
Учебное пособие 1988
.pdfIssue № 1 (49), 2021 |
ISSN 2542-0526 |
2.4 %, not to mention the sum of the squares of the deviations, which is many times less than when calculated by means of the norms method.
Secondly, for centrifuged and vibrocentrifuged columns, the diagrammatic approach in any suggested implementation method yields better results when using the differential characteristics of concrete than the integral characteristics of concrete.
There are two important points, though. Firstly, all the suggested methods, even the simplified one, showed considerably better convergence with the experimental data than the calculation by means of the method of norms. Note that all the same noted features of the deviation of the calculated values from the experimental results were also typical of the deformations of the columns as well as of their load-bearing capacities.
I.e., considering the greater differentiation of concrete characteristics in the calculations leads oftentimes very considerably more accurate calculation for both groups of limiting states. The suggested analytical method for the strength of short centrally compressed reinforced concrete columns based on the deformation diagram of materials in both iterative and approximate and simplified formulations satisfactorily estimates the bearing capacity of vibrated, centrifuged and vibrocentrifuged columns.
Таble 1
Deviations in the values of the bearing capacity of the columns obtained by means of different calculation methods from the experimental value
|
|
|
Deviations of the design values from the experimental ones, % |
|
||||||||||
|
|
|
|
|
|
|
|
|
|
|
|
|
|
|
|
|
|
|
|
Using the diagram approach, to the |
|
|
|
|
|||||
|
|
|
|
|
|
|
|
|
|
|
|
|||
Column |
Iteration way, according to the |
|
Approximate method, accor- |
|
Simplified method, according |
|||||||||
code |
characteristics of concrete |
|
ding to the characteristics |
|
to the characteristics |
|||||||||
|
|
of concrete |
|
|
|
of concrete |
|
|||||||
|
|
|
|
|
|
|
|
|
|
|
||||
|
|
|
|
|
|
|
|
|
|
|
|
|
|
|
|
|
stan- |
integral |
differen- |
|
stan- |
integral |
|
differen- |
|
stan- |
integral |
|
differen- |
|
|
dard |
tial |
|
dard |
|
tial |
|
dard |
|
tial |
|||
|
|
|
|
|
|
|
|
|
|
|
|
|
|
|
В0 |
|
11.3 |
10.2 |
–– |
|
18.2 |
13.3 |
|
–– |
|
20.3 |
19.2 |
|
–– |
|
|
|
|
|
|
|
|
|
|
|
|
|
|
|
В4 |
|
10.8 |
9.9 |
–– |
|
17.7 |
11.9 |
|
–– |
|
19.7 |
18.8 |
|
–– |
|
|
|
|
|
|
|
|
|
|
|
|
|
|
|
В6 |
|
10.1 |
9.6 |
–– |
|
17.0 |
11.6 |
|
–– |
|
19.0 |
18.0 |
|
–– |
|
|
|
|
|
|
|
|
|
|
|
|
|
|
|
Ц0 |
|
9.4 |
8.4 |
5.6 |
|
16.6 |
10.3 |
|
7.8 |
|
18.6 |
17.7 |
|
7.7 |
|
|
|
|
|
|
|
|
|
|
|
|
|
|
|
Ц4 |
|
9.1 |
8.2 |
4.4 |
|
16.3 |
10.2 |
|
7.2 |
|
18.0 |
16.9 |
|
7.2 |
|
