
Navigation / Буклеты / NT_4000_Mathematical_Models_Technical_Description_eng
.pdf
Hydrodynamic Forces and Moments
lateral force
|
|
|
F (β ,ω ) |
(ω |
min |
≤ ω ≤ ω |
max |
), (−25o ≤ β ≤ 25o ) |
|
|
|
y |
|
|
|
||
Fy |
= |
~ |
(− ∞ ≤ ω < ω min , ω max < ω < ∞ ), |
|||||
|
Fy (β , 1 ω ) |
|||||||
|
|
|
|
|
|
(− 180o ≤ β < −25o ,25o < β < 180o ) |
||
hydrodynamic roll moment |
|
|
||||||
|
|
|
Mx (β ,ω ) |
(ω min ≤ ω ≤ ω max ), (−25o ≤ β ≤ 25o ) |
||||
|
|
|
||||||
Mx |
= |
|
~ |
(− ∞ ≤ ω < ω min , |
ω max < ω < ∞ ), |
|||
|
Mx (β , 1 ω ) |
|||||||
|
|
|
|
|
|
(− 180o ≤ β < −25o , 25o < β < 180o ) |
||
hydrodynamic yaw moment |
|
|
||||||
|
|
|
Mz (β ,ω ) |
(ω min ≤ ω ≤ ω max ), (−25o ≤ β ≤ 25o ) |
||||
|
|
|
||||||
Mz |
= |
|
~ |
(− ∞ ≤ ω < ω min ,ω max < ω < ∞ ), |
||||
|
Mz (β , 1 ω ) |
|||||||
|
|
|
|
|
|
(− 180o ≤ β < −25o , 25o < β < 180o ) |
Inertial forces, Force of Buoyancy and Forces of Static Stability
Inertial forces effecting the ship are the hull inertial forces, the centrifugal force and water inertial forces. The hull inertial forces are the forces proportional to the ship mass or ship inertia moment and ship acceleration. The centrifugal force is the force proportional to angular velocity square value and to the path curvature. The water inertial forces are the forces proportional to added masses and velocity square value.
The water inertial forces are considered as additional mass or additional inertia moments to ship hull mass or ship hull inertia moment. The added masses and added inertia moments using in the mathematical model of the ship moving in calm deep water are shown in Table 1.
The added masses numerical data are stored in the database.
Table 1.
λ11 |
- |
- |
- |
- |
- |
- |
λ22 |
- |
- |
- |
- |
- |
- |
λ33 |
- |
- |
- |
- |
- |
- |
λ44 |
- |
- |
- |
- |
- |
- |
λ55 |
- |
- |
- |
- |
- |
- |
λ66 |
Chapter 2. Ship Motion in Calm Deep Water. |
29 |

Hydrodynamic Forces and Moments
Force of buoyancy and restoring moments are calculated using the following formulas:
Vertical force:
gravity force |
Fz g |
= m g , |
force of buoyancy |
FzST |
= − ρg ( + AWL (zg − zg0 )) , |
damping force |
Fzz = −(ν 33 zg + Vν 33ψ + Vλ33ω y ) , |
|
roll moment: |
|
|
restoring roll moment |
Mx ST = − m g hθ (θ )θ , |
|
damping moment |
Mxx |
= −ν 44 (V )ω x , |
trim moment: |
|
|
restoring trim moment |
My ST = − m g hψ ψ , |
|
damping moment |
Myy |
= − (V 2ν 33ψ + ν 55ω y ) , |
where – the ship displacement, AWL – the waterplane area, zg − zg0 – the gravity center changing, hθ , hψ – the transverse and longitudinal metacentric heights, θ , ψ – roll and trim angles,V, ω x ,ω y – linear and angular velocity components.
