a striking concern in modern organic chemistry, and a number of useful methods have been developed for stereoregulated synthesis of conformationally nonrigid complex molecules such as macrolide and polyether antibiotics. Special attention has therefore been paid to the aldol reaction because it constitutes one of the fundamental bond constructions in biosynthesis.
In the synthesis of complex natural products, one is frequently confronted with the task of creating intermediates possessing multiple contiguous stereogenic centers. The most e½cient synthetic strategies for such compounds are those in which the joining of two subunits results in the simultaneous creation of adjacent stereocenters. To have a better understanding of the aldol and related reactions, it is essential to be familiar with the foregoing strategies. When using them it is desirable to exert control over relative (syn/anti) as well as absolute (R/S) stereochemistry. Many studies have focused on the diastereoselective (enantioselective) aldol reactions. The major control variables in these asymmetric aldol reactions are the metal counterions, the ligands binding to these metals, and the reaction conditions. Several approaches are available for imposing asymmetric control in aldol reactions:
135

136ALDOL AND RELATED REACTIONS
1.Substrate control: This refers to the addition of an achiral enolate (or allyl metal reagent) to a chiral aldehyde (generally bearing a chiral center at the a-position). In this case, diastereoselectivity is determined by transition state preference according to Cram-Felkin-Ahn considerations.2
2.Reagent control: This involves the addition of a chiral enolate or allyl metal reagent to an achiral aldehyde. Chiral enolates are most commonly formed through the incorporation of chiral auxiliaries in the form of esters, acyl amides (oxazolines), imides (oxazolidinones) or boron enolates. Chiral allyl metal reagents are also typically joined with chiral ligands.
3.Double stereodi¨erentiation: This refers to the addition of a chiral enolate or allyl metal reagent to a chiral aldehyde. Enhanced stereoselectivity can be obtained when the aldehyde and reagent exhibit complementary facile preference (matched case). Conversely, diminished results might be observed when their facial preference is opposed (mismatched pair).
When chelated with proper chiral ligands, enolates of many metals (such as Li, Mg, Zr, B, Al, Sb, Si, and Ti) can a¨ord good stereoselectivity in asymmetric aldol reactions. Lithium and magnesium form chelates that can o¨er selectivity through Cram-Felkin-Ahn or chelation-controlled additions. The applications of titanium in particular are marvelous and diverse, and titanium enolates containing chiral ligands present an important area of enantioselective transformations. Similarly, boron enolates are widely used because of their high enantioselectivity. Heterobimetallic catalysts and/or two carbon-center catalysts activate both nucleophiles and electrophiles, thus being very good catalysts for asymmetric aldol reactions. These issues are further discussed in subsequent sections of this chapter.
It is only since the early 1980s that signi®cant progress has been made with aldol reactions. This chapter introduces some of the most important developments on the addition of metallic enolates and the more important of the related allylic metal derivatives to carbonyl compounds. These processes are depicted as paths A and B in Scheme 3±1.
Scheme 3±1
In general, the aldol reaction of an aldehyde with metal enolate creates two new chiral centers in the product molecule, and this may lead to four possible stereoisomers 2a, 2b, 2c, and 2d (Scheme 3±2 and Fig. 3±1).

3.1 INTRODUCTION 137
Scheme 3±2
Figure 3±1. Routes to the aldol products 2a±d.
Taking the boron-mediated aldol reaction as an example, one can conclude from the chair-like cyclic transition states 3a±d (Zimmerman-Traxler model3 as depicted in Fig. 3±1) that the enolate geometry can be translated into 2,3- stereochemistry in the product. One can see from Figure 3±1 that (Z)-enolate tends to give 2,3-syn-product, whereas (E )-enolate gives the 2,3-anti-one. The rationales of the high stereo-outcome are that the dialkylboron enolates have relatively short metal±oxygen bonds, and this is essential for maximizing 1,3- diaxial interactions in the transition states. The R1R2CH± moiety occupies a more stable transition state, a pseudo-equatorial position, which leads to aldol products in high stereoselectivity.
The following parameters are critically important for stereochemical control:
1.The size of the substituent moiety in the enolate
2.The proper choice of reagents
3.The conditions chosen for enolization
Accordingly, Liu et al.4 have designed two types of aldol reagents that can lead to opposite stereochemistry in aldol condensation reactions. In the following structures, compound 4 can be used for obtaining anti-aldol products, and compound 5 can be employed for synthesizing syn-aldol products (Scheme 3±3).