|
|
|
|
|
|
|
|
|
|
|
|
|
|
Ц6 |
|
8.8 |
7.6 |
4.1 |
|
16.1 |
10.0 |
|
6.0 |
|
17.4 |
16.5 |
|
6.1 |
|
|
|
|
|
|
|
|
|
|
|
|
|
|
|
ВЦ0 |
9.2 |
7.2 |
3.8 |
|
13.8 |
9.8 |
|
5.7 |
|
16.8 |
12.7 |
|
6.8 |
|
|
|
|
|
|
|
|
|
|
|
|
|
|
|
|
ВЦ4 |
8.3 |
7.1 |
3.0 |
|
12.5 |
8.3 |
|
4.5 |
|
16.2 |
11.4 |
|
5.8 |
|
|
|
|
|
|
|
|
|
|
|
|
|
|
|
|
ВЦ6 |
7.6 |
6.5 |
2.4 |
|
12.2 |
7.8 |
|
4.1 |
|
15.6 |
11.2 |
|
5.2 |
|
|
|
|
|
|
|
|
|
|
|
|
|
|
|
|
∑ |
2 |
806.2 |
634.3 |
96.7 |
|
2229.5 |
989.0 |
|
218.2 |
|
2921.9 |
2333.9 |
|
255.3 |
|
|
|
|
|
|
|
|
|
|
|
|
|
|
|
41

Russian Journal of Building Construction and Architecture
Таble 2
Deviations in column deformability values,
obtained by means of calculation methods from the experimental value
|
|
|
Deviations of the design values from the experimental ones, % |
|
||||||||||
|
|
|
|
|
|
|
|
|
|
|
|
|
|
|
|
|
|
|
|
Using the diagram approach, to the |
|
|
|
|
|||||
|
|
|
|
|
|
|
|
|
|
|
|
|||
Column |
Iteration way, according to the |
|
Approximate method, accor- |
|
Simplified method, according |
|||||||||
code |
characteristics of concrete |
|
ding to the characteristics |
|
to the characteristics |
|||||||||
|
|
of concrete |
|
|
|
of concrete |
|
|||||||
|
|
|
|
|
|
|
|
|
|
|
||||
|
|
|
|
|
|
|
|
|
|
|
|
|
|
|
|
|
stan- |
integral |
differen- |
|
stan- |
integral |
|
differen- |
|
stan- |
integral |
|
differen- |
|
|
dard |
tial |
|
dard |
|
tial |
|
dard |
|
tial |
|||
|
|
|
|
|
|
|
|
|
|
|
|
|
|
|
В0 |
|
10.2 |
9.7 |
–– |
|
18.0 |
12.8 |
|
–– |
|
18.9 |
16.2 |
|
–– |
|
|
|
|
|
|
|
|
|
|
|
|
|
|
|
В4 |
|
9.8 |
9.5 |
–– |
|
17.7 |
12.4 |
|
–– |
|
17.7 |
15.9 |
|
–– |
|
|
|
|
|
|
|
|
|
|
|
|
|
|
|
В6 |
|
9.3 |
9.1 |
–– |
|
17.5 |
12.0 |
|
–– |
|
17.0 |
15.5 |
|
–– |
|
|
|
|
|
|
|
|
|
|
|
|
|
|
|
Ц0 |
|
8.4 |
8.4 |
5.0 |
|
15.2 |
11.2 |
|
6.2 |
|
17.6 |
16.7 |
|
4.7 |
|
|
|
|
|
|
|
|
|
|
|
|
|
|
|
Ц4 |
|
8.2 |
8.0 |
4.1 |
|
15.1 |
10.6 |
|
5.8 |
|
17.0 |
16.4 |
|
4.2 |
|
|
|
|
|
|
|
|
|
|
|
|
|
|
|
Ц6 |
|
7.9 |
7.8 |
3.7 |
|
14.1 |
10.1 |
|
5.0 |
|
17.1 |
16.3 |
|
4.0 |
|
|
|
|
|
|
|
|
|
|
|
|
|
|
|
ВЦ0 |
8.4 |
7.1 |
3.2 |
|
13.6 |
9.6 |
|
4.7 |
|
17.1 |
14.5 |
|
3.8 |
|
|
|
|
|
|
|
|
|
|
|
|
|
|
|
|
ВЦ4 |
8.2 |
7.0 |
3.0 |
|
13.5 |
8.8 |
|
4.6 |
|
16.6 |
13.4 |
|
3.5 |
|
|
|
|
|
|
|
|
|
|
|
|
|
|
|
|
ВЦ6 |
7.4 |
6.8 |
2.5 |
|
12.7 |
7.4 |
|
4.3 |
|
15.6 |
13.2 |
|
3.2 |
|
|
|
|
|
|
|
|
|
|
|
|
|
|
|
|
∑ |
2 |
679.3 |
608.2 |
81.0 |
|
2129.9 |
1025.8 |
|
158.8 |
|
2662.0 |
2133.1 |
|
92.7 |
|
|
|
|
|
|
|
|
|
|
|
|
|
|
|
Conclusions. Within the framework of the diagrammatic approach, iterative, approximate and simplified methods of calculating the load-bearing capacity of reinforced concrete vibrated, centrifuged and vibrocentrifuged columns are set forth.