Hull Hydrodynamic Characteristics
The forces and moments effecting the bare hull (ship hull without rudder and propeller) are called ship hull hydrodynamic characteristics. Hydrodynamic characteristics are obtained from the ship model tests results. Series of models areused for that. The following hull geometry parameters varied:
•Bow shape;
•Stern shape;
•Ship length/draft ratio L/T (L – length on waterline, Т – ship draft at midship);
•Ship breadth/draft ratio B/T (В – ship breadth at midship);
•Block coefficient CB ;
•Stern block coefficient A2 = A2 /(BT ) ( A2 is the 2nd theoretical frame area). Hydrodynamic characteristic values are defined as follows:
Longitudinal force
FxBH = CxBH (β ,ω )0.5ρVlq2LT ,
30 NAVI-TRAINER 4000. Mathematical Models. Technical Description.

Hydrodynamic Forces and Moments
Lateral force
FyBH = CyBH (β ,ω )0.5ρVlq2LT ,
Yaw hydrodynamic moment
Mz BH = CmzBH (β ,ω )0.5ρVlq2L2T ,
Roll hydrodynamic moment
Mx BH = Cmx BH (β ,ω ,θ )0.5ρVlq2L2T ,
where Cx BH , Cy BH , Cmz BH ,Cmx BH – non-dimensional longitudinal and lateral
components of the hydrodynamic force, non-dimensional yaw moment and nondimensional roll moment on bare hull; Vlq – ship speed relatively to water.
The vertical force and trimming moment values for the ships moving at Froude numbers Fn ≤ 0.3 are small when compared to the corresponding values of buoyancy force and forces of static stability. Therefore these values are not considered in the Part 1 of the technical description.
Non-dimensional ship hydrodynamic characteristics can be defined using the test results or from the database.
Formulas for non-dimensional hydrodynamic characteristics at calm deep water are shown below.
Non-dimensional Longitudinal Hydrodynamic Force CxBH (β ,ω )
Non-dimensional longitudinal hydrodynamic force is defined as the sum of two components: resistance coefficient of bare hull at straight courseCx0 (Fn,Rn) and
additional resistance due to the path curvature Cx BH .
Resistance coefficient is calculated as the sum of the residuary resistance and frictional resistance. The formula is as follows:
Cx0 =Cxr (Fn) + Cxf 0 (Rn) ,
where Cxr (Fn) – the residuary resistance, Cxf 0 (Rn) – the resistance of an
equivalent plate. The resistance coefficient numerical data are stored in the database.
Additional resistance Cx BH is defined as a function of path curvature and drift angle. Its structural formula is as follows:
CxBH = CxβBH β + CxωBHω
β |
|
|
|
|
|
2 |
ββ |
|
|
|
ω |
ω |
ω |
ω |
|||||||
Cx BH β ω |
|
+Cx BH |
where CxβBH , CxωBH , K ,
+CxβBHβ β 2+CxωBHω ω 2+ CxβBHω β ω + CxβBHβ ω β 2ω +
β2ω 2,
β β ω ω |
– the coefficients of the approximated function. |
Cx BH |
Chapter 2. Ship Motion in Calm Deep Water. |
31 |

Hydrodynamic Forces and Moments
Non-Dimensional Lateral Component of the Hydrodynamic Force
CyBH (β ,ω )