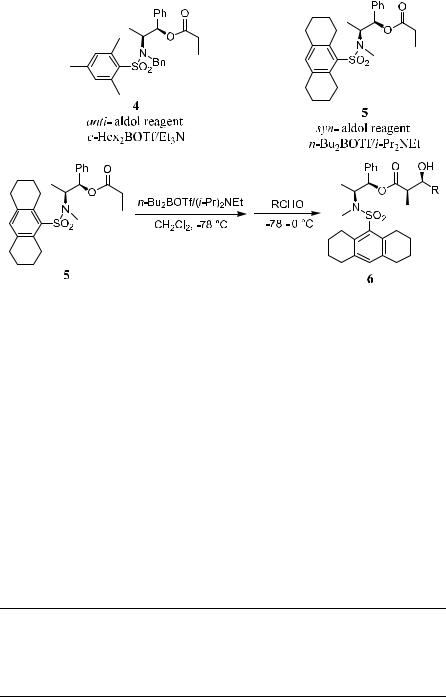
138 ALDOL AND RELATED REACTIONS
Scheme 3±3
When aldol reagent 5 is treated with aldehyde in the presence of n-Bu2BOTf and Et3N, syn-aldol product 6 can be produced with high diastereoselectivity (Table 3±1).
3.2SUBSTRATE-CONTROLLED ALDOL REACTION
3.2.1 Oxazolidones as Chiral Auxiliaries: Chiral Auxiliary-Mediated
Aldol-Type Reactions
In 1964, Mitsui et al.5 used a chiral auxiliary to achieve asymmetric aldol condensation, although the stereoselectivity was not high (58%) at that time. Signi®cant improvement came in the early 1980s when Evans et al.6 and Masamune et al.7 introduced a series of chiral auxiliaries that led to high stereo-
TABLE 3±1. Diastereoselective Aldol Reaction Using Chiral Reagent 5
Aldehyde |
syn:anti |
ds for syn |
Yield (%) |
|
|
|
|
EtCHO |
93:7 |
97:3 |
95 |
PrCHO |
94:6 |
>97:3 |
93 |
(E )-CH3CHbCHCHO |
93:7 |
>97:3 |
98 |
PhCHO |
94:6 |
95:5 |
97 |
ds ˆ diastereoselectivity.
Reprinted with permission by Pergamon-Elsevier Science Ltd., Ref. 4.
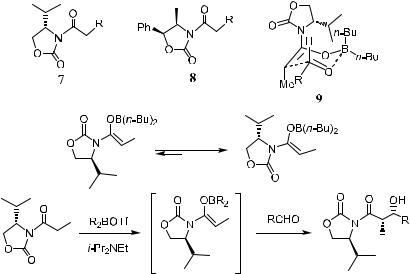
3.2 SUBSTRATE-CONTROLLED ALDOL REACTION |
139 |
selectivity. When bonded to dialkylboron enolates, these chiral auxiliaries induced aldol reactions with high selectivity.
The chiral boron enolates generated from N-acyl oxazolidones such as 7 and 8 (which were named Evans' auxiliaries and have been extensively used in the a-alkylation reactions discussed in Chapter 2) have proved to be among the most popular boron enolates due to the ease of their preparation, removal, and recycling and to their excellent stereoselectivity.8
Usually, (Z)-boron enolates can be prepared by treating N-acyl oxazolidones with di-n-butylboron tri¯ate and triethylamine in CH2Cl2 at ÿ78 C, and the enolate then prepared can easily undergo aldol reaction at this temperature to give a syn-aldol product with more than 99% diastereoselectivity (Scheme 3±4). In this example, the boron counterion plays an important role in the stereoselective aldol reaction. Triethylamine is more e¨ective than di-iso-propylethyl amine in the enolization step. Changing boron to lithium leads to a drop in stereoselectivity.
The stereoselectivity probably results from bidentate chelation of the metal (such as boron) with the oxazolidone carbonyl and the enolate oxygen via a chair-type transition state 9 (Scheme 3±4).1a,9
Scheme 3±4
When amide derivatives 10 and 12 are used in the reaction, a pair of enantiomers 11 and 13 (R ˆ CH3) can be obtained (Scheme 3±5).6
Double asymmetric induction (See section 1.5.3) can also be employed in aldol reactions. When chiral aldehyde 15 is treated with achiral boron-mediated enolate 14, a mixture of diastereomers is obtained in a ratio of 1.75:1. However, when the same aldehyde 15 is allowed to react with enolates derived from Evans' auxiliary 8, a syn-aldol product 16 is obtained with very high stereo-