Calculation using the diagrammatic approach in all settings –– iterative, approximate and simplified methods –– showed significantly better convergence with experimental data than calculation according to the method of norms.
For centrifuged and vibrocentrifuged columns, the diagrammatic approach in any setting, i.e., iterative, approximate and simplified methods, i.e., yields better results when using the differential characteristics of concrete than the integral and, moreover, the standard characteristics of concrete.
References
1. Aksomitas G. A. Prochnost' korotkikh tsentrifugirovannykh kolonn kol'tsevogo secheniya s prodol'noi armaturoi klassa At-V pri kratkovremennom szhatii. Diss. kand. tekhn. nauk [Strength of short ring-section centrifuged columns with longitudinal reinforcement of class At-V under short-term compression. Dr. eng. sci. diss.]. Vil'nyus, 1984. 261 p.
42
Issue № 1 (49), 2021 |
ISSN 2542-0526 |
2.Gushcha Yu. P., Lemysh L. L. [On the issue of improving the calculation of deformations of reinforced concrete elements]. Napryazhenno-deformirovannoe sostoyanie betonnykh i zhelezobetonnykh konstruktsii: sb. statei [Stress-strain state of concrete and reinforced concrete structures: collection of articles]. Moscow, Stroiizdat Publ., 1986. P. 26––39.
3.Dmitriev S. A. [Refinement of the strength calculation of conventional and prestressed elements of the annular section]. Issledovanie prochnosti, zhestkosti i treshchinostoikosti zhelezobetonnykh konstruktsii. Vyp. 26 [Research of strength, rigidity and crack resistance of reinforced concrete structures. Iss. 26]. Moscow, Stroiizdat Publ., 1962. P. 5––20.
4.Ivashchenko E. I. Razrabotka metodov rascheta zhelezobetonnykh elementov na osnove deistvitel'nykh diagramm deformirovaniya materialov s uchetom fakticheskogo izmeneniya ploshchadi ikh poperechnykh sechenii. Diss. kand. tekhn. nauk [Development of methods for calculating reinforced concrete elements based on actual diagrams of the deformation of materials, taking into account the actual change in the area of their cross-sections. Dr. eng. sci. diss.]. Voronezh, 2006. 230 p.
5.Klochkov A. G., Chistyakov E. A. K raschetu nesushchei sposobnosti gibkikh vnetsentrenno-szhatykh zhelezobetonnykh elementov kol'tsevogo secheniya [To calculate the load-bearing capacity of flexible non-centrally compressedreinforcedconcreteelementsofannularcross-section].Vestnik LPI,1966,vol.3,no.2,pp. 15––26.
6.Kryukov A. A., Zhdanov A. E. Podkhody k otsenke deformativnosti izgibaemykh zhelezobetonnykh elementov na osnove iteratsionnykh metodov rascheta [Approaches to estimating the deformability of bent reinforced concrete elements based on iterative calculation methods]. Vestnik BGTU im. V. G. Shukhova, 2017, no. 1, pp. 73––76.
7.Mailyan L. R., Stel'makh S. A., Kholodnyak M. G., Shcherban'E. M., Khalyushev A. K. Vliyanie tekhnologii proizvodstva na strukturoobrazovanie i svoistva betona vibrotsentrifugirovannykh kolonn [Influence of production technology on the structure formation and properties of concrete of vibro centrifuged columns]. Stroitel'stvo i arkhitektura, 2017, vol. 5, iss. 4 (17), pp. 224––228.
8.Nazhuev M. P., Yanovskaya A. V., Kholodnyak M. G., Stel'makh S. A., Shcherban'E. M., Osadchenko S. A. Analiz zarubezhnogo opyta razvitiya tekhnologii vibrotsentrifugirovannykh stroitel'nykh konstruktsii i izdelii iz betona [Analysis of foreign experience in the development of technology of vibro centrifuged building structures and concrete products]. Vestnik Evraziiskoi nauki, 2018, no. 3.