Non-dimensional lateral hydrodynamic force is obtained as a regression function. Its structural formula is as follows:
|
|
|
|
|
|
|
|
|
|
|
|
|
|
|
|
|
|
|
|
|
|
|
|
|
|
|
|
|
|
|
|
|
|
||||||
Cy BH = CyβBH β + Cyω |
BH |
ω |
+ CyβBHβ |
β 2+CyωBHω |
ω |
2+ CyβBHω β |
ω |
+ CyβBHβ ω β 2 |
ω |
+ |
|||||||||||||||||||||||||||||
β |
|
|
|
|
|
|
|
2 |
|
|
β β |
|
|
|
2 |
|
|
|
2 |
|
|
|
|
|
|
|
|
|
|
|
|
|
|
|
|||||
ω |
ω |
|
|
|
|
|
|
ω |
ω |
|
|
|
|
|
|
|
|
|
|
|
|
|
|
|
|||||||||||||||
Cy BH |
|
β ω |
|
+Cy BH |
β ω |
|
|
, |
|
|
|
|
|
|
|
|
|
|
|
|
|
|
|||||||||||||||||
β |
|
|
|
|
|
|
|
|
|
|
|
|
β β |
|
|
|
|
|
|
|
|
|
|
|
|
|
|
|
|
|
|
|
|
||||||
|
|
ω |
|
|
|
|
|
|
|
|
|
ω |
ω |
|
) – coefficients of the approximated function. |
||||||||||||||||||||||||
where ( Cy BH |
, Cy BH , K ,Cy BH |
|
Non-Dimensional Yaw Moment CmzBH (β ,ω )
Non-dimensional yaw moment is represented as a regression function. Its structural formula is as follows:
Cmz BH = Cmβ BH β + CmωBHω + Cmβ BHβ β 2+CmωBHω ω 2+ Cmβ ωBH β ω + Cmβ BHβ ω β 2ω +
β |
|
|
|
|
|
|
2 |
|
β β |
|
|
|
2 |
|
|
2 |
|
|
|
||
ω |
ω |
|
|
|
|
ω |
ω |
|
|
|
|||||||||||
Cm BH |
β ω |
|
|
+Cm BH |
β ω |
|
, |
|
|||||||||||||
where ( Cmzβ |
BH , C |
|
|
|
|
|
|
) – coefficients of the approximated function. |
|||||||||||||
mzω |
BH , K ,Cmzβ βBHω |
|
ω |
Non-Dimensional Roll Hydro-Dynamic Moment CmxBH (β ,ω ,θ )
Non-dimensional roll moment is also represented as a regression function. However, it does not depend only on drift angle β and path curvature ω, but also on roll angle θ. The structural formula of the roll hydrodynamic moment is as follows:
CmxBH (β ,θ , |
|
|
) = CmxBH 0 (β , |
|
)+ CmxBH (β ,θ , |
|
)θ = |
|
|
|
|
|
|
|
|
|
|
|
|
|
|
|
|
|
|
|
|
|
|
|
|
|
|
|
|
|
|
|
||||||||||||||||||||||||||||||||||||||||||||||||||||
ω |
ω |
ω |
|
|
|
|
|
|
|
|
|
|
|
|
|
|
|
|
|
|
|
|
|
|
|
|
|
|
|
|
|
|
|
|||||||||||||||||||||||||||||||||||||||||||||||||||||||||
[Cmx0 BH0 + Cmxβ |
|
|
|
|
|
|
|
|
|
|
|
|
|
|
|
|
|
|
|
|
|
|
|
|
|
|
|
|
|
|
|
|
|
|
|
|
|
|
|
|
|
|
|
|
|
|
|
|
|
|
|
|
|
|
|
|
|
|
|
|
||||||||||||||||||||||||||||||
BH0 β + Cmxω |
BH0 |
|
|
+ Cmxβ βBH0 β 2+ CmxBHω ω |
0 |
|
|
|
2+CmxBHβ ω |
0 β |
|
|
+ |
|||||||||||||||||||||||||||||||||||||||||||||||||||||||||||||||||||||||||||||
ω |
ω |
ω |
||||||||||||||||||||||||||||||||||||||||||||||||||||||||||||||||||||||||||||||||||||||||
β β |
|
|
|
|
|
|
|
2 |
|
|
|
|
|
|
|
|
β |
|
|
|
|
|
2 |
|
|
|
β β |
|
|
|
|
|
|
2 |
|
|
|
2 |
|
|
|
|
|
|
|
|
|
|
|
|
|
3 |
|
|
|
|
|
β |
|
|
|
|
|
|
|
|
|
|
|
|
|
|
|
3 |
|
|
||||||||||||||
ω |
|
|
|
|
|
|
|
ω |
ω |
|
|
|
ω |
ω |
|
|
|
|
|
ω |
ω |
ω |
|
|
|
ω |
ω |
ω |
|
|
|
|
|
|
|
|||||||||||||||||||||||||||||||||||||||||||||||||||||||
CmxBH 0 β |
|
ω + |
CmxBH 0 β ω |
|
+CmxBH 0 β |
|
|
ω |
|
+ Cmx BH0 |
ω |
|
|
+CmxBH 0 β ω |
|
|
+ |
|||||||||||||||||||||||||||||||||||||||||||||||||||||||||||||||||||||||||
β β |
|
|
|
|
|
|
|
2 |
|
|
|
3 |
|
|
|
|
|
|
β |
|
|
|
|
|
|
|
|
|
|
|
|
|
|
|
|
|
|
β β |
|
|
2 |
|
|
|
|
|
|
|
|
|
|
|
|
|
|
|
|
|
|
|
|
|
|
3 |
|
|||||||||||||||||||||||||
ω |
|
ω |
|
ω |
|
|
|
|
|
|
|
|
|
|
|
|
|
|
|
|
ω |
|
|
|
|
|
|
|
|
ω |
|
|
|
|
|
|
|
|
|
|
|
|
|
ω |
ω |
ω |
]θ , |
|||||||||||||||||||||||||||||||||||||||||||
Cmx BH0 β ω |
] + [dCmxBH |
β + dCmxBH |
ω + dCmxBH β |
|
|
ω + dCmxBH ω |
|
|
|
|||||||||||||||||||||||||||||||||||||||||||||||||||||||||||||||||||||||||||||||||
where CmxBHβ |
0, C |
mxω |
|
|
|
|
|
|
|
|
|
|
|
|
||||||||||||||||||||||||||||||||||||||||||||||||||||||||||||||||||||||||||||
BH0, K,Cmxβ βBHω |
ω0 , K,d CmxBHβ , K,d CmxBHω ω ω ) – coefficients of the |
|||||||||||||||||||||||||||||||||||||||||||||||||||||||||||||||||||||||||||||||||||||||||
approximated function. |
|
|
|
|
|
|
|
|
|
|
|
|
|
|
|
|
|
|
|
|
|
|
|
|
|
|
|
|
|
|
|
|
|
|
|
|
|
|
|
|
|
|
|
|
|
|
|
|
|
|
|
|
|
|
|
|
|
|
|
|
|
|
|
|
Let us remind that the above mentioned non-dimensional bare hull hydrodynamic characteristics can be used in the range of small values of drift angles and path curvature. Extrapolation of hydrodynamic characteristics onto the entire domain is done using special method. The method allows to obtain bare hull hydrodynamic characteristics at large values of drift angles and path curvature taking into account their values at small drift angles and path curvature, at large drift angles and straight
course ( 20o ≤ β ≤ 90o , ω = 0 ) and turning at ω = ∞ .
All coefficients’ numerical data are stored in the database.
32 NAVI-TRAINER 4000. Mathematical Models. Technical Description.
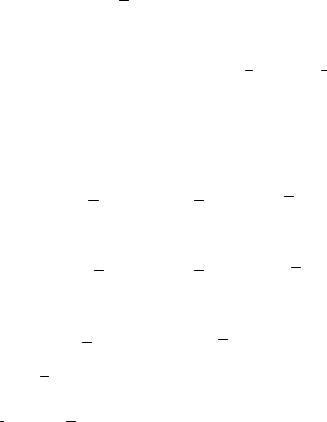
Hydrodynamic Forces and Moments
Steering Device Hydrodynamics
Rudders of different shapes are the most commonly used as the ship steering device. This section describes the rudders hydrodynamic characteristics. The steering devices that provide ship speed and steering simultaneously (e.g. vane propellers, nozzles, azimuth thrusters, waterjets etc.) are described in the ship propulsion device section.
Hydrodynamic force on rudder depends on the ship motion kinematic parameters, rudder geometry, its relative area, rudder angle, propeller operating conditions etc.
The angle between centreplane and rudder (rudder angle δ) defines the lateral force value on rudder. The rudder angle is positive at starboard rudder deflection.
Ship hull and propeller influence the hydrodynamic force on rudder. On the other hand, rudder and propeller effect the hydrodynamic characteristics of the bare hull. The additional values of force and moments due to interference of hull, propeller and rudder are called interaction force and moment. The ship mathematical model considers the interaction force and moment.
The database information allows to create mathematical models of the ships equipped with balanced and semibalanced rudders, Shilling’s rudders, Becker’s rudders, etc.