9.Obernikhin D. V., Nikulin A. I. Eksperimental'nye issledovaniya deformativnosti izgibaemykh zhelezobetonnykh elementov razlichnykh poperechnykh sechenii [Experimental studies of the deformability of bent reinforced concrete elements of various cross-sections]. Vestnik BGTU im. V. G. Shukhova, 2017, no. 4, pp. 56––59.
10.Ryazanov M. A. Raschet izgibaemykh elementov s uchetom fizicheskoi nelineinosti deformirovaniya [Calculation of bending elements taking into account the physical nonlinearity of deformation]. Vestnik BGTU im. V. G. Shukhova, 2016, no. 12, pp. 58––64.
11.Stel'makh S. A., Kholodnyak M. G., Shcherban'E. M., Nasevich A. S., Yanovskaya A. V. Ustroistvo dlya izgotovleniya izdelii iz vibrotsentrifugirovannogo betona [Device for manufacturing products from vibrocentrifuged concrete]. Zayavka na poleznuyu model' Rossiya, MPK V28V 21/30. No. 2020103753/09; zayavl. 29.01.2020.
12.Suleimanova L. A. Vysokokachestvennye energosberegayushchie i konkurentosposobnye stroitel'nye materialy, izdeliya i konstruktsii [High-quality energy-saving and competitive building materials, products and structures]. Vestnik BGTU im. V. G. Shukhova, 2017, no. 1, pp. 9––16.
43

Russian Journal of Building Construction and Architecture
13.Kholodnyak M. G., Stel'makh S. A., Shcherban'E. M., Nazhuev M. P., Yanovskaya A. V., Osadchenko S. A. Mekhanicheskie svoistva vibrotsentrifugirovannykh betonov s kombinirovannym zapolnitelem i voloknistoi dobavkoi [Mechanical properties of vibro-centrifuged concretes with combined aggregate and fiber additive].
Inzhenernyi vestnik Dona, 2018, no. 3.
14.Kholodnyak M. G., Stel'makh S. A., Shcherban'E. M., Tret'yakov D. A., Dao V. N., Zaikin V. I. Predlozheniya po raschetnomu opredeleniyu prochnostnykh kharakteristik vibrirovannykh, tsentrifugirovannykh i vibrotsentrifugirovannykh betonov [Proposals for the calculated determination of the strength characteristics of vibrated, centrifuged and vibro-centrifuged concrete]. Vestnik Evraziiskoi nauki, 2018, no. 6.
15.Darko Tasevski, Ruiz Miguel Fernández, Muttoni Aurelio. Compressive strength and deformation capacity of concrete under sustained loading and low stress rates. Journal of Advanced Concrete Technology, 2018, vol. 16, pp. 396––415.
16.Ferrotto M. F., Fischer O., Cavaleri L. Analysis-oriented stress-strain model of CRFP-confined circular concrete columns with applied preload. Mater Struct, 2018, vol. 51, no. 44.
17.Ippei Maruyama, Lura Pietro. Properties of early-age concrete relevant to cracking in massive concrete. Cement and Concrete Research, 2019, vol. 123, P. 105770.
18.Mailyan L. R., Stel’makh S. A., Shcherban’E. M., Kholodnyak M. G. Determination and use of hidden strength reserves of centrifuged reinforced constructions by means of calculation and experimental methods.
Russian Journal of Building Construction and Architecture, 2020, no. 1 (45), pp. 6––14.
19.Shuyskiy A. I., Stel’makh S. A., Shcherban’E. M., Kholodnyak M. G. Investigation of the Influence of the Initial Composition of Heavy Concrete Designed for the Manufacture of Ring-Section Products on its Properties. Materials Science Forum, 2018, vol. 931, pp. 508––514.
20.Stel'makh S. A., Shcherban'E. M., Shuyskiy A. I., Nazhuev M. P. Theoretical and Practical Aspects of the Formation of the Variational Structure of Centrifuged Products from Heavy Concrete. Materials Science Forum, 2018, no. 931, pp. 502––507.
21.Stel'makh S. A., Shcherban E. M., Zholobova O. A. Prescription and Technological Aspects of Manufacturing High-Quality Centrifuged Products and Structures from Heavy Concrete. IOP Conference Series: Materials Science and Engineering. International Multi-Conference on Industrial Engineering and Modern technologies, 2018, vol. 463, p. 022056.