The following rudder geometry parameters are taken into account in mathematical model:
rudder type,
relative rudder area AR = AR / LT ( AR – rudder lateral area), rudder aspect ratio, λR = hR2 AR , ( hR – rudder height),
rudder position relatively to the propeller ( xR = xR / L , yR = yR / DP – rudder stock co-ordinates).
Lateral force on rudder as well as interaction forces and their moments at vertical and longitudinal axes are calculated. The formulas are as follows:
Lateral force
FyRIN = [CyR (β ,ω ,δ ,CT ) + CyIN (β ,ω ,δ )]0.5ρVlq2LTAR ,
yaw moment
MzRIN = [CyR (β ,ω ,δ )lR + CmIN (β ,ω ,δ )]0.5ρVlq2L2TAR ,
roll moment
Mx R = CyR (β ,ω ,δ ,CT ) ZR 0.5ρVlq2L2TAR ,
where AR = AR /(LT ) – relative rudder area, CyR – non-dimensional lateral force on rudder; CyIN , CmIN – non-dimensional interaction force and moment;
lR = lR / L , ZR = ZR /T – non-dimensional X-co-ordinate of the rudder stock and Z – co-ordinate of the lateral rudder force; δ – rudder angle, СТ – propeller thrust.
Chapter 2. Ship Motion in Calm Deep Water. |
33 |

Hydrodynamic Forces and Moments
Non-dimensional values of the lateral force and moment on rudder and interaction force and moment are calculated as regression function as follows:
0 |
|
|
|
|
β |
|
|
|
|
|
|
|
|
|
|
|
|
|
β β |
|
2 |
|
|
|
|
|
2 |
β |
|
|
|
|
|
|
|
β β |
|
|
|
2 |
|
|
|
||||||
|
|
ω |
|
|
|
|
|
β |
ω |
ω |
ω |
ω |
|
+ |
|||||||||||||||||||||||||||||||||||
Cy R = [Cy R0 + Cy R0 β |
+ Cy R0ω + Cy R0 |
+Cy R0ω |
|
+ Cy R0 β ω + Cy R0 |
|
|
β ω |
||||||||||||||||||||||||||||||||||||||||||
β |
|
|
|
|
|
|
2 |
|
β β |
|
|
|
2 |
|
2 |
δ |
|
|
|
|
|
|
|
|
|
δ |
|
|
|
|
|
δ |
|
|
|
|
|
|
2 |
|
|
|
|
||||||
ω |
ω |
|
|
|
|
ω |
ω |
|
|
|
|
|
|
|
|
|
ω |
|
|
|
|
ω |
ω |
|
|
|
|
|
|
|
|||||||||||||||||||
|
β ω |
|
|
|
|
|
|
|
|
|
|
|
|
|
|
|
ω |
)δ ] |
|
||||||||||||||||||||||||||||||
Cy R0 |
|
+Cy R0 |
β ω |
+(Cy R (λR ,xR ,yRP ) + Cy R |
ω + Cy R |
|
|
||||||||||||||||||||||||||||||||||||||||||
fy (CT ) f1( |
|
R ), |
|
|
|
|
|
|
|
|
|
|
|
|
|
|
|
|
|
|
|
|
|
|
|
|
|
|
|
|
|
|
|
|
|
|
|
|
|
|
|
|
|
||||||
A |
|
|
|
|
|
|
|
|
|
|
|
|
|
|
|
|
|
|
|
|
|
|
|
|
|
|
|
|
|
|
|
|
|
|
|
|
|
|
|
|
|
Cy IN = [Cy0IN + CyβIN β + CyωIN ω + CyβINβ β 2+CyωINω ω 2+ CyβINω β ω + CyβINβ ω β 2ω +
C β |
|
|
|
β |
|
2 |
+C β β |
|
|
|
β 2 |
|
2 |
+(Cδ |
+ Cδ |
|
|
|
+ Cδ |
|
|
|
|
|
|
|
2)δ ]f ( |
|
). |
|||
ω |
|
ω |
ω |
ω |
|
ω |
ω |
ω |
|
ω |
ω |
|
ω |
|
ω |
A |
||||||||||||||||
y IN |
y IN |
y IN |
|
y IN |
|
|
y IN |
|
|
|
|
|
1 R |
|||||||||||||||||||
|
|
|
|
|
|
|
|
|
|
|
|
|
|
β |
|
|
|
|
|
β β |
|
|
|
|
|
|
||||||
|
|
|
|
|
|
|
|
|
|
|
|
|
|
|
ω |
|
|
|
ω |
ω |
), |
|
|
|||||||||
In the formulas mentioned above (Cy R0 |
, Cy R0 |
, K ,Cy R0 |
|
|
|
|
|
|
|
(CyβIN , CyωIN , K ,CyβINβ ω ω ) are the approximating function coefficients. They depend on ship hull size, shape and rudder shape and size, f (CT ) is the function that obtain
the propeller influence on the rudder force and the interaction force, f1(AR ) is the coefficient that describes the relative rudder area value.
Non-dimensional yaw moment
Cmz IN = [Cm0 IN + Cmβ IN β + CmωINω + CmβINβ β 2+CmωINω ω 2+ Cmβ ωIN β ω + Cmβ INβ ω β 2ω +
C β |
|
|
|
β |
|
2+C β β |
|
|
|
β 2 |
|
|
|
2 |
+(Cδ |
+ Cδ |
|
|
|
+ Cδ |
|
|
|
|
|
2)δ ]f |
|
( |
|
), |
|
ω |
|
ω |
ω |
ω |
|
ω |
ω |
ω |
|
ω |
ω |
|
ω |
ω |
2 |
A |
|||||||||||||||
mIN |
|
|
|
mIN |
mIN |
mIN |
|
mIN |
|
R |
|||||||||||||||||||||
β |
|
|
ω |
|
|
|
β β |
|
|
|
|
|
|
|
|
|
|
|
|
|
|
|
|
|
|
|
|
||||
|
|
|
|
|
ω |
|
ω |
|
) are the approximating function coefficients. They |
||||||||||||||||||||||
where ( Cmz IN , Cmz IN , K,Cmz IN |
depends on ship hull sizes and shape and rudder form and size, f2(AR ) is the coefficient that describes the relative rudder area value influence.
To describe the performance of steering gear, the rudder hydrodynamic moment about rudder stock is calculated.
In addition to the values of the of the lateral force at rudder that are calculated relatively to the ship gravity center coordinates, hydrodynamic moment at rudder stock is also calculated, which is necessary for engine modelling. The calculation formula is as follows:
Mbal R = Cm bal R (β ,ω ,δ ,CT )0.5ρVlq2 AR bcp ,
where Cm bal R (β ,ω ,δ ,CT ) – non-dimensional hydrodynamic moment about rudder stock, bcp – the rudder chord.
The approximate function coefficients for hydrodynamic characteristics calculation are stored in the database.
Rudder deflection δ = δ (t ) is done by the steering gear in according to the specified control action.
34 NAVI-TRAINER 4000. Mathematical Models. Technical Description.

Hydrodynamic Forces and Moments
Propeller Hydrodynamic Characteristics
Propellers
Propeller is one of the propulsion devices in common use. In mathematical model, two propeller types are be used: fixed pitch propeller (FPP) and controllable pitch propeller (CPP).
When developing the propeller mathematical model, the following geometric parameters are considered:
•propeller type,
•propeller diameter, DP ,
• pitch (for FPP) or structural pitch (for CPP), PP / DP ,
•blade-area ratio, AP / AP0 ,
•blades number, ZP ,
• position relatively to the hull, xP = xP / L , yP = yP / B ,
•shaft inclination.
The force developed by propeller in the shaft direction (propeller thrust) as well as its lateral force and moment, are defined for the propeller operating in the free water, and then are adjusted according to the flow angle at propeller position, hull and appendage shape. The values of the forces and the moment are calculated according to the formulas:
propeller thrust
Fx P = ρ n2 DP 4 (1− t) KT (J,PP / DP ) ; lateral force on propeller
Fy P = ρ n2 DP 4 Ky (J,PP / DP ,n) ; shaft torque
Qp = ρ n2 DP 5 KQ (J,PP / DP ) ;
where n – propeller rotation frequency (RPM), DP – the propeller diameter, t – the trust reduction fraction (obtained the influence of hull and appendage shape),
J = |
Vp (1− Wp ) |
– advance propeller coefficient, Vp |
– axial velocity, Wp - wake |
|
n DP |
||||
|
|
|
scaling factor, PP/DP – pitch ratio.