44
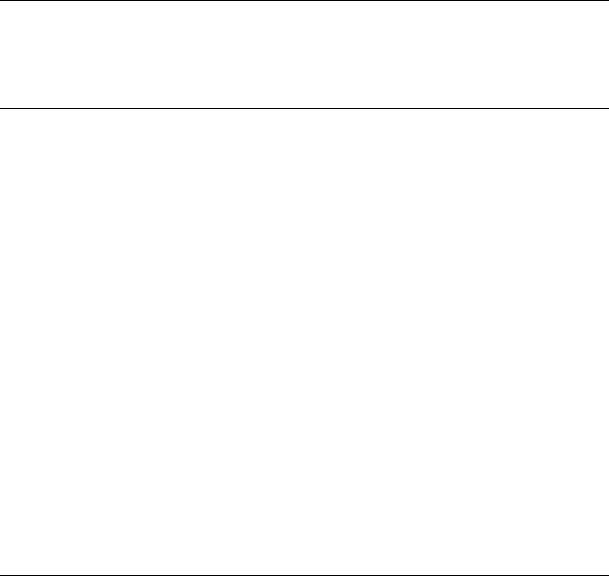
Issue № 1 (49), 2021 |
ISSN 2542-0526 |
HEAT AND GAS SUPPLY,VENTILATION,AIR CONDITIONING,
GAS SUPPLY AND ILLUMINATION
DOI 10.36622/VSTU.2021.49.1.004
UDC 536.24
L. A. Kushchev1, V. N. Melkumov2, N. Yu. Savvin 3
COMPUTER SIMULATION OF FLOW IN CORRUGATED CHANNEL
OF PLATE HEAT EXCHANGER
Belgorod State Technological University Named after V. G. Shukhov, Institute of civil engineering 1, 3 Russia, Belgorod
Voronezh State Technical University 2
Russia, Voronezh
1 D. Sc. in Engineering, Prof. of the Dept. of Heating and Ventilation, tel.: +7-910-363-62-09, e-mail: leonidkuskev@gmail.com
2 D. Sc. in Engineering, Prof. of the Dept. of Heat and Gas Supply and Oil and Gas Business, tel.: (473) 271-53-21, e-mail: teplosnab_kaf@vgasu.vrn.ru
3 PhD student of the Dept. of Heating and Ventilation, tel.: +7-952-422-25-75, e-mail: n-savvin@mail.ru
Statement of the problem. The heat exchange process occurring in a modified corrugated interplate channel of an intensified plate heat exchanger with an increased turbulence of the heat carrier is discussed. A computer model of the coolant movement in the speed range of 0.1––1.5 m/s is developed and the turbulence coefficient of the plate heat exchanger is determined.
Results. The article presents the results of computer modeling of the coolant movement in the interplate corrugated channel of the original plate heat exchanger using the Ansys software package. The criteria of system stability are defined. 3D modeling of the channel formed by corrugated plates is performed. In the study of the process of turbulence several high-speed modes of movement of the coolant were considered. The turbulence coefficient Tu, % is determined.
Conclusions. As a result of computer simulation, an increase in the heat transfer coefficient K, W/(m2 ) was found due to an increased turbulization of the flow, which leads to a decrease in metal consumption and a decrease in the cost of heat exchange equipment.
Keywords: computer simulation, heat transfer coefficient, plate heat exchanger, corrugated surface and the coefficient of turbulence.
Introduction. The development of computer technology and its takeover of all spheres of human life and work has become an essential part of technological progress. The ANSYS engineering analysis software is commonly used for performing thermal engineering and hydrodynamic investigations. The complex enables one not only to conduct qualitative modeling of systems of various physical nature, but also to examine the response of these systems to external
© Kushchev L. A., Melkumov V. N., Savvin N. Yu., 2021
45

Russian Journal of Building Construction and Architecture
influences in the form of distribution of temperatures, stresses, velocities, etc. The use of such programs helps design organizations to reduce the development cycle as well as the cost of products and improve product quality [4].