Propeller load is calculated by formula:
|
Fx P |
||
CT = |
|
|
. |
0.25π D 2 |
0.5ρV 2 |
||
|
P |
|
|
At circulation, the lateral force on hull due to hull propeller interaction is considered when hull hydrodynamic characteristics are obtained (interaction force, see
Steering Device Hydrodynamics, page 33).
Lateral force due to propeller influence on ship hull when she goes astern is considered as a function of propeller pitch and propeller rotation frequency.
Chapter 2. Ship Motion in Calm Deep Water. |
35 |

Hydrodynamic Forces and Moments
As it is well-known, the propeller thrust vector is directed perpendicularly to the propeller disk plane. In case of shaft axis being imparallel to the centreplane or to the base plane, thrust components are calculated as the thrust projection on the body axis.
For two or more propellers, each propeller thrust is calculated, and the total thrust and moment are calculated as the sum of corresponding values.
If necessary, propeller thrust calculations may be obtained with consideration of the cavitation process.
The duct influence on the thrust value of ducted propeller is taken into account by special correction of propeller thrust.
Steering Ducted Propellers
The fixed pitch propeller or controllable pitch propeller can be used as the steering ducted propeller. The following propeller geometric parameters are considered in mathematical model:
•minimal internal duct diameter, DN ,
•relative duct length, lN / DN , (lN – duct length),
•position near to hull, xN = xN / L , yN = yN / B ( xN , yN – duct stock coordinates),
•shaft axis inclination.
The model allows to calculate forces and moments developed by the steering ducted propeller. The following formulas are used:
longitudinal force component
F |
x PN |
= C |
x PN |
(δ ,β ,C )(0.5 ρV 2 |
πD |
l |
N |
+ |
|
|
|
F |
xP |
|
|
) , |
|
|
||||||||||
|
|
|
|
|||||||||||||||||||||||||
|
|
|
|
T |
lq |
|
|
N |
|
|
|
|
|
|
|
|
|
|
|
|
|
|
||||||
lateral force component |
|
|
|
|
|
|
|
|
|
|
|
|
|
|
|
|
|
|
|
|
||||||||
F |
|
|
= C |
y PN |
(δ , β ,C )(0.5 ρV 2 |
πD l |
N |
+ |
|
F |
|
) , |
|
|
||||||||||||||
|
|
|
|
|
|
|||||||||||||||||||||||
y PN |
|
|
|
T |
lq |
|
N |
|
|
|
|
|
xP |
|
|
|
|
|
|
|||||||||
yaw moment |
|
|
|
|
|
|
|
|
|
|
|
|
|
|
|
|
|
|
|
|
|
|
|
|||||
M |
z PN |
= C |
mz PN |
(δ , β ,C )(0.5 ρV 2 |
πD l |
N |
+ |
|
F |
|
|
|
) l |
N |
, |
|||||||||||||
|
|
|
|
|||||||||||||||||||||||||
|
|
|
|
|
T |
|
lq |
|
N |
|
|
|
|
|
|
xP |
|
|
||||||||||
where Cx PN |
Cy PN |
Cmz PN |
– non-dimensional components of the longitudinal and |
lateral forces and the yaw moment of steering ducted propeller, DN , lN – the duct diameter and length, FxP is the propeller thrust.
Non-dimensional force components on the duct and non-dimensional moment are defined by formulas:
non-dimensional longitudinal force
C |
x PN |
= C0 |
(β ,C ) + Cδ |
(β ,C ) |
|
δ |
|
+ Cδδ |
(β ,C )δ 2 |
, |
|
|
|
||||||||||
|
x PN |
T |
x PN |
T |
|
|
|
x PN |
T |
|
36 NAVI-TRAINER 4000. Mathematical Models. Technical Description.

Hydrodynamic Forces and Moments
non-dimensional lateral force
Cy PN = Cy0 PN (β ,CT )+ Cyδ PN (β ,CT )δ ,
non-dimensional yaw moment
CmzPN = Cmz0 PN (β ,CT )+ Cmzδ PN (β ,CT )δ
where Cx0PN , Cxδ PN , CxδδPN – the coefficients depending on the drift angle and the propeller load coefficient.