Currently, plate heat exchangers are commonly employed in the chemical, food industries, heat power engineering and a range of other industries. Their introduction has become cutting-edge in the energy sector of housing and communal services. This is due to the high technical and economic performance of the plate heat exchange equipment. Hence the problem of modernizing and improving the technical characteristics of such equipment is extremely relevant.
The objective of the study is to develop a computer model of the movement of a coolant with increased turbulization based on a 3D model of a corrugated channel of an intensified plate heat exchanger for housing and communal services.
1. Computer simulation of the movement of the coolant. Based on the “Practical Guide to the Ansys software package [6] for modeling heat exchange processes in an intensified plate heat exchanger, we set forth the following system stability criteria:
––the isothermal process of heat exchange with the environment is considered; Petrolimex 60/70 bitumen in accordance with the requirements of TCVN 7493-2005;
––the liquid is incompressible;
––density of the liquid ρ, kg/m3 = const (along the length of the surface);
––fluid movement occurs under the influence of gravity (g = 9.81 m/s2);
––W1 = 0.1––1.5 m/s where W1 is the fluid velocity at the inlet to the heat exchanger;
––liquid temperature 70 ° C at an ambient temperature of –23 ° C (according to the temperature schedule of heat energy supply for Belgorod region);
––p = 0.9 MPa, p is the pressure at the beginning of the considered section of the heat exchange surface;
––convergence of the solution of the model equations is 10-3.
All the methods of intensifying the heat exchange process are designed to increase the heat transfer coefficient K, W/(m2 0С) [5, 11] which for plate heat exchangers is given by the formula:
К = |
|
|
|
|
|
|
, |
|
|
|
|
|
|
|
|
||
|
1 |
|
1 |
|
СТ |
(1) |
||
|
1 |
2 |
|
СТ |
|
|
where β is the coefficient considering the decrease in the heat transfer coefficient due to the thermal resistance of scale and impurities on the plate depending on the quality of the water is
46

Issue № 1 (49), 2021 |
ISSN 2542-0526 |
taken equal to 0.7––0.85; α1 is the coefficient of heat transfer from the heating water to the plate wall, W/(m2 0С); α2 is the coefficient of heat absorption from the wall of the plate to the heated water, W/(m2 0С); δСТ is the thickness of the heat exchange plate, m; λСТ is the coefficient of thermal conductivity of the plate material, W/(m2 0С).
The direction of the flow of heat carriers in the heating and heated circuits is taken in compliance with SP 41-101-95 “Designing Heat Points” counter-current as the most effective for operation in heat supply systems of housing and communal services [10, 14]. The flow diagram of the coolants in the heat exchanger is shown in Fig.1.
Fig. 1. Scheme of movement of heat carriers in intensified lamellar
heat exchanger:
t1gr is the initial temperature of the heating circuit coolant; t2gr is the final temperature of the heating circuit coolant; t1ng is the initial temperature of the coolant of the heated circuit; t2ng is the final temperature of the heating agent
of the heated circuit
In the process of studying the values of the velocities of the coolant movement in the interplate channel of the intensified plate heat exchanger, the following were taken:
––0.4 m/s as the optimal speed while calculating plate water heaters (this value was established during the review of regulatory documents for the design of heat exchange equipment [8]);
––1 m/s is taken as the maximum for the standard size of the investigated heat exchangerin compliance with SP 41-101-95 “Designing of Heat Points”;
––1.5 m/s is the maximum possible speed according to Ridan studies.
The liquid pressure at the beginning of the surface was taken as 0.9 MPa, since, according to the normative documentation for the equipment in operation in the internal heating and hot water supply systems of housing and communal services, this value does not exceed 1 MPa.
2. Model of the modified corrugated channel. We have suggested an original design of an intensified lamellar apparatus whose feature are corrugated plates with spherical depressions, located according to a linear law on the areas between the corrugations [12].
The radius of the spherical indentations r0 in order to maintain the rigidity of the plate must have a value (0.1 ÷ 0.7) of the plate thickness. The recommended value is 0.35 mm. The dis-
47

Russian Journal of Building Construction and Architecture
tance between the spherical recesses located in the channels formed by the corrugations, according to the fundamental research of Zukauskas [5], (6 ÷ 12) r0 is recommended. This distance ensures the formation of a continuous turbulent wake.