The duct deflection is usually fulfilled by the steering engine. Duct deflection angle δ, propeller pitch and propeller rotation frequency are the steering values.
Waterjet
The mathematical model allows to simulate the water jet performance with steering and reversing gear. The following propeller geometric parameters can be considered in the mathematical model:
•impeller diameter, dWJ ,
•waterjet output nozzle diameter, DWJ ,
•waterjet height, hWJ ,
• position, xWJ = xWJ / L , yWJ = yWJ / B ,
•rudder device sizes and type
Waterjet thrust is defined depending on the impeller rotation frequency and ship velocity. The force developed by the waterjet in its axis direction is calculated using formula:
~ |
|
D2 |
2 |
FWJ = 0.5 |
ρπ |
4 |
VWJ CT (nWJ ,dWJ ) , |
where D – the output jet diameter, VWJ – the flow velocity at output section, CT – the impeller load coefficient, nWJ – the impeller rotation frequency, dWJ – the impeller diameter.
The flow velocity at waterjet output section is calculated using the formula:
VWJ = Vx lq (1− WWJ )
where WWJ – the water jet scaling factor.
The impeller load coefficient depends on the flow velocity at waterjet input section and its throttling-pressure characteristic KH = f (Kq) ( KH – pressure coefficient, Kq
– throttling coefficient).
Forces and moments components in body axis are calculated using formulas:
longitudinal force
= ~ δ
Fx WJ FWJ cos k WJ ,
Chapter 2. Ship Motion in Calm Deep Water. |
37 |
Hydrodynamic Forces and Moments
lateral force
= ~ δ
Fy WJ FWJ sin k WJ ,
where δWJ – the rudder device angle, k – the coefficient depends on the rudder device geometry.
Roll and trim moments as well as the yaw moment due to waterjet operation are calculated considering the water jet position on the ship.
Waterjet propeller control effects impeller rotation frequency, vanes position and blades’ angle of the steering and reversing gear.
Azimuth Thrusters
The mathematical model allows to simulate azimuth thruster operation (including Z- Drive). Fixed pitch propeller or controllable pitch propeller can be used.
Size and position of devise are taken into account.
Lateral and longitudinal forces, roll, trim and yaw moments are calculated. Force and moment components in body axis are calculated using formulas:
longitudinal force
Fx AP |
′ |
′ |
= FxAP cos(δ AP ) + FyAP sin(δ AP ) , |
||
lateral force |
|
|
Fy AP |
′ |
′ |
= −FxAP sin(δ AP ) + FyAP cos(δ AP ) , |
roll moment
Mx AP = FyAP zAP
trim moment
My AP = FxAP zAP
yaw moment
Mz AP = −FxAP y AP + Fy AP xAP |
|
|
|
||
where δ |
AP |
– the azimuth thrusters deflection angle, F′ |
and F′ |
are the |
|
|
|
xAP |
yAP |
|
|
projections of forces in the body axis, |
xAP , y AP , zAP – the distance from the |
propeller disk and propeller center co-ordinates to the center of gravity.
F′ |
and F′ |
are defined as functions of the azimuth thrusters deflection angle |
xAP |
yAP |
|
taking into account the value of local drift angle. The formulas are:
F′ |
= R |
AP |
cos(γ |
AP |
) |
|
||
xAP |
|
|
|
|
|
|||
F′ |
= R |
AP |
sin(γ |
AP |
) , |
|
||
yAP |
|
|
|
|
|
|||
where RAP |
– the propeller thrust, γ AP (α AP,C |
) – the angle between the force |
||||||
|
|
|
|
|
|
|
T |
direction and propeller axis (α AP = δ AP − β AP , β AP – the local drift angle).
38 NAVI-TRAINER 4000. Mathematical Models. Technical Description.