It is recommended that structural and operating methods are employed together, in a complex manner in order to obtain optimal conditions for the heat exchange process. This conclusion is confirmed by theoretical and practical research by A. A. Gukhman [3], since the major process of heat transfer occurs in the boundary layer [1, 19], and hence the development of effective methods for influencing the wall region promotes turbulization of the coolant flow [9, 7, 16].
The main stage in modeling heat exchange processes in the Ansys software package is the creation of a 3D model shown in Fig. 2.
Fig. 2. 3D-model of modified corrugated plates
This 3D model of the modified corrugated plates makes it possible to visually identify the turbulence areas located in the temperature boundary layer.
According to the scientific research by X. Kexin, S. Robin, P. Kumar, employees of an engineering center located in New Delhi, a high level of heat transfer in the boundary layer is facilitated by an enhanced renewal of vortex formations, i.e. rapid mixing of some volumes with different temperatures and speeds with others. An increase in the difference between the temperature distribution and the speed of movement of the coolant near the heat-transferring wall with a modified geometry contributes to that in the intensity of heat transfer [15, 17, 18,
48

Issue № 1 (49), 2021 |
ISSN 2542-0526 |
20, 21]. In order to identify the turbulization coefficient Tu, %, the major range of variation of the coolant velocity (from 0.1 m/s to 1.5 m/s) in the modified interplate channel was examined. Limiting mode, 1.5 m/s is not employed in housing and communal services due to the increased flow rate of the coolant, but it presents theoretical interest. The outcomes of the study are presented in the form of the temperature distribution of the coolant in the interplate channel formed by two modified corrugated plates (Fig. 3).
а)
b)
c)
Fig. 3. Graphical distributions of the degree of flow turbulization at different flow rates around the modified heat transfer surface: coolant speed –– 0.4 m/s (a), 1 m/s (b), 1.5 m/s (c)
49

Russian Journal of Building Construction and Architecture
When analyzing the results of the graphical distribution of the degree of flow turbulization at different speeds of the coolant, vortex formation zones were identified that contribute to an increase in the efficiency of heat transfer and the heat transfer coefficient K, W / (m2 0С). Ultimately, a high value of K will lead to a decrease in metal consumption and a decrease in economic costs.
As a result of computer simulation of the movement of the coolant, the following parameters of the fluid flow were identified:
1)the degree of flow turbulization in the entire section of the turbulization zone;
2)the length of the turbulization zone (the turbulization zone ends when the turbulence of the flow of the vortex zone and the major fluid flow is equal);
3)the height of the turbulization zone.
The average value of the flow turbulence coefficient for calculating the heat transfer coefficient identified using the Ansys program is shown in the table.
|
|
|
|
|
|
|
|
|
Table |
|
Average turbulence coefficient |
|
|
|
|
|
|||
at different speeds of the coolant in the interplate channel |
|
|
|
||||||
|
|
|
|
|
|
|
|
|
|
Coolant speed, m/s |
|
0.1 |
0.25 |
|
0.5 |
0.75 |
1 |
1.25 |
1.5 |
|
|
|
|
|
|
|
|
|
|
Turbulence coefficient value |
|
2.4 |
4.3 |
|
6.9 |
11.1 |
14 |
19.7 |
24.6 |
for modified corrugated plate Tu,% |
|
|
|||||||
|
|
|
|
|
|
|
|
|
|
|
|
|
|
|
|
|
|
|
|
Using the least squares method [2], we obtain linear dependences of the turbulization coefficient on the average fluid velocity of the interplate channel for the modified corrugated plate Tu, %.
Tu =13.4 W, |
(2) |
where W is the coolant velocity in the interplate channel, m/s.
Hence, as a result of computer simulation of the movement of the coolant in the corrugated interplate channel, the turbulization coefficient Tu, %, necessary to improve the method of calculating the heat transfer coefficient K, W/(m2 0С), which is the major characteristic of the efficiency of the heat exchange equipment, was identified. It has been established that the use of original plates with spherical recesses causes an increase in the heat transfer coefficient K, W/(m2 0С) [16]. The results of experimental studies are shown in Fig. 4.
As the graph indicates, the heat transfer coefficient of an intensified plate heat exchanger increases linearly as does the coefficient of a serial plate heat exchanger, but the value K for the
50